Catherine Annen1, Rais Latypov2, Sofya Chistyakova2, Alexander R. Cruden3 & Troels F. D. Nielsen4
1Institute of Geophysics of the CAS, Prague 4, Czech Republic; annen@ig.cas.cz
2School of Geosciences, University of the Witwatersrand, Johannesburg, South Africa; rais.latypov@wits.ac.za ; sofia.chistyakova@wits.ac.za
3School of Earth, Atmosphere and Environment, Monash University, Melbourne, Australia; Sandy.Cruden@monash.edu
4Department of Petrology and Economic Geology, Geological Survey of Denmark and Greenland, Copenhagen, Denmark; tfn@geus.dk
This webpage is a summary of: Annen, C., Latypov, R., Chistyakova, S., Cruden, A. R., and Nielsen, T. F. D. Catastrophic growth of totally molten magma chambers in months to years, Science Advances 8, 38, eabq0394.
The ca. 55 Ma Skaergaard layered mafic intrusion (Eastern Greenland) is characterized by an onion skin-like mineralogical structure (Nielsen, 2004; Salmonsen & Tegner, 2013; Hagen-Peter et al., 2019; Nielsen et al., 2019b). Cumulus phases appear and disappear, and mineral compositions evolve in the same way from the vertical side walls, horizontal roof and floor of the intrusion, which indicates that the ~300 km3 of the Skaergaard magma body differentiated from a parental liquid under closed system conditions (Figure 1). This implies that the Skaergaard intrusion was a large, fully liquid (<< 1 wt% crystals) magma chamber before it started to crystallize. Such a large magma chamber requires very rapid injection of melt in order to prevent any crystallization from occurring before emplacement is completed (Annen, 2009).

Figure 1: Geological and stratigraphic cross sections of the Skaergaard layered intrusion, East Greenland. The Skaergaard intrusion is subdivided into three major petrological units: the Layered Series (LS), Upper Border Series (UBS), and Marginal Border Series (MBS), which crystallized concurrently along essentially parallel trends of differentiation. The LS formed above the floor, while the UBS crystallized below the roof and the MBS solidified inward from the vertical walls of the intrusion. (A) E–W cross section through the Skaergaard intrusion (B) The two inward-migrating crystallization fronts converged at the Sandwich Horizon (SH) as indicated by the mirror images of the cumulus phase arrivals and An-content of plagioclase in the LS and UBS. Modified from Hagen-Peter et al. (2019) and Nielsen et al. (2019b) with some compositional points for the Hidden Zone (HZ) from Holness et al. (2015). LZ – Lower Zone, MZ – Main Zone, UZ – Upper Zone, SL – Sea Level; Ol, olivine, Aug, augite; Mt, magnetite, Ap, apatite, Bu, (ferro)bustamite. An-content=100An/(An+Ab).
We used numerical simulations of magma emplacement, heat transfer and cooling to determine permissible timescales required to fill the Skaergaard intrusion before the occurrence of significant crystallization. In our simulations, magma was emplaced as layers upwards from the chamber floor and the temperatures and crystal fractions in the intrusion were calculated. The variables were the time intervals between the emplacement of magma batches, the initial temperatures of the country rocks and possible superheating of the injected magma. We approximated the possible effect of heat transfer by magma convection by homogenizing the heat content in magmas with < 10 wt% crystals. The cooling effect of hydrothermal convection in the country rock was neglected, which makes our results conservative.
The numerical simulations indicate that to avoid partial crystallization before full emplacement the Skaergaard magma chamber must have been emplaced in years to decades, with a vertical growth rate of a few 100 m/yr (Figure 2). Slower emplacement rates result in the formation of a crystal-rich magma chamber or partial solidification of the magma body before the end of emplacement. The robustness of the results and the validity of the approach are supported by a good fit between modelled temperature profiles in the country rock and temperature estimates obtained from rocks in the contact metamorphic aureole of the Skaergaard intrusions reported in the literature (Figure 3).
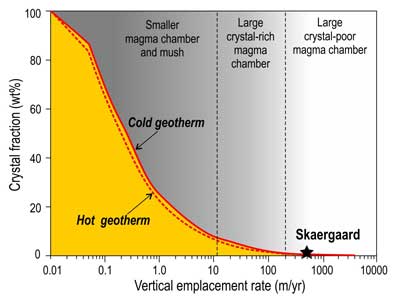
Figure 2. Crystal fraction (wt%) versus vertical magma emplacement rate (m/yr) in the Skaergaard intrusion, East Greenland. Numerical simulations show that the minimum vertical emplacement rate required for a large, crystal-free chamber such as Skaergaard to grow without the onset of crystallization along its margins must be on the order of several 100 to a few 1000 m/yr.
According to the model, the volumetric flow rate of magma into the Skaergaard magma chamber must have been 10s to 100s of km3/yr, which is several orders of magnitude faster than emplacement rates reported for other intrusive bodies (Crisp, 1984; de Saint Blanquat et al., 2011; Leuthold et al., 2012; Menand et al., 2015). This suggests that events of the type of Skaergaard intrusion are rare and catastrophic. We propose that such rapid accumulations of magma are accommodated by subsidence of the floor rocks along faults that may have been lubricated by magma (Cruden, 1998; Cruden & Weinberg, 2018).
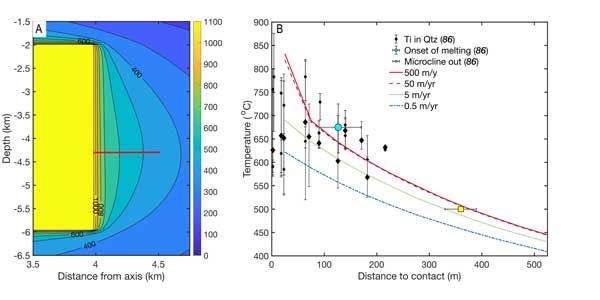
Figure 3. Computed thermal aureole in models of the Skaergaard intrusion. (A) Maximum temperatures reached for an intrusion emplacement rate of 500 m/yr. The horizontal red line shows the location of the temperature profiles in (B). (B) Maximum temperatures in the country rock. The curves plot the results of numerical simulations for different vertical emplacement rates. The symbols plot temperature estimates obtained by Bufe et al. (2014a) from Titanium in Quartz thermometry, the onset of country rock melting and the microcline out isotherm.
The Skaergaard chamber was filled by magma that must have been extracted from a deeper reservoir during subsidence of the the crustal block below the intrusion. Mafic dikes tens to hundreds of meters wide and several kilometers long are coeval with the Skaergaard intrusion (Brooks & Nielsen, 1978; Nielsen et al., 2019a). Assuming reasonable values for magma density and viscosity, dikes with these dimensions can transport magma at volumetric flow rates of 10s to 100s km3/yr, matching our calculated estimates of the filling rate of the Skaergaard intrusion. Contemporary East Greenland lavas and dykes of appropriate bulk compositions are all aphyric.
Despite differences in magma compositions and tectonic context, some aspects of the Skaergaard intrusion are similar to caldera-forming super-eruptions: they represent rare catastrophic events involving rapid transfer of magma along faults that accommodate subsidence of rocks below the caldera or intrusion. The Skaergaard intrusion is part of a Large Igneous Province (LIP) that formed during the opening of the North Atlantic. The high intrusion rates that we estimate for the Skaergaard magma chamber at a depth of a few kilometers are commensurate with the high eruptive fluxes of basalts observed at the surface in LIPs (Swanson et al., 1975; Reidel, 1998; Reidel et al., 2019; Biasi & Karlstrom, 2021). We conclude that some layered mafic intrusions are the plutonic equivalent of LIP-related volcanoes that rapidly erupt large volumes of basaltic magma on the Earth’s surface.
References
- Annen, C., 2009, What are the magma fluxes required to build up magma chambers large enough to feed major ignimbrite eruptions? Eos Transactions AGU, 90, Fall Meet. Suppl., Abstract V21G-04.
- Biasi, J., and L. Karlstrom, 2021, Timescales of magma transport in the Columbia River flood basalts, determined by paleomagnetic data: Earth and Planetary Science Letters, 576, 117169.
- Brooks, C. K., and T. F. D. Nielsen, 1978, Early stages in the differentiation of the Skaergaard magma as revealed by a closely related suite of dike rocks: Lithos, 11, 1–14.
- Bufe, N. A., M. B. Holness, and M. C. S. Humphreys, 2014, Contact Metamorphism of Precambrian Gneiss by the Skaergaard Intrusion: Journal of Petrology, 55, 1595–1617.
- Crisp, J. A., 1984, Rates of magma emplacement and volcanic output: Journal of Volcanology and Geothermal Research, 20, 177–211.
- Cruden, A. R., 1998, On the emplacement of tabular granites: Journal of the Geological Society, 155, 853–862.
- Cruden, A. R., and R. F. Weinberg, 2018, Chapter 2 - Mechanisms of Magma Transport and Storage in the Lower and Middle Crust—Magma Segregation, Ascent and Emplacement, in S. Burchardt, ed., Volcanic and Igneous Plumbing Systems, Elsevier, 13–53.
- Hagen-Peter, G., C. Tegner, and C. E. Lesher, 2019, Strontium isotope systematics for plagioclase of the Skaergaard intrusion (East Greenland): A window to crustal assimilation, differentiation, and magma dynamics: Geology, 47, 313–316.
- Holness, M. B., C. Tegner, O. Namur, and L. Pilbeam, 2015, The earliest history of the Skaergaard magma chamber: a textural and geochemical study of the Cambridge drill core: Journal of Petrology, 56, 1199–1227.
- Leuthold, J., O. Müntener, L. P. Baumgartner, B. Putlitz, M. Ovtcharova, and U. Schaltegger, 2012, Time resolved construction of a bimodal laccolith (Torres del Paine, Patagonia): Earth and Planetary Science Letters, 325–326, 85–92.
- Menand, T., C. Annen, and M. de Saint Blanquat, 2015, Rates of magma transfer in the crust: Insights into magma reservoir recharge and pluton growth: Geology, 43, 199–202.
- Nielsen, T. F. D., 2004, The Shape and Volume of the Skaergaard Intrusion, Greenland: Implications for Mass Balance and Bulk Composition: Journal of Petrology, 45, 507–530.
- Nielsen, T. F. D., C. K. Brooks, and J. K. Keiding, 2019a, Bulk Liquid for the Skaergaard Intrusion and Its PGE-Au Mineralization: Composition, Correlation, Liquid Line of Descent, and Timing of Sulphide Saturation and Silicate–Silicate Immiscibility: Journal of Petrology, 60, 1853–1880.
- Nielsen, T. F. D., N. S. Rudashevsky, V. N. Rudashevsky, S. M. Weatherley, and J. C. Ø. Andersen, 2019b, Elemental Distributions and Mineral Parageneses of the Skaergaard PGE–Au Mineralization: Consequences of Accumulation, Redistribution, and Equilibration in an Upward-Migrating Mush Zone: Journal of Petrology, 60, 1903–1934.
- Reidel, S., T. Tolan, and V. Camp, 2019, Columbia River flood basalt flow emplacement rates—Fast, slow, or variable?, in Field Volcanology: A Tribute to the Distinguished Career of Don Swanson, Geological Society of America Special Paper 538, https://doi.org/10.1130/2018.2538(01)
- Reidel, S. P., 1998, Emplacement of Columbia River flood basalt: Journal of Geophysical Research, 103, 27393–27410.
- de Saint Blanquat, M., E. Horsman, G. Habert, S. Morgan, O. Vanderhaeghe, R. Law, and B. Tikoff, 2011, Multiscale magmatic cyclicity, duration of pluton construction, and the paradoxical relationship between tectonism and plutonism in continental arcs: Tectonophysics, 500, 20–33.
- Salmonsen, L. P., and C. Tegner, 2013, Crystallization sequence of the Upper Border Series of the Skaergaard Intrusion: revised subdivision and implications for chamber-scale magma homogeneity: Contributions to Mineralogy and Petrology, 165, 1155–1171.
- Swanson, D. A., T. L. Wright, and R. T. Helz, 1975, Linear vent systems and estimated rates of magma production and eruption for the Yakima Basalt on the Columbia Plateau: American Journal of Science, 275, 877–905.
last updated 21st December, 2022 |