 |
|
The Antarctic Rift: Plume vs. Plate Dynamics
|
|
Sergio
Rocchi
Dipartimento
di Scienze della Terra, Università di Pisa,
Italy
rocchi@dst.unipi.it
Co-workers:
P. Armienti (Univ. Pisa), G. Di Vincenzo (IGG CNR
Pisa), M. D’Orazio (Univ. Pisa), I. Nardini
(Univ. Pisa), F. Rossetti (Univ. Roma), F.Storti (Univ.
Roma), S. Tonarini (IGG CNR Pisa)
Click here to
download a PDF version of this webpage
|
Introduction
Thinning of the lithosphere in major continental rift
systems is commonly considered in terms of the end-members models of
active versus passive rifting, and a genetic connection is commonly
acknowledged between lithospheric extension, magmatism and mantle plume
activity.
The West Antarctic Rift System (WARS) (Figure 1) in
one of the major active continental rifts on Earth, with late Oligocene
to Recent volcanic activity [1]. Geophysical investigations and studies
of volcanism led to the proposal of a genetic link between the WARS
and an active plume centered below Marie Byrd Land [2]. The evidence
cited in favour of this hypothesis includes:
-
geochemical similarity between the basalts from the
WARS and basalts associated with long-lived hot-spot tracks [3];
-
the presence in Marie Byrd Land of horst-graben
sub-ice topography producing a large uplifted dome [4];
-
modest Cenozoic extension in the WARS, insufficient
to generate the observed amount of magmatism;
-
the lack of significant plate tectonic events coeval
with rifting and volcanism in West Antarctica [3]; and
-
high heat flow in the Ross Sea area [5].
This model has been progressively extended to the whole
rift system, mainly based on the geochemical features of magmas, leading
to the hypothesis of rifting linked to two plumes, active below Marie
Byrd Land and Mt. Erebus, respectively [5].
Recent geological-geophysical investigations in the
Ross Sea region (namely Victoria Land and the Ross Sea) highlighted
complex Cenozoic geodynamics dominated by intraplate, right-lateral
strike-slip tectonics inducing a significant oblique component in the
rifting process [6]. This, and the spatial, structural, and chronological
distribution of plutons and dyke swarms recently found on the western
Ross Sea shoulder (e.g., [7-10]), casts doubts on the plume
scenario and may support a transtension-related source for the Cenozoic
magmatism of the Ross Sea region.
In this webpage, the geochemical, chronological and
structural evidence is critically compared with the main features expected
for a plume-powered system, and a model is proposed that is an alternative
to both plume-driven and purely passive rifting.
|
Tectonics, magmatism and geochemistry
- No time progression of volcanic activity exists in the WARS.
- This could be related to the peculiar setting of the Antarctic
plate, which has been stationary since the late Cretaceous, and
almost completely encircled by mid-ocean ridges [3].
»
NO definitive proof either in favour or against plume occurrence in
the WARS.
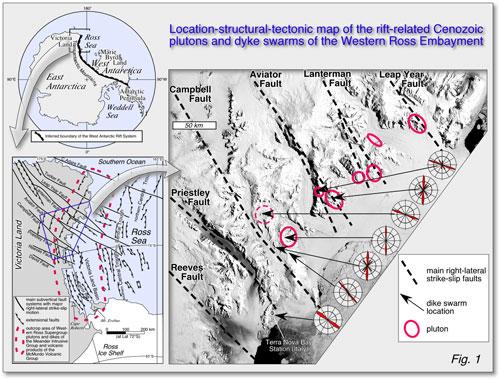
Figure 1: Location map. Click on map to enlarge.
2. Doming? Trend and
and shape of surface uplift
- Overall domal uplift in Marie Bird Land.
- No doming on the western coast of the Ross Sea. Cenozoic uplift
is linear, leading to the formation of the Transantarctic Mountains,
dissected by transverse fault systems in northern Victoria Land.
»
Lack of the circular symmetry typically associated with mantle plumes.
3. Uplift-extension:
relative timing
- Prolonged subsidence and basin formation in the Ross Sea during
the Cretaceous and Cenozoic.
- The main extensional episode occurred in the late Cretaceous [11],
while the main denudation-uplift event began in the middle Eocene.
»
The activity of a plume with higher-than-normal mantle potential temperature
is difficult to reconcile with (1) Cretaceous and Cenozoic subsidence,
and (2) uplift following extension rather than preceding it.
4. Extension-magmatism:
relative timing
- The main extensional episode occurred in the late Cretaceous.
- Magmatism started in the middle Eocene.
»
The magmatism started 30 Myr later than the main extension.
5. Cenozoic
lithospheric extension
- The amount of Cenozoic extension is still matter of debate [12,
13].
»
The model proposed here does not require Cenozoic extension.
6. Volume
of magmatism
- Aeromagnetic data have been interpreted as evidence for a large
volume of volcanic products (106 km3) concealed
beneath the ice sheet [14].
- This volume, when the long duration of igneous activity is taken
into account (almost 50 Myr), gives low average magma production rates.
»
The magma production rate is much lower than that expected in a mantle
plume-dominated scenario [15]. |
7.
Magma chemistry
- Trace elements and Sr-Nd-Pb isotopes indicate OIB-HIMU chemistry
(Figure 2).
- Primitive lavas have low He isotope ratios (R/Ra=6-7) [16]. In
the standard helium-isotope model, these values are lower than those
expected for magmas from mantle plumes rising from the lower mantle.
Recent criticism on He systematics [17] has questioned the primary
validity of the standard model for He, and this should be borne in
mind when considering these data (see also Helium
fundamentals webpage).
»
Focal point: a mantle plume is a physical entity, not a geochemical reservoir.
Therefore plume occurrence (including both active and fossil) cannot be
inferred from geochemistry alone.
- A residual potassic (hydrous) phase in the source [8] implies a
magma source temperature lower than invoked for mantle plumes [18].
Mantle domains rich in hydrous phases are sufficient to explain “hot-spot-like”
basalt geochemistry without the need for anomalously high mantle temperatures
[19].
- The geochemical features of magmatism did not vary over 50 Myr
[8].
- The geochemical features of magmatism show little variability across
the whole rift and beyond (i.e. across the whole Antarctic
plate) [8].
- It is worth noting that HIMU igneous provinces straddling the continent-ocean
boundary (e.g., the Cameroon Line) share the geochemical
features of continental rifts and oceanic islands.
»
Low source temperature, the same source all across the Antarctic plate
and beyond, and the same source for 50 Myr: is there a fossil plume head
below the WARS?
- The geochemically-based claim for a fossil plume head source, is
invoked to satisfy the need for a shallow, weak, enriched layer (the
perisphere? [20]), common to large areas beneath the Southern Ocean
and the adjoining continents. One of the most commonly cited isotopic
arguments in support of mantle plumes is the high 206Pb/204Pb
ratio, proposed to result from deep mantle plumes that entrained slab
material subducted into the deep mantle over a long time period (109
years). However, such a high 206Pb/204Pb ratio
can also be attained in the magma source at shallow depth, in a shorter
period of time (108 years, provided the source has a rather
high U/Pb ratio [21]). The Cenozoic mafic dykes and lavas from northern
Victoria Land have average U/Pb ratio of 0.44±11 and 0.66±0.17.
This implies a high U/Pb ratio in the magma source, which therefore
has been able to produce high 206Pb/204Pb ratios
over a time span of the order of 108 years.
» In the model
proposed here, source enrichment occurred in the late Cretaceous, some
tens of million years before the commencement of magmatism. It is not
related to mantle plume activity, but to a geologic process for which
there is evidence – lithospheric extension.
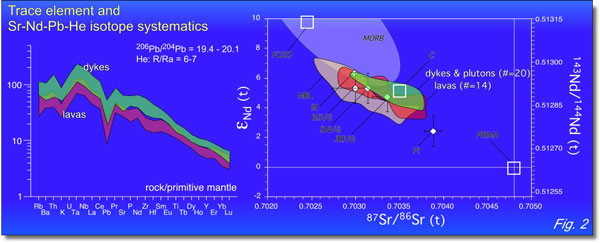
Figure 2: Trace element and
Sr-Nd-Pb-He isotope systematics. Abbreviations: MBL: Neogene lavas from
Marie Byrd Land; PI: Peter Island; BI: Balleny Islands; JRIVG, SNVG, BSVG:
volcanic groups of the Antarctic Peninsula. Details on data sources are
given in [8]. Click on figure to enlarge. |
Relative timing of
events
8. Space-time distribution
of igneous activity
- In addition to the lack of hot spot tracks, no radial patterns
of igneous activity are found.
- Rather, magmatism was activated sequentially in adjacent crustal
sectors.
- The dyke swarms have NNW or NS strikes, pointing to a geometric
link with intraplate NNW strike-slip fault systems [8].
- NNW and NS dykes are coeval [8].
- Pseudotachylyte generation is coeval with dyke emplacement [22].
»
Magma emplacement and regional tectonic activity share the same geometry
and timing.
Geometry
and timing of igneous activity vs. plate dynamics
- Tight geometric link between dextral strike-slip systems in northern
Victoria Land and Southern Ocean Fracture Zones ([6], Figure 3)
- Beginning of magmatism is coeval with global plate reorganization
[23, 24] and increase in differential movements of Southern Ocean
Fracture Zones (43-47 Ma) [25, 26].
»
The tectonic framework to which emplacement of magmas is linked, is
related to plate-scale tectonic processes. Also the beginning of magmatism
coincides with a process that could trigger differential movements along
the strike-slip fault systems, i.e differential movements on oceanic
fracture zones, as shown by the Cenozoic age of pseudotachylytes generated
on a NNW fault system in Victoria Land, which is a Paleozoic fault reactivated
by the oceanic fracture zones [6, 8, 22]. Hence, the igneous activity
appears to be governed by plate dynamics, i.e. from above, and not from
below [27].
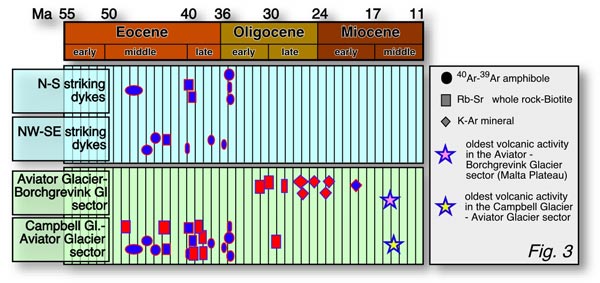
Figure 3: The chronology of Cenozoic magmatism in northern Victoria
Land.
|
Temperature
Hot mantle? Mantle tomography
- The depth to which seismic tomography can reliably image in the
Antarctic region is 200 km, which is not deep enough to confirm or
disprove the presence of an active deep mantle plume.
- Slower (hotter?) mantle does not show circular symmetry, but is
rather linear. In the continental part of the Antarctic Plate it is
clearly bounded by the cold East Antarctic Craton, and in the oceanic
part of both the Antarctic and Australian plates it overlaps with
the belt of ridge transformation (i.e., the zone around 150°E
where the closely spaced oceanic fracture zones displace the Australia-Antarctica
ridge by ~ 1,300 km, from 50°S to ~ 62°S) (Figure 4) [28].
» Shallow,
hot mantle is related to a linear geodynamic feature > 4,000 km long,
that extends from Tasmania to the Ross Sea. |
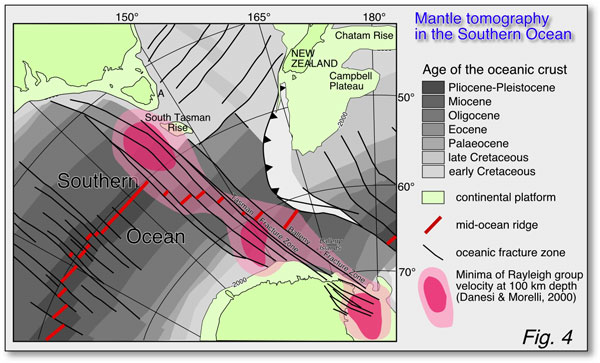
Figure 4: Plate tectonic setting and mantle tomography in the Southern
Ocean.
A general model for
the tectonomagmatic history of the western Ross Embayment
- Late Cretaceous: an early rift phase occurred with orthogonal
extension that stretched the crust and the underlying strong lithospheric
mantle (Figure 4, [10]). Lithospheric thinning probably led to the
production of very small degree partial melts. These were not sufficient
to give way to surface magmatism (i.e. rifting was amagmatic),
but distributed fertile, enriched, low-melting point veins/domains
widely throughout the Antarctic plate mantle.
- Middle Eocene: the increase of differential velocity along
the Southern Ocean Fracture Zones reactivated the Paleozoic tectonic
discontinuities in northern Victoria Land as intraplate dextral strike-slip
fault systems. The activity of these lithospheric deformation belts
promoted local decompression melting of the enriched mantle domains
created during the late Cretaceous and isotopically matured since
then (Figure 4). The magma rose and was emplaced along the main NW-SE
discontinuities and along the N-S transtensional faults arrays departing
from the master NW-SE systems (Figure 1).
- This model (Figure 5) relates the driving forces of events such
as uplift, active faulting, magmatism and seismicity to the dynamics
of the Antarctic plate rather than to deep-source forces such as mantle
plumes. It is therefore potentially testable, e.g., by:
- checking the distribution of magmatism vs. strike-slip fault systems
in the still poorly known areas onshore, such as central Victoria
Land (where fault systems are almost lacking) and northernmost Victoria
Land (where the faults are widely spaced: Figure 1);
- determining the age of pseudotachylytes in other areas, to control
further their contemporaneity with magma emplacement;
- using the growing seismic data set to determine mantle velocity
anomalies deeper than 200 km;
- using aeromagnetic data to define better the shapes of partly buried
intrusions and link them to a tectonic framework;
- investigating the origin of felsic magmas (differentiation vs. unmixing
[9]) to constrain the original volume of mafic melt and developing
models for the structure of the upper crust.
Figure 5: Model for Cretaceous and Cenozoic tectonic
development of the Antarctic rift. Click on figure to enlarge. |
-
LeMasurier W.E., Thomson J.W.,
eds. 1990. Volcanoes of the Antarctic Plate and
Southern Oceans. Antarctic Research Series.,
48. American Geophysical Union,
487.
-
Behrendt J.C., LeMasurier W.E.,
Cooper A.K., Tessensohn F., Tréhu A., Damaske
D., 1991. Geophysical studies of the West Antarctic
Rift System. Tectonics. 10(6):
1257-1273.
-
Hole M.J., LeMasurier W.E.,
1994. Tectonic controls on the geochemical composition
of Cenozoic, mafic alkaline volcanic rocks from
West Antarctica. Contributions to Mineralogy
and Petrology. 117: 187-202.
-
LeMasurier W.E., Landis C.A.,
1996. Mantle-plume activity recorded by low relief
erosion surfaces in West Antarctica and New Zealand.
Geological Society of America Bulletin.
108: 1450-1466.
-
Storey B., Leat P.T., Weaver
S.D., Pankhurst R.J., Bradshaw J.D., Kelley S.,
1999. Mantle plumes and Antarctica-New Zealand rifting:
evidence from mid-Cretaceous mafic dykes. Journal
of the Geological Society, London. 156:
659-671.
-
Salvini F., Brancolini G., Busetti
M., Storti F., Mazzarini F., Coren F., 1997. Cenozoic
geodynamics of the Ross Sea region, Antarctica:
Crustal extension, intraplate strike-slip faulting,
and tectonic inheritance. Journal of Geophysical
Research. 102(B11): 24,669-24,696.
-
-
-
Rocchi S., Fioretti A.M., Cavazzini
G., 2002. Petrography,
geochemistry and geochronology of the Cenozoic Cape
Crossfire, Cape King and No Ridge igneous complexes
(northern Victoria Land, Antarctica), in Antarctica
at the close of a millennium. Proceedings of
the 8th International Symposium on Antarctic Earth
Sciences, Wellington 1999, Royal Society of New
Zealand Bulletin, J.A. Gamble, D.N.B. Skinner, and
S. Henrys, Editors: Wellington, New Zealand. 215-225.
-
Rocchi S., Storti F., Di Vincenzo
G., Rossetti F., 2003. Intraplate
strike-slip tectonics as alternative to mantle plume
activity for the Cenozoic rift magmatism in the
Ross Sea region, Antarctica, in Intraplate
strike-slip deformation belts, F. Storti, R.E.
Holdsworth, and S. F., Editors. Geological Society
Special Publication. 158-171.
-
Fitzgerald P.G., Stump E., 1997.
Cretaceous and Cenozoic episodic denudation of the
Transantarctic Mountains, Antarctica: new constraints
from apatite fission track thermochronology in the
Scott Glacier region. Journal of Geophysical
Research. 102 (B4): 7747-7765.
-
Cande S.C., Stock J.M., Müller
R.D., Ishihara T., 2000. Cenozoic motion between
East and West Antarctica. Nature. 404:
145-150.
-
Lawver L.A., Gahagan L.M., 1994.
Constraints on timing of extension in the Ross Sea
region. Terra Antartica. 1:
545-552.
-
Behrendt J.C., Saltus R., Damaske
D., McCafferty A., Finn C.A., Blankenship D., Bell
R.E., 1996. Patterns of late Cenozoic volcanic and
tectonic activity in the West Antarctic rift system
revealed by aeromagnetic surveys. Tectonics.
15(2): 660-676.
-
Finn C., Bell R.E., Blankenship
D.D., Behrendt J.C., 2001. The relation of crustal
structure, warm mantle, and ice sheets to Cenozoic
volcanism in West Antarctica. in Antarctic Neotectonics,
Siena, Italy, 11-15 July 2001.
-
Nardini I., Armienti P., Rocchi
S., Tonarini S., Harrison D., 2003. Cenozoic Volcanism
in the Western Ross Embayment: any evidence for
a mantle plume from isotope systematics? in 9th
International Symposium on Antarctic Earth Sciences,
Potsdam (Germany), 8-12 September 2003.
-
-
Smith A.D., Lewis C., 1999.
The planet beyond the plume hypothesis. Earth
Science Reviews. 48: 135-182.
-
Bonatti E., 1990. Not so hot
“hot spots” in the oceanic mantle. Science.
250: 107-111.
-
-
Halliday A.N., Lee D.-C., Tommasini
S., Davies G.R., Paslick C.R., Fitton J.D., James
D.E., 1995. Incompatible trace elements in OIB and
MORB and source enrichment in the sub-oceanic mantle.
Earth and Planetary Science Letters. 133:
379-395.
-
Rossetti F., Di Vincenzo G.,
Läufer A., Lisker F., Rocchi S., Storti F.,
2003. Cenozoic right-lateral strike-slip faulting
in North Victoria Land: and integrated structural,
AFT and 40Ar-39Ar study. in
9th International Symposium on Antarctic Earth
Sciences - Antarctic Contribution to Global Earth
Science. Potsdam (Germany).
-
Lithgow-Bertelloni C., Richards
M.A., 1998. The dynamics of Cenozoic and Mesozoic
plate motions. Reviews of Geophysics. 36:
27-78.
-
Veevers J.J., 2000. Change of
tectono-stratigraphic regime in the Australian plate
during the 99 Ma (mid-Cretaceous) and 43 Ma (mid-Eocene)
swerves of the Pacific. Geology. 28:
47-50.
-
Cande S.C., Mutter J.C., 1982.
A revised identification of the oldest sea-floor
spreading anomalies between Autralia and Antarctica.
Earth and Planetary Science Letters. 58:
151-160.
-
Richards M.A., Lithgow-Bertelloni
C., 1996. Plate motion changes, the Hawaii-Emperor
bend, and the apparent success and failure of geodynamic
models. Earth and Planetary Science Letters.
137: 19-27.
-
-
Danesi S., Morelli A., 2000.
Group velocity of Rayleigh waves in the Antarctic
region. Physics of the Earth and Planetary Interiors.
122: 55-66.
last updated 7th October,
2006 |
|
|