 |
|
The
Deccan Volcanic Province:
Thoughts about its genesis |
|
|
Click here to
go to Discussion of this page
Click here to
download a PDF version of this webpage
|
1.
Impetus for this
contribution
The Deccan volcanic
province (DVP) is one of the world's largest LIPs
and perhaps the best studied continental flood basalt
(CFB). However, its genesis and evolution are still
poorly understood.
Recently, Sheth
(1999) convincingly refuted the plume model as a basis
for the genesis of the DVP (see The
Deccan beyond the plume hypothesis). However,
his suggestion that the DVP and Laccadive-Chagos ridge
formed as a consequence of southward crack propagation
along the Vishnu fracture zone is not consistent with
data concerning the geomagmatic and tectonic history
of the Indian peninsular plate. The current status
of knowledge thus represents a shortfall of understanding.
The need to fill this gap in our knowledge, and to
establish a genetic model for the DVP based on the
vast volumes of existing scientific data, was the
impetus for the present contribution.
2.
Introduction
The DVP is one of the
Earth's giant continental flood basalts and has a
total exposed area of about half a million square
kilometers, between latitudes 16° -
24° N and longitudes 70° - 77° E.
In the northwestern, central and southern Indian peninsula,
the approximate volume of the DVP is about 2 x 106
km3 and its estimated age is 64-65 Ma.
It is generally believed that the DVP originated during
Gondwanaland breakup as part of the Seychelles-India
separation event. Another important belief concerning
this CFB is that it is the “head” of a
plume which is currently active as Reunion volcanism,
with the “tail” consisting of the rather
irregular chain of volcanic islands extending from
Reunion to and along the Laccadive-Maldive-Chagos
ridges. The latter model has been refuted convincingly,
as mentioned above. However, a viable alternative
model has not yet been proposed. In this contribution
we attempt to provide an alternative hypothesis based
on existing geological information about the DVP.
3.
The Deccan volcanic province:
existing scientific data
The DVP erupted on the
Archean-Proterozoic shield areas of south, north-west
and central India and the adjoining offshore area
off the west coast (Figure 1) (Devey & Stephens,
1991). The volcanics cover two cratonic areas –
the Dharwar craton of the south Indian shield and
the central Indian craton. Apart from this, the DVP
is associated with four major rift zones of peninsular
India (Figure 1). It is juxtaposed with the east-west-running
Narmada-Satpura-Tapi rift which is a horst-and-graben-type
rift zone that trends ENE-WSW for > 1600 km along
central India (Mishra, 1977). In northwest
India, the DVP is in contact with the Cambay, Kutch
and West Coast rifts.
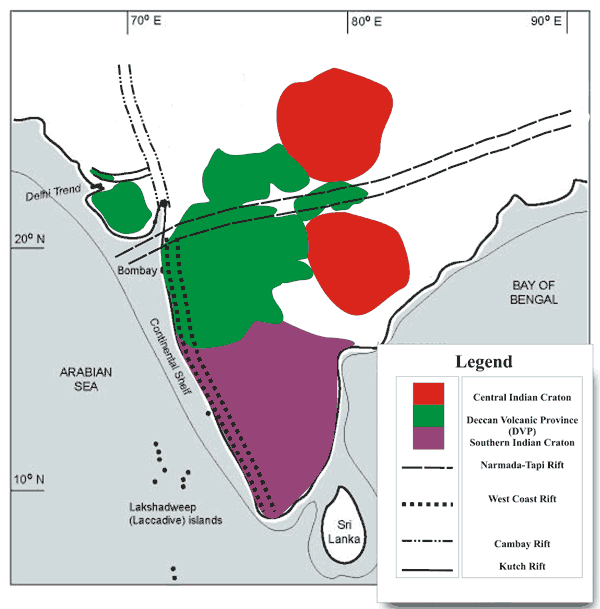
Figure 1: Geological map showing
the location of the Deccan volcanic province and its
relationship with the geo-tectonic features of the
region. Modified after Sheth (2005).
To evaluate the causes
of DVP genesis, it is essential to understand the
geological context of its hosts. Therefore, to interpret
the data from the Deccan volcanics, we first review
the nature of the hosts.
Along the West Coast
rift, the south Indian shield witnessed several
prior phases of magmatism before it hosted the Deccan
volcanics. The first recorded event occurred at 678
Ma when gabbro, granophyre and anorthosite magmatism
occurred (Nair & Vidyadharan, 1982). This
was followed by granitic plutonism, almost 128 My
later, i.e., at 550 Ma (Soman et al.,
1983). Subsequently, the region hosted pegmatitic
intrusions at 460 Ma (Soman et al., 1982).
These pegmatites mark the end of the major phases
of the magmatic episode because there was then a complete
hiatus of magmatic events in the region until 93 Ma
(date averaged from six differing K-Ar dates), i.e.,
for more than 350 My! At 93 Ma, the West Coast region
again experienced magmatism, this time involving rhyolitic
and dacitic volcanics (Valsangkar et al., 1980).
This was followed by vast mafic volcanism and plutonism
which resulted in the DVP and associated dyke swarms
during the period 64-65 Ma, and covered the huge area
mentioned above.
Along the Narmada-Tapi
rift zone, prior to the Deccan episode, no major
magmatic event is reported. Instead, the horst (Satpura)
and grabens (Narmada and Tapi) paved the way for enormous
sedimentation, which gave rise to the Mahakoshal group
of rocks (Chanda & Bhattacharya, 1966).
These metasediments recorded several phases of shearing
implying that the Narmada – Tapi rift is associated
with intense shear deformation along an east-west
trend. These metasediments are followed by Jurassic-early
Cretaceous sediments on top of which the Deccan volcanics
lie (Chanda & Bhattacharya, op.cit).
The tectonics of
the northwest Indian peninsula and the offshore region
are inter-related. The summary of the tectonic
history of this region given here is based on the
studies of several workers (Glennie, 1932;
Qureshy, 1971; Owen, 1976; Kaila
et al., 1979; 1981; Biswas, 1982; 1987;
Harbison & Bassinger, 1973; Gupta et
al., 1998). As shown in Figure 1, four major rift
zones are in contact with the Deccan volcanics, the
Narmada-Tapi rift, the West Coast rift, the Cambay
rift and the Kutch rift. Kutch rifting occurred in
the late Triassic to early Jurrasic followed by early
Cretaceous Cambay rifting. The Narmada-Tapi and West
Coast rifts were reactivated in the late Cretaceous.
The Narmada-Tapi rift zone is believed to be extending
along its trend into the offshore area of the Indian
west coast.
Concerning geophysics
(Chandrasekharam, 1985), the Bouguer gravity
anomaly pattern and seismic profiles along the West
Coast rift indicate:
-
thinning
of the continental crust along the western coast.
This implies delamination of the lithosphere beneath
the west coast,
-
rifting
of the coast in a horst-and-graben pattern, and
-
shear displacement
of the West Coast fault.
Deep Seismic Sounding
(DSS) investigations have been carried out in the
Indian peninsula (Reddy et al., 1999). This
study indicates that the crust seems to become thinner
(24 km) towards the northern parts of the west coast,
that is north of 15°N. The west coast was also
characterized by upwarp of the Moho during the late
Cretaceous period.
Vertical crustal movements
are recognized along the West Coast rift, and shear
displacement along the Narmada-Tapi rift zone and
its extension into the offshore areas (Biswas,
1982).
4.
The new proposed hypothesis: an effort to bridge the
gap in knowledge
4.A:
The first phase of magmatism, 678 to 460 Ma: Of
the four rifts, only the West Coast rift exclusively
hosts magmatic rocks older than the Deccan volcanic
event at 64-65 Ma. These magmatic rocks, which formed
at 678-460 Ma, show a clear trend of fractional melting
(gabbro-rhyolite to pegmatites). This implies the
presence of a magma chamber beneath the Indian lithosphere
under the west coast. In this magma chamber, magma
could have remained stored and preserved its primary
chemistry. The primary magma could have risen from
its depth of segregation which was beneath or within
the west coast lithosphere, but certainly not deeper
than 200 km because the first magmatic event was gabbroic.
The hiatus in igneous activity from 460 Ma
to 93 Ma along the West Coast rift indicates that
during this period the magma pressure in the chamber
was less than lithospheric, and/or the temperature
was too low for magma to cross the liquidus of its
compositions. By 93 Ma, that is 350 My later, this
problem was removed.
4.B The
beginning of the second phase of igneous activity,
at 93 Ma: This phase, along the West Coast rift,
is marked by a magmatic event which was not plutonic,
like previous ones, but rather volcanic. Unlike the
previous episode, it began with magma of felsic composition
– the rhyolites. It is relevant to note that
Madagascar-Indian plate breakup took place at around
93 Ma. This breakup also occurred along the western
continental margin of India. Obviously, such a megascale
rifting event will leave its signature in the form
of volcanic, not plutonic activity, which can explain
the simultaneous rhyolitic volcanism. The composition,
felsic instead of mafic, probably indicates that the
temperature was too low to melt mafic components in
the magma chamber beneath the West Coast region, but
elevated enough to produce the rhyolitic melts, i.e.,
it was around 1000°C.
4.C The
Deccan volcanism, at 64-65 Ma: The second event
of this phase is the Deccan volcanism which occurred
after a hiatus of 30 Ma. Also, after exactly the same
hiatus, and at the same time of 64-65 Ma, the Indian
plate experienced breakup from yet another partner
at Gondwanaland time – the Seychelles. This
probably indicates that the second stage of magmatism,
which began at 93 Ma, was controlled by the breakup
events between Gondwanaland microplates and the Indian
plate. Also, it shows that the breakup process was
a gradual and progressive phenomenon, starting with
Madagascar-India separation and, after another 30
My, Seychelles-India separation. Since this process
controlled West Coast magmatism, which tectonism is
expected to do, we deduce that the magmatism was also
progressive. Thus, we conclude that (i) the rhyolitic
volcanism at 93 Ma resulted from Madagascar-India
breakup, and (ii) that this breakup event was a continuous
process which led to Seychelles-India breakup after
another 30 My and to Deccan volcanism at the same
time. The progressive chemical trend of the volcanics,
i.e., from rhyolite to basalt, indicates
gradual progressive increase in temperature and/or
gradual progressive lowering of the liquidus in the
magma chamber as a result of gradual progressive rifting/breakup
of the Indian plate with Madagascar and the Seychelles
respectively.
The shift from plutonism
during first phase to volcanism during the
second phase perhaps indicates the presence of direct,
uninterrupted conduits from the magma chamber to the
surface of the continental crust during the second
phase. This is what is expected during extensive rifting
events such as Madagascar-India and Seychelles-India
separation which did not occur during the first phase.
The large volume
of the Deccan volcanics and the high rate
of volcanism during the Deccan episode indicate:
-
higher rate of adiabatic
decompression due to continental scale rifting,
-
consequently, higher
rate of melting of magma in the chamber,
-
further, continental
delamination of the western continental crust due
to elevation in temperature and decrease in viscosity
caused by the presence of a heat source in the form
of a magma chamber at its base, and
-
a direct plumbing
system between the melt and the surface, during
eruption.
Considering that the
West Coast and Narmada-Tapi rift zones were reactivated
at the time of Deccan volcanism and the Cambay and Kutch
rifts were also available as direct conduits for the
upward movement of melt, we infer that the presence
of these four rifts and geophysical evidence of lithosphere
thinning beneath the westen coast explains the size,
volume and eruption rate of the Deccan volcanics.
The geochemical
variation within the Deccan volcanics, as mentioned
above, perhaps indicates differences in the chemistry
of the host rocks. For example, along the Narmada-Tapi
rift zone the magmatic melt must have interacted with
the host sediments, which are Mahakoshal Jurassics
along with Archean metamorphics. Similarly along the
West Coast, Cambay and Kutch rifts, the melt would
have interacted with Archean-Precambrian metamorphics.
Consequential changes in the chemistry would be reflected
in the geochemistry of the DVP volcanics.
4.D
Post Deccan
4.D.1: 61 Ma:
The rifting which had started at 93 Ma resulted in
the opening of the Carlsberg ridge at about 61 Ma.
Deccan volcanism also continued, as suggested by the
age of DVP rocks from the Bombay area (Sheth &
Ray, 2002). This is the time when the Laccadive
ridge also experienced Deccan volcanism.
4.D.2: 55-50 Ma:
Sea-floor spreading along the Carlsberg ridge resulted
in the emplacement of ocean floor between the ridge
and the continental margin of the Indian West Coast.
Deccan volcanism along the West Coast rift produced
the Maldive ridge at around 55 Ma and the Chagos ridge
at around 50 Ma. Clearly, the Maldive and Chagos ridges
formed by interaction of the West Coast rift magma
with Carlsberg ridge mantle magma because the Laccadive
ridge, at 61 Ma, is characterised by melt with the
same chemistry as melt from the magma chamber beneath
the western continental crust of India.
The locations of these
ridges mark the position of India at a given point
in time. At this time, the Indian plate was moving
northwards at a velocity of 18-19 cm/year. Thus, the
position of Indian plate was controlled by (a) its
own velocity in a northerly direction, and (b) the
speed of Carlsberg ridge propagation.
4.D.3: 45-35 Ma:
By this time, the northward movement of India had
slowed considerably because the Indian plate had collided
with the Eurasian plate. As a result, intraplate tectonics
were solely responsible for deformational events.
Along the West Coast rift, two types of forces were
important (Figure 2), the north-south-trending West
Coast and Cambay rift forces and the east-west-trending
Narmada-Tapi rift force. The net vector force of these
two combined resulted in shearing and stretching along
the West Coast rift zone (Figure 2). Simultaneously,
isostatic balancing forces resulting from the emplacement
of a huge volume of volcanics resulted in vertical
movements along the west coast. These conclusions
are based on the fact that the West Coast rift is
bounded by intersecting sets of faults and fractures
which extend up to the Laccadive ridge (Figure 2).
The fracture system north of 16°N formed during
the late Cretaceous whereas the systems to the south
of 16°N formed during the middle to late Tertiary.
This indicates that deformation along the coast had
started in the late Cretaceous and gradually progressed
southwards during the late Tertiary. Probably, the
combined outcome of these forces resulted in Laccadive
ridge separation (due to stretching), southward displacement
(due to shearing) and subsidence (due to isostatic
balancing) during the late Tertiary.
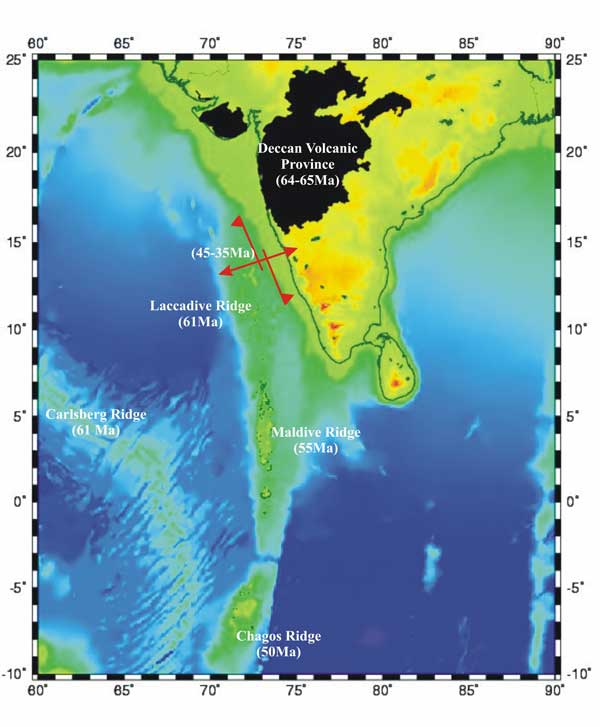
Figure 2: Diagrammatic representation
of the proposed hypothesis described herein for the
geological history of the Indian plate from 65 Ma
onwards. The major stress directions along western
continental margin of India 45-35 Ma are indicated
by red arrows.
Summary
We propose that
a magma chamber underlies the West Coast rift of the
Indian peninsular. In support of this idea, we point
to evidence for continuous magmatism along the west
coast at 678 – 460 Ma, which shows a continuous
trend of fractional melting. This was followed by
a lull in magmatism for almost 350 My. Magmatism started
again at around 93 Ma when Madagascar broke away from
the Indian plate. Since that rifting took place along
the west continental margin of India (i.e. today’s
western coast), we conclude that the associated magmatism
along the western continental margin of the Indian
plate was related to Madagascar-India break up. Rifting
led to decompression which in turn led to partial
melting in the magma chamber beneath the western continental
margin of India. This is supported by the fact that
magmatism during this phase started with felsic volcanism
and not mafic.
As rifting proceeded
along the western margin of the Indian plate, the
rate of partial melting in the magma chamber increased
proportionately leading to the mafic Deccan volcanism.
The high rate of Deccan volcanism was due to the fact
that along with the West Coast rift, three other deep
crustal rifts were activated simultaneously. This
could have been due to continuous rifting along the
west coast from 93 Ma onwards. We propose that the
high volume of Deccan volcanism was because:
-
The magma
chamber accumulated magma for almost 350 My, between
460 Ma and 93 Ma, during which time magma was added
to the chamber and crystallized, and
-
Delamination
of the Indian continental crust occurred above the
magma chamber.
We envisage the
magma chamber process to be as follows. A huge magma
chamber progressively accumulates melt, first from
the underlying mantle and eventually from both mantle
and continental crust (by delamination). The molten
material solidifies with time and remains in the chamber
during the period 460-93 Ma. When continental breakup
of greater India starts (including Madagascar rifting
away) the solified magma begins to melt. Since the
melting point of felsic components is lowest, these
are melted first and rhyolites typically comprise
the first phase of volcanics. Alkaline magma is not
initially formed because it has a higher melting point.
Following Deccan volcanism, the Carlsberg
ridge formed and the Indian plate continued to move
north. The systematic time progression of volcanism
between the Carlsberg ridge and the Indian plate is
due to sea floor spreading and not to plate
movement above hotspot.
When the Indian plate collided with
the Eurasian plate, its velocity decreased considerably
and intraplate forces started to play a lead role.
The main forces were then an EW force along the
Narmada-Tapi rift and a NS force along the West
Coast rift. The combined affect of these forces
led to rifting and shearing along the West Coast
rift and resulted in subsidence, pull-apart and
en echelon deformation along the Laccadive
ridge.
To reiterate, the proposition that
~ 106 km3 of Deccan basalts
was erupted from a magma chamber in which it was
stored is radical. However, there are several strong
points that support this suggestion:
-
The long history of magmatism
along the same zone which culminated in the
eruption of the Deccan Traps,
-
A lull in the magmatism
for 350 My and its subsequent reactivation,
-
DSS results which show
that the Moho upwarped beneath the west coast
during the late Cretaceous. This could be associated
with formation of the magma chamber,
-
Continental scale rifting
(Seychelles-India) and Deccan volcanism are
contemporaneous. The timing of the major phase
of Deccan volcanism is considered to be ~ 66-62
Ma (Courtillot et al., 1986; Venkatesan
et al., 1993) and the timing of Seychelles-India
breakup is also thought to be late Cretaceous
(Biswas, 1982). Note that the breakup
of Gondwanaland was a continuous process which
started (in the case of greater India) with
the separation of Madagascar (at ~ 93 Ma) and
by 63 Ma, Seychelles also separated from mainland
India (Biswas, 1982; Gombos et
al., 1995). The breakup of Seychelles-India
is thought to be the main rifting phase associated
with Deccan flood basalt eruption and the available
data suggest that they were contemporaneous.
This age data provides strong evidence in favor
of stored magma which underwent progressive
melting, in proportion to advancement in rifting
(and consequent lowering of liquidus of the
magma in the chamber), and resulted in volcanism
whose rate and volume corresponded to the rate
and scale of rifting.
-
The time of reactivation
of the deep crustal continental rifts coincided
with breakup events elsewhere in greater India,
-
The volcanism in the second
phase started out felsic and gradually became
mafic over a 30-My period, which points toward
progressive lowering of the liquidus, and not
a sudden increase in temperature, and
-
Geophysical evidence points
towards thinning of the continental crust north
of 15°N, that is exactly beneath the Deccan
volcanic province. This provides additional
support for the existence of delaminated continental
crust there.
We offer these ideas
for further work and discussion.
Acknowledgments
The authors appreciate the continuous
encouragement, valuable discussions and critical
comments of Dr. Harsh K. Gupta, Secretary, Department
of Ocean Development, Government of India and Dr.
P.C. Pandey, Director, NCAOR. The authors are thankful
to Prof. John J. Mahoney who very kindly provided
his critical comments which helped us to improve
the manuscript. We were inspired to work on the
Deccan by the thought-provoking arguments and iconoclastic
views of Dr. H. C. Sheth. We thank our colleagues
at NCAOR for their remarkable contribution all through
the brainstorming sessions and discussions of the
Deccan.
References
- Biswas,
S.K. 1982, Bull. Assoc. Petrol. Geol.,
66, 1497-1513.
- Biswas,
S.K. 1987, Tectonophysics, 135,
307-327.
- Chanda,
S.K., & Bhattacharya, A., 1966, J. Geol.
Soc. India, 7, 91-99.
- Chandrasekharam,
D. 1985, PEPI, 41, 186-198.
- Courtillot,
V., J. Besse, D. Vandamme, R. Montigny, J.J. Jaeger
& H. Cappetta 1986, EPSL, 80,
361-374.
- Devey,
C.W. & Stephens, W.E., 1991, J. Geol. Soc.
London, 148, 979-983.
- Glennie,
E.A., 1932, Surv. India Prof. Pap., 27,
10-16.8.
- Gombos,
A.M, Jr., W.G. Powell & I.O. Norton 1995,
Sedimentary Geology, 96,
119-129.
- Gupta,
H., Rastogi, B.K., Indra Mohan, Rao, C.V.R.K.,
Sarma, S.V.S. & Rao, R.U.M., 1998, Tectonophysics,
287, 299-318.
- Harbison,
R.N. & Bassinger, B.G., 1970, Geophysics,
35, 603-612.
- Kaila,
K.L., Roy Chowdhury, V., Reddy, P.R., Krishna,
V.G., Narain, H., Subbotin, S.I., Sollogub, V.B.,
Chekunov, A.V., Kharetchko, G.E., Lazarenko, M.A.
& Iichenko, T.V, 1979, J. Geol. Soc. India,
20, 307-333.
- Kaila,
K.L., Reddy, P.R, Dixit, M.M. & Lazarenko,
M.A., 1981, J. Geol. Soc. India, 22,
1-16.
- Mahoney,
J.J., 1988, in Macdougall, J.D., ed., Kluwer Academic
Publ., 151-194.
- Mishra,
D.C. 1977, Earth Planet. Sci. Lett., 36,
301-308.
- Sheth,
H. C. in Plates, Plumes & Paradigms (ed. Foulger,
G. R., J.H. Natland, D.C. Presnall and D.L. Anderson)
000-000 (Geological Society of America, this volume).
- Nair,
M.M. & Vidyadharan, K.T., 1982, J. Geol.
Soc. India, 23, 46-51.
- Owen,
H.G., 1976, Phil. Trans. Royal Soc. London,
281, 223-291.
- Quershy,
M.N., 1971, J. Geophys. Res., 76,
545-557.
- Reddy,
P.R, Venkatesvarlu, N., Rao, K. & Prasad,
A.S.S.S.R.S 1999, Current Sci., 77.
- Sheth,
H.C., 1999, Tectonophysics, 311,
1-29.
- Sheth,
H.C. & Ray, J.S., 2002, Int. Geol. Rev.,
44, 624-638.
- Soman,
K., Nair, N.G.K., Golubyev, V.N. & Arakelyan,
M.M., 1982, J. Geol. Soc. India, 23,
458-462.
- Soman,
K., Santosh, M. & Golubyev, V.N., 1983, Indian
J. Earth Sci., 10, 137-141.
- Valsangkar,
A.B., Radhakrishnamurthy, C., Subbarao, K.V. &
Beckinsale, R.D., 1981, Geol.Soc. India Mem.,
3, 265-276.
- Venkatesan,
T.R., Pande, K. & Gopalan, K. 1993, EPSL,
119, 181-189.
|
Discussion
Tuesday, 29th March, 2005: Hetu
Sheth
This article is very welcome, and I appreciate
that the authors are seeking alternate explanations
for the magnificent Deccan volcanic province of India.
I believe, however, that elements of the alternative
model proposed here are untenable. I offer the following
specific comments which I am sure will be taken constructively,
because we have the same goal. All references cited
here can be found in Sheth (2005).
1. The authors state that “[Sheth’s]
suggestion that the DVP and Laccadive-Chagos ridge
formed as a consequence of southward crack propagation
along the Vishnu fracture zone is not consistent with
data concerning the geomagmatic and tectonic history
of the Indian peninsular plate”. It is not clear
what they mean by this. If the Ar-Ar dates obtained
on the Laccadive-Chagos Ridge rocks are to be believed
(Baksi, 1999, 2005 gives reasons not to believe
them), then southward crack propagation in a northerly
moving plate, as proposed by Sheth (2005),
is a good alternative to a plate moving over a fixed
plume. If these dates should not be believed (a movement
I shall support), I am happier because then I don’t
have to explain the “track” by southward
crack propagation either. It is simply a leaky fracture
zone without evidence of systematic age progression,
like many others scattered worldwide.
2. The present volume of the Deccan is not 2 x 106
km3. This may have been the original volume.
3. The authors use 93 Ma as the valid age of the
St. Mary’s Islands felsic volcanics, related
to India-Madagascar breakup. However, this date, obtained
by Valsangkar et al. (1981), was only an
average of six widely differing K-Ar dates, and may
have no geological meaning as pointed out by Pande
et al. (2001). Torsvik et al. (2001,
Terra Nova) obtained direct U-Pb zircon ages of ~
90 Ma on these volcanics, and Pande et al.
(2001) got excellent crystallization ages of ~ 85
Ma on them by the Ar-Ar technique.
4. 93 Ma vs. 85 Ma is an important point. If the
former is correct, then the St. Mary’s Islands
rhyodacites are at the beginning of the Indo-Madagascar
province. If their age is 85 Ma, however, they are
at or near the end of the Indo-Madagascar province.
This has important implications for mantle and crustal
dynamics and sources, and of course the environmental
effects of such volcanic episodes. In the Deccan,
many/most felsic rocks appear to postdate the “flood
basalt” episode (Sheth et al., 2001;
Sheth & Ray, 2002), similar to the 85
Ma St. Mary’s Islands rhyodacites and Indo-Madagascar
flood basalts case.
5. The latest geophysical/seismological studies (Mohan
& Ravi Kumar, 2004) do not support the widespread
and long-held view (partly based on deep seismic soundings)
that the basement crust along the western Indian coast
is greatly thinned. Mohan & Ravi Kumar
(2004) find that this crust is of normal shield thickness
(36 – 40 km). Singh (1998) arrived
at a similar conclusion for the Navsari area based
on gravity modelling. Some sort of fracture/structural
boundary control through normal-thickness crust is
apparently at work.
6. Fractional melting is not what the authors think
it is. Fractional melting, as opposed to batch melting,
means that small melt fractions are removed from their
source rock as soon as they are formed, and do not
react with the residue. The production of rhyolites
from the partial melting of a basaltic source does
not mean the melting was fractional.
7. A frozen magma chamber that rested under the western
Indian coast for 300 Myr is a difficult idea to embrace.
Surely, the early magmatic events would have left
a depleted residue there, but such residues would
be difficult to melt. If a frozen magma chamber, do
the authors envisage it to be basaltic/gabbroic in
composition? Its partial melting then could not produce
the voluminous basaltic magmatism of the Indo-Madagascar
and Deccan provinces. The authors have overlooked
the enormous volumes of basalts in the former, which
outcrop mostly in Madagascar.
8. The partial melting of such a magma chamber could
have produced felsic volcanism; however, this usually
involves or is aided by hydrous melting. Where did
the water come from, at 90 Ma and 65 Ma? And how did
the 90-85 Ma Indo-Madagascar event leave sufficient
fertile source rock to be melted at 65 Ma?
9. What is the mechanism of continental crustal delamination
that the authors propose? What would have made it
denser than the underlying mantle to enable it to
sink?
10. An alkalic magma does not necessarily have a
higher temperature (not melting point) than a tholeiitic
one. Melting points, or rather solidi, are for source
rocks, not for magmas. Tholeiitic magmas are favoured
by higher-degree melting, shallower depths, and hydrous
melting. Primary alkalic magmas are favoured by lower-degree
melting, greater depths, and CO2 in the
mantle source. (ome magmas are also alkalic due to
contamination by lithosphere, or advanced fractional
crystallization. Indeed, at low values of lithospheric
extension and mantle decompression, the first magmas
to form are expected to be deep, low-melt-fraction,
volatile-rich alkalic, mafic magmas.
I hope these comments are useful.
Tuesday, 4th May, 2005: Anju Tiwary
In reply to Hetu’s comments:
1. We would be interested in more specifics regarding
Hetu's perception of the origin and evolution of the
Vishnu fracture zone.
2. The number we quote for the present volume of
the Deccan is been taken from published work, as mentioned
in our article. We use the number quoted in "Flood
basalts, mantle plumes and mass extinctions”
by Steve Self and Mike Rampino, and available on the
website http://www.geolsoc.org.uk/template.cfm?name=fbasalts
3 and 4. Our purpose in using the Valsangkar
et al. (1981) reference was to let the reader
know that the India-Madagascar separation is widely
accepted to be at ~ 90 Ma, as is evident from several
other articles (Storey et al. 1995; Plummer,
1995; Gombos et al., 1995; Raval &
Veeraswamy, 2003). It is beyond the scope of
this article to discuss the issue of “reliability
of ages” of rhyolites at St.Mary’s Island
or whether they are related to the timing of India-Madagascar
separation or not.
5: As far as crustal thickness is concerned, we are
looking from a holistic point of view concerning how
the crustal thickness varies northwards from the Indian
shield along the major west coast trends. There are
numerous published studies on this issue. A recent
seismological study carried out by Gupta et al.
(2003) shows northward “crustal thinning”
(with respect to the southern Indian shield) along
pre-existing trends. Taking most of the published
geophysical work in the region (DSS, Gravity, Magnetotellurics,
and Receiver Function Analysis) into account the northward
reduction in crustal thickness is very clear (Reddy
et al. 1999; Kaila et al., 1992; Kaila
& Krishna, 1992; Chandrasekharam,
1985; Gupta et al., 2003). Gupta et al.
(2003) presented the regional picture (between latitudes
20°N-80°N) based on the receiver-function
analysis whereas the work carried out by Mohan
& Ravi Kumar (2004) focuses mainly in the
central part of the Deccan Traps (between 20°N
and 18.3°N). Moreover, we must also keep in mind
that the non-uniqueness involved in the receiver-function
analyses before embarking upon any particular number.
In view of all this, one must consider the constraints
on the crustal thickness from multiple geophysical
techniques.
6: The definition of fractional melting and batch
melting is given here to avoid confusion. This definition
is taken from the book Igneous Petrogenesis
by Marjorie Wilson, page 59.
Equilibrium or Batch melting: the
partial melt formed continually reacts and equilibrates
with the crystalline residue until the moment of segregation.
Up to this point the bulk composition of the system
remains constant.
Fractional or Rayleigh melting: the
partial melt is continuously removed from the system
as soon as it is formed, so that no reaction with
the crystalline residue is possible. For this type
of partial melting the bulk composition of the system
is continuously changing.
Therefore, through batch melting it is not possible
to get rhyolitic melt from the source of basaltic
composition but fractional melting of basalt can produce
rhyolitic melt because the melt gets out of the system
instead of further reacting with it to reach equilibration.
7: Our article clearly states that due to the geological
history of intermittent magmatism, it is viable to
conjecture (a) magma chamber(s) beneath the west coast
fault. Since a magma chamber cannot remain completely
isolated from its surroundings, whatever the time
span of its dormant phase, it is not possible to deduce
the primary composition of magma chamber. The processes
of magma mixing, contamination and partial melting/fractional
crystallization would obviously overlap in time. Hence,
it is not possible to comment on the composition of
the magma chamber during its evolution between 460
Ma and 93 Ma. However, the primary melt would be EMORB,
that is erupted during the first phase of magmatism
at 670 Ma.
The question of the volume of the Deccan volcanic
province is not difficult to answer, if one takes
into consideration the complete geological evolution
of the Western Indian Peninsula. We would like to
know Hetu's views about the geochemistry of the continental
crust and lithosphere beneath west coast fault Does
he think that this fault is a palaeo-suture? If so,
then shouldn’t there be underplated and obducted
fossil oceanic crust? That would provide a source
for voluminous basalt.
8: First, we would like to correct the view that
partial melting of basalt has to be aided by hydrous
melt to produce felsic volcanism. The presence of
water decreases the melting point temperatures of
mafic minerals, so felsic volcanics are produced at
lower temperatures. If hydrous phases are not present,
then felsic volcanics are produced from basaltic material
at higher temperatures than in hydrous conditions.
Our reply is the same regarding fertile sources as
for huge basaltic volumes. The underplated and obducted
oceanic crust which occupied the western continental
fault lithosphere had to be of the Precambrian Era
and therefore enriched.
9: The presence of underplated oceanic crustal material
would make it denser and allow sinking.
10: It is incorrect to assume that tholeiitic or
alkaline magma characterizes conditions as mentioned
by Hetu. Tholeiitic and/or alkaline magmas are found
in virtually all kinds of tectonic settings and conditions
(Page 11, table 1.3 of Igneous Petrogenesis
by Marjorie Wilson).
Regarding melting points, Hetu is right that melting
points are for solid phases and but then we consider
that magma from 460 Ma and later fluxes in the chambers
were solidified by 93 Ma. So, we are indeed talking
about melting points.
We thank Hetu for his comments.
References:
Chandrasekharam, D. 1985; PEPI, 41,
186-198.
Gombos A.M. Jr., Powell, W.J., Norton, I.O. (1995);
Sedimentary Geology, 96,
119-129.
Gupta et al. (2003); Geophys. Res. Lett.,
30, 1419
Kaila & Krishna (1992); Curr. Sci., 62,
117-154
Kaila et al. (1992); Geophys. Jour. Int;
111, 45-66
Mohan and Ravi Kumar (2004); Geophys. Res. Lett.,
31, 1419
Plummer , Ph. S (1995) Journal of African Earth
Sciences, 20, 91-101.
Raval V. and Veeraswamy K., (2003); Journal of
Virtual Explorer; 12, 117-143.
Reddy et al. (1999); Current Sci., 77
Storey, M., Mahoney, J.J., Saunders, A.D., Duncan,
R.A., Kelly, S.P., and Coffin, M.F., (1995), Science,
267, 852-855.
|
last
updated 5th May, 2005 |
|
|