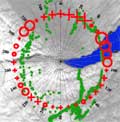 |
Mantle
transition zone discontinuities beneath
the Baikal rift and adjacent areas
Kelly
H. Liu & Stephen
S. Gao
Department of Geological Sciences and Engineering,
University of Missouri-Rolla
liukh@umr.edu |
|
|
Click here to
download a PDF version of this webpage
Click
here to go to Discussion of this page
Introduction
The Baikal rift zone (BRZ)
in Siberia is a major continental rift zone. This 1500-km-long
en echelon system of rift depression, which
originated about 35 Ma ago along the boundary between
the Archean Siberian platform and the Paleozoic-Mesozoic
Altai-Sayan-Baikal foldbelt, is the most seismically
active continental rift in the world [Logatchev
& Zorin, 1992; Keller et al., 1995]
(Figure 1). Similar to most other major continental
rifts, the BRZ is characterized by higher than normal
heat flow [Lysak, 1984], negative gravity anomalies
[Zorin et al., 1989], and thinned crust [Zorin
et al. 2002; Gao
et al.,
2004]. Rift-orthogonal mantle flow associated with
the rifting and rift-parallel magma-filled cracks beneath
the BRZ are suggested by measured shear-wave splitting
parameters [Gao et al., 1994a;
1997]
and inversion of P-wave arrival times [Gao
et al.,
2003]. [Ed: for more on the Baikal rift, see also
Diffuse,
long-lived Cenozoic volcanism in Mongolia, Topography,
geoid and gravity anomalies in Western Mongolia
and One
rift, two models.]
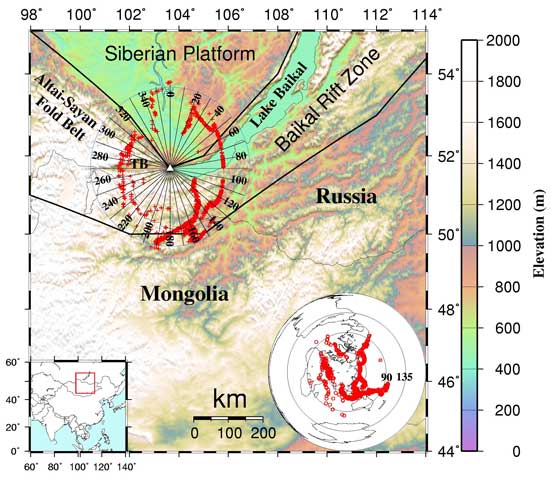
Figure 1. Topographic
map of the study area showing tectonic provinces, distribution
of ray-piercing points at 540 km depth (crosses), and
azimuthal bins. The bins have a width of 20° and
overlap by 10°. Consequently, the central azimuth
of the nth bin is 10n degrees from North. Station TLY
is represented by the open triangle, and TB is the Tunkin
Basin. Tectonically the BRZ is a domal structure in
the Altai-Sayan-Baikal foldbelt. In this study we refer
to the part of the foldbelt west of bin 15 as the Altai-Sayan
foldbelt, and to the eastern part with rifted valleys
as the BRZ. The insets show the location of the study
area, and the epicenters of the earthquakes used in
the study.
While most other continental
rifts such as the Rio Grande and East African rifts
are found to be underlain by a low velocity zone in
the upper mantle and even the mantle transition zone
(MTZ, 410-660 km depth range) [e.g., Davis,
1991; Slack et al., 1996; Achauer &
Masson, 2002; Wilson et al., 2005a], contradictory
seismic tomography results were obtained for the BRZ.
Most studies suggest low seismic velocities in the upper
mantle beneath the rift [e.g., Gao et al.,
1994b;
2003;
Achauer & Masson, 2002; Friederich,
2003; Brazier & Nyblade, 2003; Tiberi
et al., 2003; Zhao
et al.,
2006], but others did not find such anomalies [e.g.,
Petit
et al.,
1998]. The depth extent of the low-velocity anomaly
differs greatly among the studies, from less than 100
km to as deep as 600 km. Some studies suggested a plume-like
low-velocity cylindrical anomaly extending from about
135 km to the MTZ [Friederich, 2003], while
others indicated a depth extent of about 700 km [Petit
et al., 1998]. [Ed: see also Topography,
geoid and gravity anomalies in Western Mongolia
by Petit et al. 2005.]
The present study is aimed
at measuring the spatial variation of the depth to the
410 km discontinuity (d410) and the 660 km
discontinuity (d660) in the vicinity of the
BRZ and the Siberian platform. The objective is to provide
additional constraints on the deep structure, temperature,
and dynamics of the rift.
Data
and Method
The broadband seismograms
used in the study were recorded by GSN station TLY (Talaya,
N51.6807°, E103.6438°, elevation 579 m). Seismograms
from teleseismic events (epicentral distances 30°
to ~100°) that occurred between May 1991 and September
2005 were converted to radial receiver functions (RFs)
using the procedure of Ammon et al. [1990],
i.e., deconvolving the radial component with the vertical
component. A total of 1718 high-quality RFs were used
in the study (Figure 2). We use the RF-stacking approach
of Dueker & Sheehan [1998] to enhance the
weak P-to-S converted phases originating
from the top and bottom discontinuities of the MTZ (Figure
3). The bootstrap resampling procedure [Press et
al., 1992; Efron & Tibshirani, 1986]
was used to estimate the standard deviations (STDs)
of the discontinuity depths.
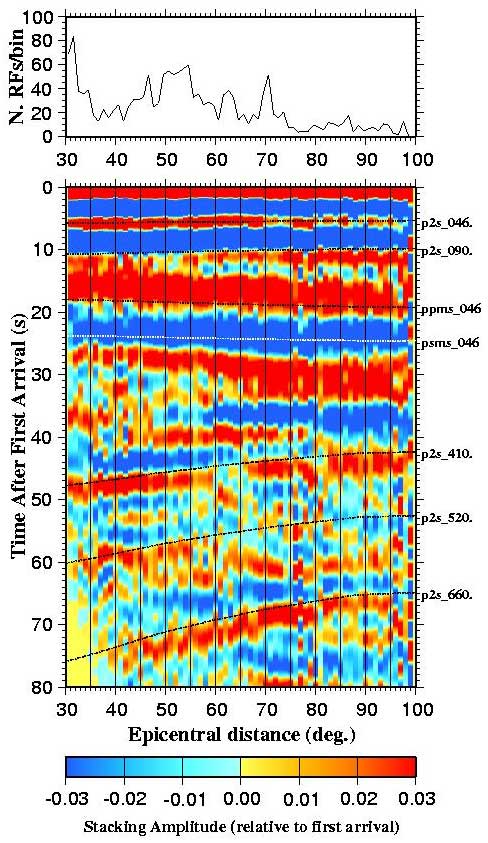
Figure 2. The bottom
plot shows stacked radial receiver functions. The 1718
radial receiver functions used in the study are arranged
according to their depth-corrected epicentral distances
into 1° ranges and those in the same range are then
stacked. The dashed lines labeled as P2S_xyz are predicted
moveout curves for P-to-S converted phases at depth
xyz km, and the lines labeled ppms_046 and psms_046
are the Moho (which has a depth of 46 km) reverberation
phases PPmS and PSmS, respectively. The top plot shows
the number of receiver functions per bin.
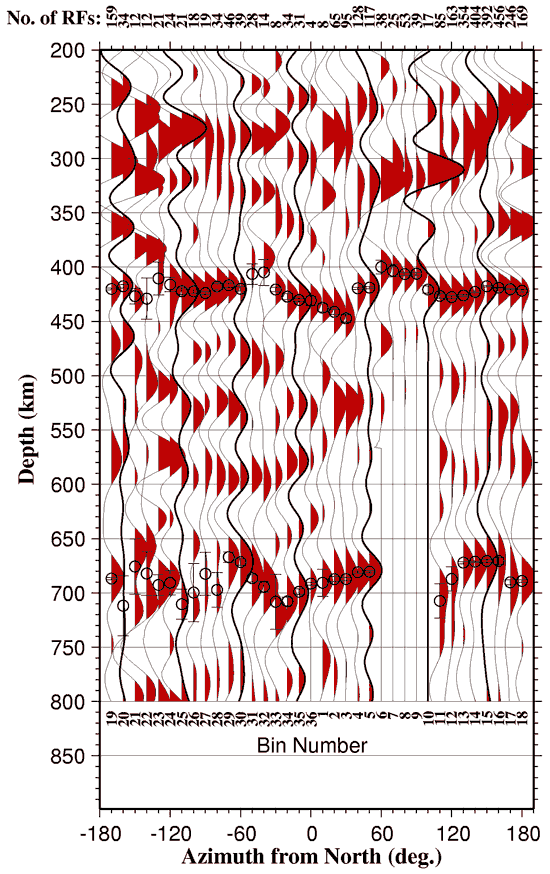
Figure 3. Results from
stacking radial receiver functions beneath the azimuthal
bins. Positive polarities of the traces are shaded.
The traces were normalized by the average of the maximum
amplitudes in the 400-450 and 660-720 depth ranges.
The top row shows the number of receiver functions per
bin at 540 km depth. Mean depths and their STDs from
the bootstrap steps are shown as circles and bars in
the 400-450 and 650-700 km depth ranges.
Results
and Discussion
1.
The 410 km Discontinuity (d410)
Figure 4A: The
depth to d410 varies from 400 ± 6
km to 447± 4 km. The mean depth is 421±
2 km, which is slightly deeper than the global
average of 418 km [Flanagan & Shearer,
1998]. Beneath the Altai-Sayan fold belt, d410
is relatively flat with a depth that is close
to the mean. A significant deepening of d410
toward the rift axis with a magnitude of
about 25 km is observed beneath the Siberian
platform, corresponding to an increase in
mantle temperature of about 300°C in
the vicinity of the d410
[Dueker & Sheehan, 1998; Gao
et al.,
2002; Shen
et al.,
2002; Liu
et al.,
2003]. An uplift of the d410
is found beneath the lake with a magnitude
of 47 km, corresponding to a temperature
reduction of about 550°C
beneath the rift relative to the south margin
of the platform.
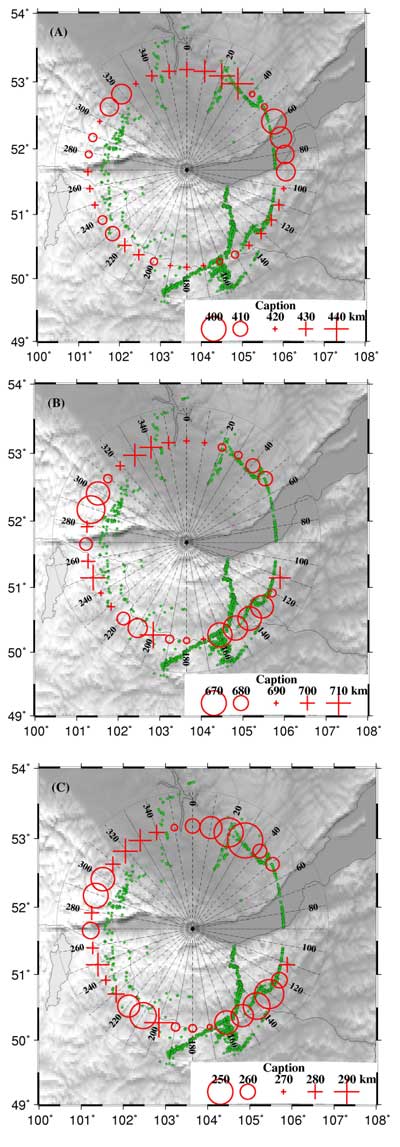
|
2.
The 660 km Discontinuity (d660)
Figure 4B: The mean
depth of d660 (688± 2 km) is significantly
greater than the global average of 660 km [Flanagan
& Shearer, 1998]. An uplift of d660
is observed beneath the foldbelt, centered near
the Tunkin Basin. The southern half of the uplift,
however, cannot be well-determined due to the
small number of RFs and resulting large STDs.
If the uplift is confirmed using additional data,
it suggests a thermal anomaly with a lateral temperature
contrast of several hundred degrees at the bottom
of the MTZ. A possible cause of the anomaly is
a mantle plume that has not reached d410.
Beneath the platform, d660 shallows gradually
toward the rift, from 708 km to 681 km, corresponding
to a temperature increase of about 460°C.
3.
MTZ Thickness
Figure 4C: The thickness
of the MTZ in our study area ranges from 240 to
293 km, with a mean of 264± 3 km, suggesting
a colder MTZ on average. The MTZ beneath the platform
thickens gradually toward the interior of the
platform, from 240 km to 290 km. This corresponds
to a decrease in temperature of about 380°C.
The variations in d410 and d660
contribute to the thickening of the MTZ approximately
equally, suggesting that the temperature of the
entire MTZ decreases toward the interior of the
platform.
Beneath the lake,
the depth extent of the thickened region (relative
to the platform) suggested by the uplifted d410
cannot be accurately determined due to the lack
of seismic rays that traverse d660. If
we assume that the lateral temperature anomaly
in the entire MTZ is uniformly –550°C,
as suggested by the magnitude of the uplift of
d410, an approximately 30-km depression
of d660 is expected. This is comparable
to the magnitude of the deepening of d660.
Under the assumption that dVp/dT
= -4.1 x 10-4 km/s°C [Anderson,
1989], the increase in P-wave velocity
is about 0.2 km/s, corresponding to a 2% high
velocity anomaly in the MTZ. The observed variation
of the MTZ thickness is consistent with seismic
tomography results. Although most seismic tomography
studies [e.g., Achauer & Masson,
2002; Gao et al., 1994, 2003;
Tiberi et al., 2003] revealed low-velocity
regions in the upper mantle beneath the rift,
there is still a lack of strong evidence for such
anomalies extending to the MTZ. On the contrary,
a recent seismic tomography study by Zhao
et al.
[2006] suggested a high velocity region with
a magnitude of 1-2% in the MTZ beneath the rift.
Figure 4. (A)
depth to d410; (B) depth to d660; (C) MTZ thickness
for each of the azimuthal bins. Green stars are
ray piercing points at 540 km depth. |
The possible mechanism
for the observed transition zone discontinuity topography
is thermal anomalies associated with the rifting process.
The observed uplift of d410 and depression
of d660 beneath the BRZ suggest a MTZ that
is colder than the surrounding areas. The uplift of
d410 rules out the possibility that the rifting
is due to a mantle plume originating below d410.
However, the possibility that the rifting is related
to a plume originating in the upper mantle cannot be
excluded based on existing data. To our knowledge, this
is the first time that a cold MTZ is suggested beneath
a major continental rift, and so far no existing geodynamic
or mineralogical models can explain this unexpected
result. Previous seismic tomography results and the
characteristics of the RFs presented in this study do
not support the hypothesis that the uplift of d410
is caused by excess water in the top of the MTZ. One
possible cause of the low temperature in the MTZ is
that the opening of the rift at about 35 Ma created
a zone of high surface heat flux [Lysak, 1984]
and partial melt (and consequently reduction in seismic
velocity) in the upper mantle beneath the rift. This
model, however, must remain quite speculative until
additional information regarding the thermal history
and thermal properties beneath the study area can be
obtained so that vigorous geodynamic modeling can be
performed.
In summary, using data
from a single broadband station, we have imaged spatial
variation of mantle transition zone discontinuities.
The main features are the unexpected uplift of the d410
and possible depression of d660, suggesting
a reduced temperature and increased velocity with a
magnitude of 2% in the mantle transition zone beneath
the BRZ relative to the southern margin of the Siberian
platform. We also observed a gradual cooling of the
MTZ beneath the Siberian platform toward its interior.
References
-
Achauer, U.,
and F. Masson (2002), Seismic tomography of continental
rifts revisited: from relative to absolute heterogeneities,
Tectonophysics, 358, 17-37.
-
Ammon, C.J.,
G.E. Randall, and G. Zandt (1990), On the non-uniqueness
of receiver function inversions, J. Geophys.
Res., 95, 15,303-15,318.
-
-
Brazier, R.A.,
and A.A. Nyblade (2003), Upper mantle P
velocity structure beneath the Baikal Rift from
modeling regional seismic data, Geophys. Res.
Lett., 30, 1153, doi:10.1029/2002GL016115.
-
Davis, P.M.
(1991), Continental rift structures and dynamics
with reference to teleseismic studies of the Rio
Grande and East African rifts, Tectonophysics,
197, 309-325.
-
Dueker, K.G.,
and A.F. Sheehan (1998), Mantle discontinuity structure
beneath the Colorado Rocky Mountains and High Plains,
J. Geophys. Res., 103,
7153-7169.
-
Efron, B.,
and R. Tibshirani (1986), Bootstrap methods for
standard errors, confidence intervals, and other
measures of statistical accuracy, Stat. Sci.,
1, 54-75.
-
Flanagan,
M.P., and P.M. Shearer (1998), Global mapping of
topography on transition zone velocity discontinuities
by stacking SS precursors, J. Geophys. Res.,
103, 2673-2692.
-
Friederich,
W. (2003), The S-velocity structure of
the East Asian mantle from inversion of shear and
surface waveforms, Geophys. J. Int., 153,
88-102.
-
Gao,
S., P.M. Davis, H. Liu, P.D. Slack, Y.A. Zorin,
V.V. Mordvinova, V.M. Kozhevnikov, and R.P. Meyer
(1994a), Seismic anisotropy and mantle flow beneath
the Baikal rift Zone, Nature, 371,
149-151.
-
Gao,
S., P.M. Davis, H. Liu, P. Slack, Y.A. Zorin, N.A.
Logatchev, M. Kogan, P. Burkholder, and R.P. Meyer
(1994b), Asymmetric upwarp of the asthenosphere
beneath the Baikal rift zone, Siberia, J. Geophys.
Res., 99, 15,319-15,330.
-
Gao,
S., P.M. Davis, H. Liu, P.D. Slack, A.W. Rigor,
Y.A. Zorin, V.V. Mordvinova, V.M. Kozhevnikov, and
N.A. Logatchev (1997), SKS splitting beneath continental
rift zones, J. Geophys. Res., 102,
22,781-22,797.
-
-
Gao,
S.S., K.H. Liu, P.M. Davis, P.D. Slack, Y.A. Zorin,
V.V. Mordvinova, and V.M. Kozhevnikov (2003), Evidence
for small-scale mantle convection in the upper mantle
beneath the Baikal rift Zone, J. Geophys. Res.,
108, 2194, doi:10.1029/2002JB002039.
-
-
Keller, G.R.,
M. Bott, R.F. Wendlandt, D.I. Doser, and P. Morgan
(1995), The Baikal rift system, in Continental
rifts; evolution, structure, tectonics, Elsevier,
Amsterdam.
-
-
Logatchev,
N.A., and Y.A. Zorin, Y.A. (1992), Baikal rift zone
- structure and geodynamics, Tectonophysics,
208, 273-286.
-
Lysak, S.V.
(1984), Terrestrial heat flow in the south of east
Siberia, Tectonophysics, 103,
205-215.
-
-
Shen,
Y., S.C. Solomon, I. Th. Bjarnason, G. Nolet, W.J.
Morgan, R.M. Allen, K. Vogfjord, S. Jakobsdottir,
R. Stefansson, B.R. Julian, and G.R. Foulger (2002),
Seismic evidence for a tilted mantle plume and north-south
mantle flow beneath Iceland, Earth Planet. Sci.
Lett., 197, 261-272.
-
Slack, P.D.,
P.M. Davis, W.S. Baldridge, K.H. Olsen, A. Glahn,
U. Achauer, and W. Spence (1996), The upper mantle
structure of the central Rio Grande Rift region
from teleseismic P and S wave travel time delays
and attenuation, J. Geophys. Res., 101,
16,003-16,023.
-
Tiberi, C.,
M. Diament, J. Deverchere, C. Petit-Mariani, V.
Mikhailov, S. Tikhotsky, and U. Achauer (2003),
Deep structure of the Baikal rift zone revealed
by joint inversion of gravity and seismology, J.
Geophys. Res., 108, 2133,
doi: 10.1029/2002JB001880.
-
Wilson, D.,
R. Aster, M. West, J. Ni, S. Grand, W. Gao, W.S.
Baldridge, S. Semken, and P. Patel (2005), Lithospheric
structure of the Rio Grande rift, Nature,
433, 851-855.
-
-
Zorin, Y.A.,
V.M. Kozhevnikov, M.R. Novoselova, and E.K. Turutanov
(1989), Thickness of the lithosphere beneath the
Baikal rift zone and adjacent regions, Tectonophysics,
168, 327-337.
-
Zorin, Y.A.,
V.V. Mordvinova, G.L. Kosarev, E.K.Turutanov, B.G.
Belichenkoa, and S.S. Gao (2002), Low seismic velocity
layers in the Earth's crust of Eastern Siberia (Russia)
and Mongolia, Receiver function data and geological
implication, Tectonophysics, 359,
307-327.
Discussion
Mon Dec 18th, 2006: Alexei Ivanov
The paper by Liu & Gao is very interesting
and, I believe, it will give new impetus to the discussion
of the Baikal rift origin. I wish to comment on one
sentence from their webpage.
"One possible cause of the low
temperature in the MTZ [beneath the rift] is that
the opening of the rift at about 35 Ma created a zone
of high surface heat flux [Lysak, 1984] and
partial melt (and consequently reduction in seismic
velocity) in the upper mantle beneath the rift."
The authors admit that this is a speculative
idea. Indeed, it just cannot be correct. There is
no evidence of 35 Ma old volcanism within the rift.
The oldest volcanic rocks close to the south Baikal
(a few km away from Talaya seismic station used by
Liu & Gao) are only 18 Ma old. The oldest rocks
in the broader area are not much older (about 22 Ma).
I attach a recent paper (Rasskazov
et al., 2002), which includes Ar/Ar and K-Ar ages
for the South Baikal and Khubsugul lake areas. The
puzzle about the Baikal rift is: "Why there is
there no volcanism within the deepest rift basins
of Lake Baikal?". The same question can be asked
regarding a recent paper by Lebedev et al. (Asthenospheric
flow and origin of volcanism in the Baikal Rift area,
Earth Planet. Sci. Lett., 249,
415-424, 2006.). Any model MUST consider the fact
that volcanism seems to be independent of extension
in the Baikal rift. There is, however, the influence
of pre-existing lithospheric structure on rifting
and localization of volcanism.
As for the cold MTZ beneath the Baikal,
the easiest solution, in my opinion, is the presence
of a Mesozoic slab there. The authors consider this
possibility in their paper, but it seems they do not
favour it.
Back
last updated 18th
December, 2006 |