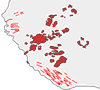 |
Supercontinental inheritance and its influence on breakup: The Central Atlantic Magmatic Province & Pangea |
Lisa Whalen1, Esteban Gazel1, Christopher Vidito2, John Puffer3, Michael Bizimis4, William Henika1, and Mark J. Caddick1
1Department of Geosciences, Virginia Tech, Blacksburg, Virginia, USA; lashley@vt.edu ; egazel@vt.edu ; bhenika@vt.edu ; caddick@vt.edu
2Department of Earth and Planetary Sciences, Rutgers University, Piscataway, New Jersey, USA; cvidito@eden.rutgers.edu
3Department of Earth and Environmental Sciences, Rutgers University, Newark, New Jersey, USA; jpuffer@andromeda.rutgers.edu
4Department of Earth and Ocean Sciences, University of South Carolina, Columbia, South Carolina, USA; mbizimis@geol.sc.edu
This webpage is a summary of: Whalen, Lisa, Esteban Gazel, Christopher Vidito, John Puffer, Michael Bizimis, William Henika and Mark J. Caddick, Supercontinental inheritance and its influence on supercontinental breakup: The central Atlantic magmatic province and the break up of Pangea, Accepted manuscript online: 3 SEP 2015 11:34AM EST | DOI: 10.1002/2015GC005885
Introduction
The mechanism that triggers supercontinent breakup is a missing piece in plate tectonics theory. We investigated mechanisms by examining the relationship between the breakup of Pangea and the formation of the Central Atlantic Magmatic Province (CAMP) large igneous province (LIP) [Marzoli et al., 1999]. The primary objective was to develop a plausible mechanism of formation for CAMP that explains its size, petrological estimates of low mantle potential temperature, and geochemical signatures. We bring together geochemical data from CAMP lavas with other geological information such as the rifting record of the Triassic basins along the East coast of North America [Schlische et al., 2003] and the tectonic history of the formation of Pangea. Recent seismic work by Benoit et al. [2014], combined with the other geologic evidence, suggests that the breakup of the previous supercontinent, Rodinia, may have affected the formation and breakup of Pangea, through a process we will call supercontinental inheritance.
The problem
The formation mechanism for LIPs is debated [King & Anderson, 1995; Lustrino, 2005; Campbell, 2007], though many workers attempt to explain CAMP with the mantle plume model [e.g., May, 1971; Morgan, 1983; Anderson, 1982; White & McKenzie, 1989; Hill, 1991; Wilson, 1997; Courtillot et al., 1999; Ernst & Buchan, 2002; Fokin, 2003]. In addition to a lack of geochemical evidence for a plume being involved with CAMP [McHone, 2000; Puffer, 2001], calculated mantle potential temperatures are too low [Herzberg & Gazel, 2009]. In addition, there is no volcanic trail leading away from the LIP [McHone, 2000]. As the plume model falls short in explaining this particular LIP, other mechanisms such as edge-driven convection [King & Anderson, 1995] have been suggested [McHone, 2000; Deckart et al., 2005; Merle et al., 2011]. In this study we investigated the potential of lithospheric delamination to explain CAMP.
Rodinia breakup
Structural elements formed during the breakup of Rodinia may have affected the formation of Pangea. During Rodinia breakup at ~540 Ma [McClellan & Gazel, 2014] the Scranton Rift basin formed. It is now buried in the subsurface on the East coast of North America [Benoit et al., 2014] (Figure 1). The resistant material of the Scranton Rift blocked the advancement of thrust sheets during the final closure of Pangea [Benoit et al., 2014] and may have caused the orientation of subduction and collision between Gondwana and Laurentia to switch from oblique in the North to orthogonal in the South [Hatcher, 2002]. We divide the CAMP samples used in this study on the basis of their geographical location relative to the Scranton Rift. Northern CAMP consists of lavas emplaced to the north of the Scranton Rift, with southern CAMP denoting lavas emplaced to the south of the Scranton Rift. CAMP lavas emplaced adjacent to the Scranton Rift are termed transitional CAMP.
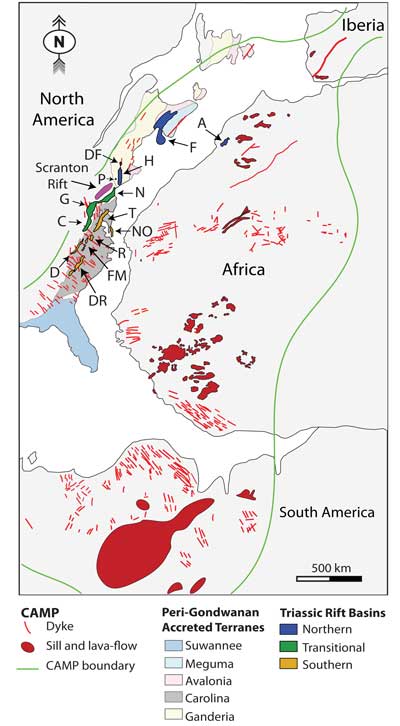
Figure 1: Map of CAMP exposures modified from Deckart et al. [2005]. CAMP boundary after McHone [2003]. Letters indicate Mesozoic rift-basin locations from Schlische et al. [2003] and Olsen [1997]. Accreted terrane boundaries from Hibbard et al, [2010] and Hatcher [2010]. Scranton Rift location from Benoit et al. [2014].
Geochemistry
The accretionary events that led to the formation of Pangea [Hatcher, 2010] may have affected the geochemistry of the sublithospheric mantle which, in turn, may have affected the geochemisty of CAMP lavas [Pegram, 1990; Puffer, 2001, 2003; De Min et al., 2003; Deckart et al., 2005; Dorais & Tubrett, 2008; Merle et al., 2011, 2013; Murphy et al., 2011; Callegaro et al., 2013]. These accretionary events are not evenly distributed along the margin. More sustained subduction occurred in the North, in the New England area [Hibbard et al., 2010]. We present geochemical evidence that CAMP lavas have an inherited arc-modified signature, from trace-element and radiogenic isotope ratios (Figure 2). Northern and transitional CAMP, more so than southern CAMP lavas, show more evidence of being produced from a mantle source that has been modified by subduction, possibly through the interaction between subducting sediment and peridotite. We rule out assimilation fractional crystallization (AFC) as an explanation for the geochemical composition of our samples through AFC modeling (details in the supplementary information). This is further supported by low mantle-like Os isotope ratios reported by regional studies [Merle et al., 2011; Callegaro et al., 2013].
In order to assess the source composition of CAMP we performed high-precision analyses of the most primitive olivine phenocrysts from basalts (in olivine control) and a modified method from Sobolev et al. [2007]. Our results show that CAMP olivine phenocryts have higher Ni and lower Ca than expected for olivines crystallized from a melt derived from a purely peridotite source (Figure 3) [Sobolev et al., 2007; Herzberg, 2011]. Thus, the source of CAMP had a significant pyroxenite component [Sobolev et al., 2007; Herzberg, 2011] that may have formed through reaction between melted sediment and peridotite. This further supports the hypothesis that CAMP basalts have an inherited subduction signature.
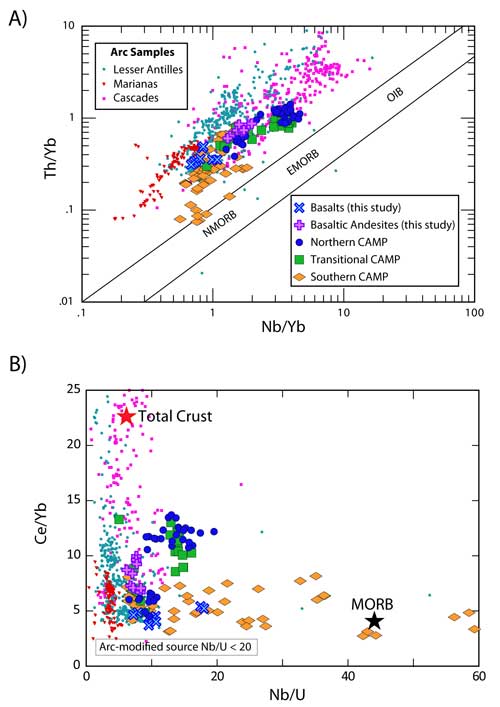
Figure 2: Trace-element comparison of northern, southern and transitional CAMP. Northern and transitional CAMP samples bear closer resemblance to arc-derived samples than southern CAMP.
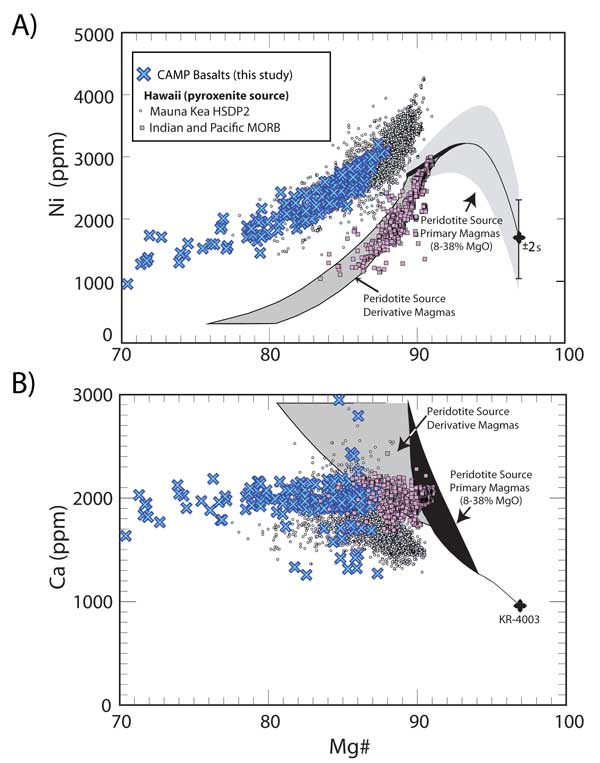
Figure 3: Trace-element data from CAMP olivine phenocrysts plotted versus Mg#. Diagrams modified from Herzberg [2011]. Additional data from Sobolev et al. [2007].
Final formation of CAMP
Initiation of the breakup of Pangea and the formation of CAMP may have been directly influenced by the breakup of Rodinia in the form of the Scranton Rift. As the final step in the formation of Pangea, Gondwana collided with Laurentia as the Rheic plate subducted beneath Laurentia. Subduction and collision were at an oblique angle [Hatcher, 2002] until Gondwana collided with the Scranton Rift and could go no further [Benoit et al., 2014]. Pivoting about the Scranton Rift, Gondwana swung around clockwise colliding head-on in the south [Hatcher, 2010]. The change in subduction direction due to the presence of the Scranton Rift in the subsurface, as well as flat-slab subduction, may have led to the underplating of Rheic slab beneath Laurentia. After increased pressure and temperature over ~40 Ma the slab eclogitized eventually delaminating and sinking into the mantle (Figure 4). This caused the upwelling of mantle that formed CAMP and triggered rifting in the north.
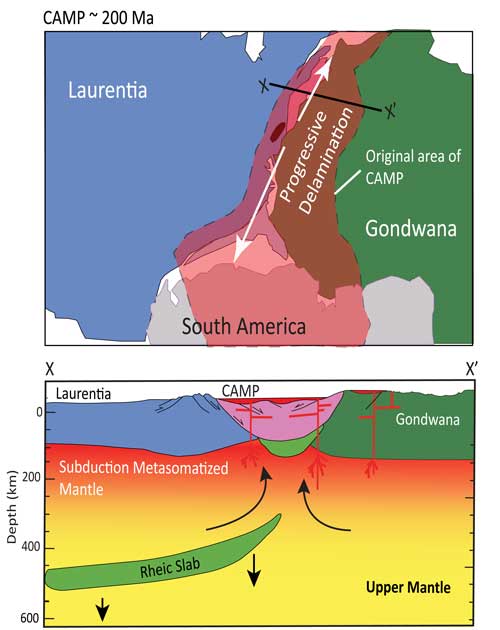
Figure 4: Cartoon showing the delamination of the Rheic plate and the formation of CAMP. Map of Pangea after Hatcher [2002]. CAMP boundary after McHone [2003]. Scranton Rift location from Benoit et al. [2014]. Accreted terrane boundaries from Hibbard et al, [2010] and Hatcher [2010].
References
-
Anderson, D. L. (1982), Hotspots, polar wander, Mesozoic convection and the geoid, Nature, 297, 391-393.
-
Benoit, M. H., C. Ebinger, and M. Crampton (2014), Orogenic bending around a rigid Proterozoic magmatic rift beneath the Central Appalachian Mountains, Earth and Planetary Science Letters, 402, 197–208.
-
Callegaro, S., A. Marzoli, H. Bertrand, M. Chiaradia, L. Reisberg, C. Meyzen, G. Bellieni, R. E. Weems, and R. Merle (2013), Upper and lower crust recycling in the source of CAMP basaltic dykes from southeastern North America, Earth and Planetary Science Letters, 376, 186-199.
-
Campbell, I. H. (2007), Testing the plume theory, Chemical Geology, 241, 153-176.
-
Courtillot, V., C. Jaupart, I. Manighetti, P. Tapponnier, and J. Besse (1999), On causal links between flood basalts and continental breakup, Earth and Planetary Science Letters, 166, 177-195.
-
De Min, A., E. M. Piccirillo, A. Marzoli, G. Bellieni, P. R. Renne, M. Ernesto, and L. S. Marques (2003), The Central Atlantic Magmatic Province (CAMP) in Brazil: petrology, geochemistry, 40Ar/39Ar ages, paleomagnetism and geodynamic implications, The central Atlantic magmatic province: insights from fragments of Pangea, Geophysical Monograph Series 136, 91-128, DOI: 10.1029/136GM06.
-
Deckart, K., H. Bertrand, and J.-P. Liégeois (2005), Geochemistry and Sr, Nd, Pb isotopic composition of the Central Atlantic Magmatic Province (CAMP) in Guyana and Guinea, Lithos, 82, 289-314.
-
Dorais, M. J., and M. Tubrett (2008), Identification of a subduction zone component in the Higganum dike, Central Atlantic Magmatic Province: A LA-ICPMS study of clinopyroxene with implications for flood basalt petrogenesis, Geochemistry, Geophysics, Geosystems, 9(10).
-
Ernst, R. E., and K. L. Buchan (2002), Maximum size and distribution in time and space of mantle plumes: evidence from large igneous provinces, Journal of Geodynamics, 34, 309-342.
-
Fokin, M. A. (2003), Space-time analysis of magmatism: the igneous record for an early Cryogenian plume track in central Appalachian orogen, M.Sc. thesis, Virginia Polytechnic Institute and State University, vii+67pp.
-
Hatcher, R. D. (2002), Alleghanian (Appalachian) orogeny, a product of zipper tectonics: Rotational transpressive continent-continent collision and closing of ancient oceans along irregular margins, Geological Society of America Special Paper 364, 199-208.
-
Hatcher, R. D. (2010), The Appalachian orogen: A brief summary, Geological Society of America Memoirs, 206, 1-19.
-
Herzberg, C. (2011), Identification of source lithology in the Hawaiian and Canary Islands: Implications for origins, Journal of Petrology, 52, 113-146.
-
Herzberg, C., and E. Gazel (2009), Petrological evidence for secular cooling in mantle plumes, Nature, 458, 619-622.
-
Hibbard, J. P., C. R. van Staal, and D. W. Rankin (2010), Comparative analysis of the geological evolution of the northern and southern Appalachian orogen: Late Ordovician-Permian, Geological Society of America Memoirs, 206, 51-69.
-
Hill, R. I. (1991), Starting plumes and continental break-up, Earth and Planetary Science Letters, 104, 398-416.
King, S. D., and D. L. Anderson (1995), An alternative mechanism of flood basalt formation, Earth and Planetary Science Letters, 136, 269-279.
-
Lustrino, M. (2005), How the delamination and detachment of lower crust can influence basaltic magmatism, Earth-Science Reviews, 72, 21-38.
-
Marzoli, A., P. R. Renne, E. M. Piccirillo, M. Ernesto, G. Bellieni, and A. D. Min (1999), Extensive 200-Million-Year-Old Continental Flood Basalts of the Central Atlantic Magmatic Province, Science, 284, 616-618.
-
May, P. R. (1971), Pattern of Triassic-Jurassic diabase dikes around the North Atlantic in the context of predrift position of the continents, Geological Society of America Bulletin, 82, 1285-1292.
-
McClellan, E., and E. Gazel (2014), The Cryogenian intra-continental rifting of Rodinia: Evidence from the Laurentian margin in eastern North America, Lithos, 206–207, 321-337.
-
McHone, J. G. (2000), Non-plume magmatism and rifting during the opening of the central Atlantic Ocean, Tectonophysics, 316, 9.
-
McHone, J. G. (2003), Volatile emissions from Central Atlantic Magmatic Province Basalts: Mass assumptions and environmental consequences, 136, 241-254.
-
Merle, R., A. Marzoli, H. Bertrand, L. Reisberg, C. Verati, C. Zimmermann, M. Chiaradia, G. Bellieni, and M. Ernesto (2011), 40Ar/39Ar ages and Sr–Nd–Pb–Os geochemistry of CAMP tholeiites from Western Maranhão basin (NE Brazil), Lithos, 122, 137-151.
-
Merle, R., A. Marzoli, L. Reisberg, H. Bertrand, A. Nemchin, M. Chiaradia, S. Callegaro, F. Jourdan, G. Bellieni, and D. Kontak (2013), Sr, Nd, Pb and Os isotope systematics of CAMP tholeiites from Eastern North America (ENA): Evidence of a subduction-enriched mantle source, Journal of Petrology, 55, 133-180.
-
Morgan, W. J. (1983), Hotspot tracks and the early rifting of the Atlantic, Tectonophysics, 94, 123-139.
-
Murphy, J. B., J. Dostal, G. Gutiérrez-Alonso, and J. D. Keppie (2011), Early Jurassic magmatism on the northern margin of CAMP: Derivation from a Proterozoic sub-continental lithospheric mantle, Lithos, 123, 158-164.
-
Olsen, P. E. (1997), Stratigraphic record of the early Mesozoic breakup of Pangea in the Laurasia-Gondwana rift system, Annual Review of Earth and Planetary Sciences, 25, 337-401.
-
Pegram, W. J. (1990), Development of continental lithospheric mantle as reflected in the chemistry of the Mesozoic Appalachian tholeiites, USA, Earth and Planetary Science Letters, 97, 316-331.
-
Puffer, J. H. (2001), Contrasting high field strength element contents of continental flood basalts from plume versus reactivated-arc sources, Geology, 29, 675-678.
-
Schlische, R. W., M. O. Withjack, and P. E. Olsen (2003), Relative timing of CAMP, rifting, continental breakup, and basin inversion: tectonic significance, The Central Atlantic Magmatic Province: Insights from Fragments of Pangea, Geophysical Monograph Series 136, 33-59.
-
Sobolev, A. V., et al. (2007), The Amount of Recycled Crust in Sources of Mantle-Derived Melts, Science, 316, 412-417.
-
White, R., and D. McKenzie (1989), Magmatism at Rift Zones: The Generation of Volcanic Continental Margins and Flood Basalts, Journal of Geophysical Research, 94(B6), 44.
-
Wilson, M. (1997), Thermal evolution of the Central Atlantic passive margins: continental break-up above a Mesozoic super-plume, Journal of the Geological Society, 154, 491-495.
last updated 24th
November, 2015 |