Laurent Gernigon1, Dieter Franke2, Laurent Geoffroy3, Christian Schiffer4, Gillian R. Foulger5 & Martyn Stoker6 1Geological Survey of Norway (NGU), Trondheim, Norway; Laurent.gernigon@ngu.no
2Bundesanstalt für Geowissenschaften und Rohstoffe (Federal Institute for Geosciences and Natural Resources), Hannover, Germany; Dieter.Franke@bgr.de
3Institut Universitaire Européen de la Mer (IUEM), Plouzané, France; Laurent.Geoffroy@univ-brest.fr
4Department of Earth Sciences, Uppsala University, Villavägen 16, 75236 Uppsala, Sweden; christian.schiffer@geo.uu.se
5Department of Earth Sciences, Durham University, Durham, UK, g.r.foulger@durham.ac.uk
6Australian School of Petroleum, University of Adelaide, Adelaide, SA 5005, Australia; martyn.stoker@gmail.com
This webpage summarizes part of Gernigon, L., Franke, D., Geoffroy, L., Schiffer, C., Foulger, G.R. & Stoker, M. 2019: Crustal fragmentation, magmatism, and the diachronous opening of the Norwegian-Greenland Sea. Earth-Science Reviews, doi: https://doi.org/10.1016/j.earscirev.2019.04.011.
Introduction
The opening of the Norwegian-Greenland Sea (NGS) represents the final stage of the progressive Pangean dislocation between North America, Greenland and Eurasia. This process involved a long history of rifting in the NE Atlantic region and a resulting complex mosaic of inherited basement terranes and structures (Figure 1). Ultimately, a polyphase system of continental rifts facilitated rupture of the lithosphere in the Early Cenozoic. A major rift-to-drift characteristic of the NGS is its widespread conjugate volcanic passive margins (VPMs) which are part of one of the world’s largest igneous provinces—the North Atlantic Igneous Province.
Despite wide consensus on these geophysical observations, an understanding of the mechanism of formation of VPMs and the onset of magmatic breakup in the NGS remains incomplete and debated (Geoffroy, 2005; Franke et al. 2019; Guan et al. 2019). In our recent paper, we summarised and discussed how the original continental amalgamation (Baltica-Greenland) ultimately broke apart and how and when the early spreading stage culminated in the formation of a new ocean surrounded by atypical continental fragments.
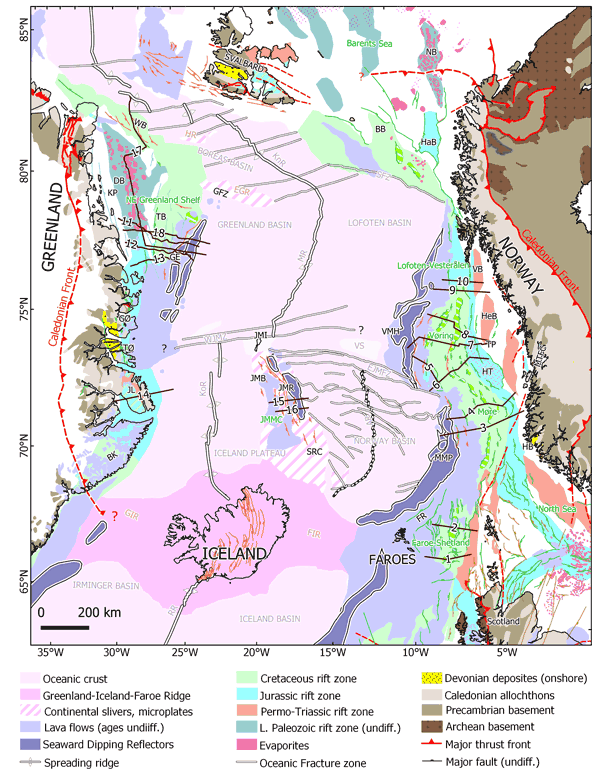
Figure 1: Regional tectonic map of the NGS and location of the main Paleozoic to Cenozoic rift zones and sedimentary basins. See Gernigon et al. (2019) for details and basin descriptions. Click here or on Figure for enlargement.
Pre-Tertiary extension of the Norwegian-Greenland Sea
In terms of timing and lithospheric configuration, breakup of the NGS was not the result of a continuum of lithospheric deformation (e.g., without episodes of thermal cooling) initiated in the Jurassic or earlier. Severe crustal and lithospheric deformation in the Late Jurassic-Early Creatcous predates the last rifting phase and breakup stage(s) in the NGS by more than 50–70 Myrs. Accordingly, older rifted sedimentary basins in the NGS were probably under thermal relaxation before the Late Cretaceous-Paleocene renewed phase of rifting preceding the formation of the VPMs (e.g., van Wijk & Cloetingh, 2002).
In the outer part of the mid-Norwegian margin, the Late Cretaceous-Paleocene rifting and VPM developed westwards of a paleo-necking zone in the outer flexure zone of large Cretaceous sag basins already affected by thermal cooling (Figure 2). A lithosphere resistant to subsequent reactivation offers an elegant explanation for why the different NGS rifting axes (e.g., Late Jurassic-Early Cretaceous and Late Cretaceous-Paleocene) migrated or jumped from the proximal margin to the central margin to the distal margin domain (Figure 2). Before breakup, the last event likely affected the thicker continental distal marginal plateau, which was only mildly affected by the mid-Mesozoic thinning event(s). Crustal thinning may have been insufficient to allow a large zone of exhumed and heavily serpentinised mantle material to form just underneath the Inner SDRs (See previous discussion in Gernigon et al. 2015; Theissen-Krah et al. 2017; Abdelmalak et al. 2017; Zastrozhnov et al. 2018; Millet et al. 2019).
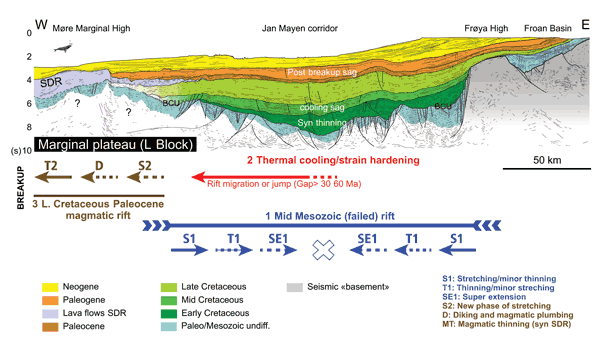
Figure 2: Regional section across the mid-Norwegian margin. Margin evolution was characterised by a first sequence of stretching (S1) and drastic thinning (T1) but the rift slow-down, or most likely failure, in the mid-Cretaceous before a second phase of rifting (S2, T2) initiated about 30–60 Myr later. After strain hardening of lithosphere, a new phase of stretching and thinning focused on the weaker western flank of a preserved marginal plateau before breakup (e.g., L-Block in the nomenclature of Guan et al. 2019). Click here or on Figure for enlargement.
Final weakening of the lithosphere by thinning and/or magmatism
Between NE Greenland and the Lofoten-N Vøring margins, Skogseid et al. (2000), Tsikalas et al. (2002) and Lundin & Doré (2018) showed that the seaward-dipping reflectors (SDRs) and the breakup axis trend oblique to the pre-existing Paleozoic/Mesozoic rift structures (Figure 3). This oblique breakup creates the final but "apparent" asymmetry of the margin but does not necessarily reflect the configuration of the pre-breakup rift system.
A predisposed set of dextral transforms and large-scale shear deformation (e.g., transcontinental shear zones) may explain why the line of breakup obliquely bisected the pre-existing Cretaceous rift system in the NGS (Lundin & Doré, 2018; Schiffer et al. 2019). Another possible explanation for the oblique and locally sharp breakup during the Early Eocene is magmatism. The most significant pre- and syn-breakup phases of magmatism were concentrated in the distal VPMs. Several modelling and field examples show that lithospheric softening associated with melting mantle instabilities at the base of the thermal lithosphere, crustal magma chambers and/or magmatic dyke intrusion can accommodate, localize, or relocalise the deformation at different lithospheric levels (e.g., Callot et al. 2002; Buck & Karner 2004; Yamasaki & Gernigon, 2009; Geoffroy et al. 2015).
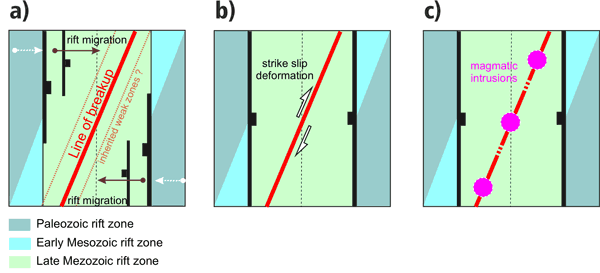
Figure 3: Different scenarios to explain the obliquity of the final line or breakup in the NGS. a) Progressive and asymmetric cooling and strain migration of the rift system. b) Strike-slip deformation of the lithospheric plate. c) Magmatic plumbing of the lithosphere. All these processes could interact.
Diachronous and segmented emplacement of SDRs
The main characteristics of VPMs are thick volcanic wedges of SDRs emplaced along the proto-breakup axes (Eldholm et al. 2000) (Figures 4 and 5). On the basis of geophysical data, a standardised set of descriptive terms and nomenclature has been established to classify the seismic ‘volcanostratigraphy’ (e.g., Inner Flows, Landwards Flows; Inner SDRs, Outer High and Outer SDRs) in the NGS (Planke et al. 2000; Berndt et al. 2001). The conjugate SDRs observed between Norway and Greenland have been interpreted as being emplaced over (highly) distended continental crust (Hinz, 1987) or oceanic crust (Mutter et al. 1984). Scientific drilling has provided evidence that, where sampled in the NE Atlantic, Inner SDRs magmas often incorporate material from the continental lithosphere (Meyer et al. 2007). As the Inner SDRs were earlier envisaged, the crust beneath Outer SDRs may be dominantly mafic. However, in general, its origin (continental or oceanic) is uncertain and poorly constrained.
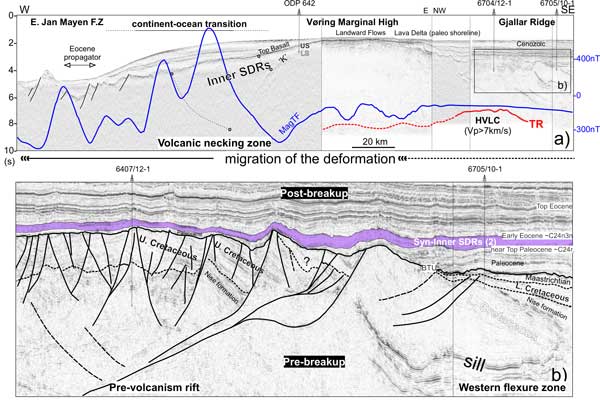
Figure 4: Example of seismic line (in two-way travel-time) from the outer Vøring Basin to the Vøring Marginal High (locations shown in Figure 1). The line (a) traverses structures of the oceanic domain in the west, a major SDR complex in the centre and pre-, syn-, and post-rift successions in the east. In the eastern portion of the line (see enlarged section (b) in the lower panel), the upper Cretaceous faulted blocks illustrate Late Cretaceous-Paleocene extension preceding the onset of major volcanism. A thin onlapping Early Eocene sequence (in purple) along this section coincides in time with the period of drastic magmatism including Inner SDR formation and thinning observed further west and constrained by ODP well 642. That well also drilled the transition (Horizon "K") between the Upper and Lower Series volcanics (US and LS) of the Vøring volcanic plateau. The purple curve in the upper panel represents the total magnetic field (MagTF) along the profile. Click here or on Figure for enlargement.
The SDRs acquire remnant magnetisation during emplacement of the lavas, thereby providing timing information. The entire Upper Series drilled and analysed on the Vøring Marginal High (ODP Hole 642E) preserves a dominant reversed magnetic polarity within reverse magnetic polarity Chron C24r (~57.1 to ~53.9 Ma) (Schönharting & Abrahamsen, 1989) (Figure 4). The low amplitude of the magnetic total field associated with the Landward Flows around and landward of ODP well 642 is most probably the result of negative magnetisation during the reverse C24r magnetic polarity. In contrast, the main Inner SDR wedge further west in the Vøring Marginal High is characterised by a prominent positive magnetic signature (Figure 4). To explain this positive signature associated with the Inner SDRs, normal polarity and remanent magnetisation are required for the uppermost and outer part of the Inner SDRs not sampled by the ODP well. As the underlying lava flows are correlated with negative polarity chron C24r, and because the flexure of the SDRs is most likely the consequence of a rapid development (< 2–3 Ma), the positive magnetic anomaly associated with the Inner SDRs is here regarded as the result of late lava flows emplaced during a period of positive magnetic polarity including C24n3n (53.9-53.4 Ma), C24n2n (53.2-53.1) and/or C24n1n (53.0-52.6 Ma) (Figure 6).
In contrast to the Vøring Marginal High, the Inner SDRs in northwestern Møre Marginal High have a negative magnetic signature. They formed landward of, and before, the first positive magnetic polarity chrons C24n1n and C24n3n and negative polarity chron C24r recorded in the oceanic domain. The latter are interpreted and mapped at the eastern edge of the oceanic Norway Basin, courtesy of high-resolution aeromagntic data (e.g., Gernigon et al. 2012, 2015). This spatial configuration suggests that the Inner SDRs in the northern part of the Møre Marginal High were already emplaced during the Thanetian (late Paleocene), before chron C24r and therefore prior to the emplacement of the adjacent Inner SDRs in the Vøring margin segment. In the Vøring Marginal High, the Inner SDRs are principally post-C24r and initiated at least 1–2 my later, in the Ypresian (early Eocene). This indicates that the emplacement of the Inner SDRs and breakup were likely diachronous in the NGS.
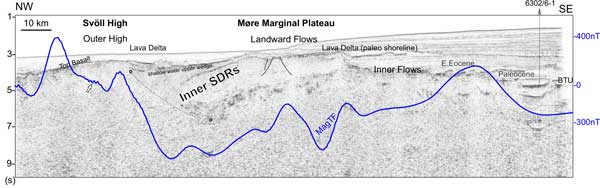
Figure 5: Seismic section across the Møre volcanic margin. This section illustrates volcanostratigraphic sequences emplaced during the onset of breakup. The purple curve represents the total magnetic field (MagTF) along the profile. Click here or on Figure for enlargement.
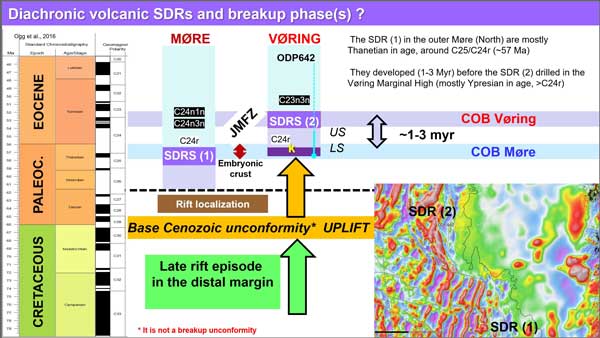
Figure 6: Tectonomagmatic sequence of the onset of breakup inferred from the geophysical data.
Discussion: Compartmental "unzipping" of the volcanic margins and propagation of seafloor spreading
VPM formation and breakup in the NGS are typically regarded as processes that occurred almost instantaneously across all NGS segments around 54 Ma (~C24r) (Skogseid et al. 2000; Gaina et al. 2009). However, assuming rigid plates and an homogeneous lithosphere, an oceanic rift should, in theory, propagate over time through the extended lithosphere as a natural consequence of the relative motion of the plates about a pole of rotation. It is unlikely that instantaneous breakup occurred of long (>200–400 km) marginal sections or that the progressive opening of the oceanic basin occurred around one common pole of rotation in the NGS. Due to the complex geological history of the NGS, it is also improbable that the pre-breakup lithosphere was homogenous. Therefore, VPM development was likely a step-by-step, diachronous process.
Gernigon et al. (2019) and Franke et al. (2019) showed that the oldest oceanic cells nucleated separately first in the northern part of the outer Møre margin and west of the Lofoten-Vesterålen margin, close to the Greenland Fracture Zone (Figure 7). The individual nascent oceanic sub-basins underwent progressive development, e.g., in the Norway Basin. SDRs and early spreading developed progressively towards the Faroe Plateau/Greenland-Iceland-Faroe Ridge (GIFR) and the Vøring Marginal High where time delays in the breakup are indicated.
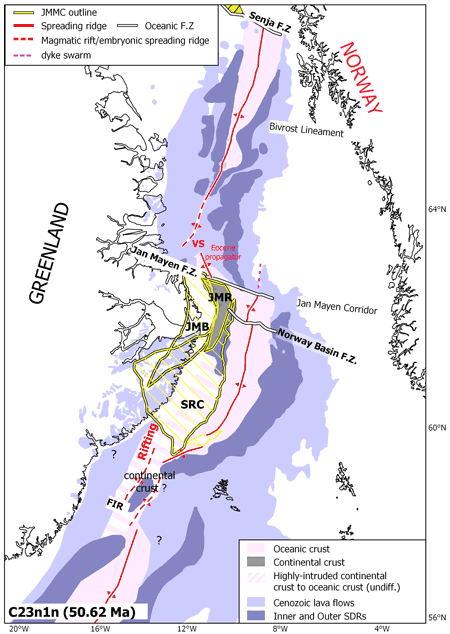
Figure 7: Early Eocene plate reconstruction of the NGS at C23n1n (50.6 Ma), when seafloor spreading is almost fully established north of the GIFR. The figure illustrates propagating and segmented oceanic segments, assuming that the crust underlying the Inner and Outer SDRs is not fully oceanic. There are typically major overlaps between the present-day outline of the Jan Mayen Microplate Complex elements (e.g., the Jan Mayen Ridge (JMR), the Jan Mayen Basin (JMB), and the Southern Ridge Complex (SRC)) and Greenland. This may be explained partly by significant Paleogene extension that affected the JMMC and the corresponding conjugate areas.
It appears that oceanic spreading centres preferentially grew within segments of heterogeneous and stretched continental lithosphere with or without a direct correlation with the propagation direction derived from global plate motion. In the NGS, the Vøring Marginal High, Faroe Plateau and, to some extent, the GIFR may simply have comprised (weak or strong) lithospheric barriers to volcanic rift propagation that hindered lateral growth of the volcanic margins and/or the narrow localisation of nascent oceanic rifts (Figure 8). The presence of such buffers may have favoured the complex rift-ridge overlapping that led to the breaking-off the JMMC. Here, the dislocation is interpreted to have been progressive (i.e. a rift-overlap type of microplate) and not necessarily the result of sudden rift relocation driven by plume interaction. The observed relatively magma-poor evolution of the western Jan Mayen margin would also conflict with a major thermal instability as origin. If a rift- and ridge-overlapping system controlled JMMC formation, a consequence is the preservation of continental material along the Faroe-Iceland-Ridge (e.g., Gernigon et al. 2012, 2015; Ellis & Stoker, 2014). Recent work suggests that a deep inherited Caledonian slab along the trend of the GIFR may have guided and controlled the rift overlap, magmatism, and dislocation of the JMMC (Schiffer et al. 2019). The origin and crustal composition of the GIFR continue to pose major unsolved questions that present research challenges for the future (Foulger et al. 2019).
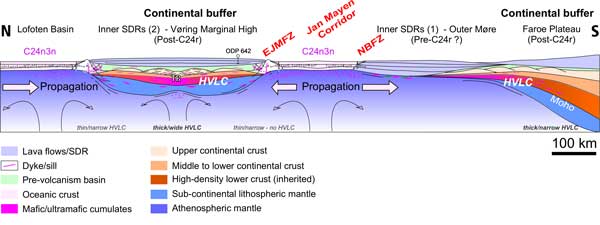
Figure 8: Conceptual model of volcanic margin segmentation during the punctiform initiation of breakup around C24n3n (Early Ypresian ~53.98 Ma). The model considers the volcanic margins to be diachronic. In this model, the breakup and early opening of the NGS were not instantaneous but propagated in different directions. Click here or on Figure for enlargement.
Conclusions
We draw the main following conclusions:
- Rifting and breakup in the NGS occurred in heterogeneous continental lithospheric assemblages.
- Palaeozoic to Cenozoic phases of rifting with intermediate cooling events preconditioned the NGS lithosphere prior to propagation of breakup. The final Late Cretaceous–Paleocene rift is commonly offset from the dominant mid-Mesozoic or older thinning axis of the NGS rifted margins. Strain hardening due to Mesozoic lithospheric cooling may explain the migration of the rift axes over time and may have partly influenced the final breakup configuration.
- Continental breakup in the NGS was highly magmatic, with large-scale volcanism (e.g., the SDRs) during VPM formation. VPMs developed preferentially either on thick continental ribbons (e.g., the Faroe Block) or moderately thinned continental crust (e.g., the Møre Marginal Plateau). The presence of a large zone of exhumed continental mantle before breakup and beneath the subaerial SDRs is unlikely. This is in marked contrast to magma-poor margins where extensive subcontinental mantle exhumation occurs in deep water prior to igneous crustal accretion.
- The final NGS line of breakup may have been controlled by highly oblique extension and associated plate shearing and/or melt intrusions and dyking before and during Inner SDR formation.
- Breakup of the NGS was neither instantaneous nor continuous across the entire region. Spreading ridges propagate initially towards local Euler poles, die out, migrate laterally, jump, change their propagating direction or eventually bifurcate. Spreading in our preferred model initiated in isolated cells in the northern Greenland Sea and the northern Norway Basin. The nascent "segmented ocean" grew step-by-step with a delay of 1–2 Myrs between emplacement of SDRs at the Møre and Vøring volcanic margins. Initial formation of oceanic crust nucleated west of the Lofoten and north of the Norway Basin in the Greenland Sea and propagated south towards the Jan Mayen Fracture Zone and the GIFR, respectively.
- We conclude that the transition from magmatic rift segments to mature seafloor spreading was a gradual process in the NGS. The early spreading system involved initial propagating segments separated by continental buffers and/or lithospheric barriers in different parts of the NGS (e.g., the Vøring Marginal High, the Faroe Plateau and part of the GIFR). The presence of such rheological heterogenities may explain the punctuated style of breakup and may have controlled the rift/drift overlap apparently required along the GIFR to explain the JMMC formation.
References
- Abdelmalak, M.M., Faleide, J.I., Planke, S., Gernigon, L., Zastrozhnov, D., Shephard, G.E. and Myklebust, R., 2017. The T-Reflection and the Deep Crustal Structure of the Vøring Margin, Offshore mid-Norway. Tectonics, 36(11): 2497-2523.
- Berndt, C., Mjelde, R., Planke, S., Shimamura, H. and Faleide, J.I., 2001b. Controls on the tectono-magmatic evolution of a volcanic transform margin: the Vøring Transform Margin, NE Atlantic. Marine Geophysical Researches, 22(3): 133-152.
- Buck, W.R. and Karner, G., 2004. Consequences of asthenospheric variability on continental rifting. In: G.D. Karner, B. Taylor, N.W. Driscoll and D.L. Kohlstedt (Editors), Rheology and deformation of the lithosphere at continental margins. Columbia University Press, New York, pp. 1-30.
- Callot, J.P., Geoffroy, L. and Brun, J.P., 2002. Development of volcanic passive margins: Three-dimensional laboratory models. Tectonics, 21(6): https://doi.org/10.1029/2001tc901019. Artn 1052.
- Eldholm, O., Gladczenko, T.P., Skogseid, J. and Planke, S., 2000. Atlantic volcanic margins; a comparative study.; Dynamics of the Norwegian margin. Geological Society Special Publications, 167: 411-428.
- Ellis, D. and Stoker, M.S., 2014. The Faroe–Shetland Basin: a regional perspective from the Paleocene to the present day and its relationship to the opening of the North Atlantic Ocean In: S.J.C. Cannon and D. Ellis (Editors), Hydrocarbon Exploration to Exploitation West of Shetlands The Geological Society of London, London.
- Foulger, G.R., Doré, T., Emeleus, C.H., Franke, D., Geoffroy, L., Gernigon, L., Hey, R., Holdsworth, R.E., Hole, M., Höskuldsson, Á., Julian, B., Kusznir, N., Martinez, F., McCaffrey, K.J.W., Natland, J.H., Peace, A., Petersen, K., Schiffer, C., Stephenson, R. and Stoker, M., 2019. The Iceland Microcontinent and a continental Greenland-Iceland-Faroe Ridge. Earth-Science Reviews: 102926. In press.
- Franke, D., Klitzke, P., Barckhausen, U., Berglar, K., Berndt, C., Damm, V., Dannowski, A., Ehrhardt, A., Engels, M., Funck, T., Geissler, W., Schnabel, M., Thorwart, M. and Trinhammer, P., 2019. Polyphase Magmatism During the Formation of the Northern East Greenland Continental Margin. Tectonics, 38(8): 2961-2982.
- Gaina, C., Gernigon, L. and Ball, P., 2009. Palaeocene-Recent plate boundaries in the NE Atlantic and the formation of the Jan Mayen microcontinent. Journal of the Geological Society, 166: 601-616.
- Geoffroy, L., 2005. Volcanic passive margins. Comptes Rendus Geoscience, 337(16): 1395-1408.
- Geoffroy, L., Burov, E. and Werner, P., 2015. Volcanic passive margins: another way to break up continents. Scientific Reports, 5: 14828.
- Gernigon, L., Blischke, A., Nasuti, A. and Sand, M., 2015. Conjugate volcanic rifted margins, seafloor spreading, and microcontinent: Insights from new high-resolution aeromagnetic surveys in the Norway Basin. Tectonics, 34(5): 907-933.
- Gernigon, L., Franke, D., Geoffroy, L., Schiffer, C., Foulger, G.R. and Stoker, M., 2019. Crustal fragmentation, magmatism, and the diachronous opening of the Norwegian-Greenland Sea. Earth-Science Reviews.
- Gernigon, L., Gaina, C., Olesen, O., Ball, P., Péron-Pinvidic, G. and Yamasaki, T., 2012. The Norway Basin revisited: From continental breakup to spreading ridge extinction. Marine and Petroleum Geology, 35(1): 1-19.
- Guan, H.X., Geoffroy, L., Gernigon, L., Chauvet, F., Grigne, C. and Werner, P., 2019. Magmatic ocean-continent transitions. Marine and Petroleum Geology, 104: 438-450.
- Hinz, K., Mutter, J.C., Zehnder, C.M. and Group, G.T.S., 1987. Symmetric conjugation of continent-ocean boundary structures along the Norwegian and East Greenland margins. Marine and Petroleum Geology, 4: 166-187.
- Lundin, E. and Doré, A., 2018. Non-Wilsonian break-up predisposed by transforms: examples from the North Atlantic and Arctic. Geological Society, London, Special Publications, 470: SP470. 6.
- Meyer, R., Van Wijk, J. and Gernigon, L., 2007. The North Atlantic Igneous Province: A review of models for its formation. Geological Society of America Special Papers, 430: 525-552.
- Millett, J.M., Manton, B.M., Zastrozhnov, D., Planke, S., Maharjan, D., Bellwald, B., Gernigon, L., Faleide, J.I., Jolley, D., Walker, F., Abdelmalak, M.M., Jerram, D.A., Myklebust, R., Kjølhamar, B.E., Halliday, J. and Birch-Hawkins, A., 2019. Basin Structure and Prospectivity of the NE Atlantic Volcanic Rifted Margin: Cross-border examples from the Faroe-Shetland, Møre and Southern Vøring Basins. Geological Society, London, Special Publications, 495: SP495-2019-12.
- Mutter, J.C., Talwani, M. and Stoffa, P.L., 1984. Evidence for a thick oceanic crust adjacent to the Norwegian margin. Journal of Geophysical Research, 89: 483-502.
- Planke, S., Symonds, P.A., Alvestad, E. and Skogseid, J., 2000. Seismic volcanostratigraphy of large-volume basaltic extrusive complexes on rifted margins. Journal of Geophysical Research-Solid Earth, 105(B8): 19335-19351.
- Schiffer, C., Doré, A.G., Foulger, G.R., Franke, D., Geoffroy, L., Gernigon, L., Holdsworth, B., Kusznir, N., Lundin, E. and McCaffrey, K., 2019. Structural inheritance in the North Atlantic. Earth-Science Reviews, in press.
- Schönharting, G. and Abrahamsen, N., 1989. Paleomagnetism of the volcanic sequence in Hole 642E, ODP Leg 104, Vøring Basin Plateau, and correlation with Early Tertiary basalts in the North Atlantic. In: O. Eldholm, J. Thiede and E. Taylor (Editors), Proceedings of the Ocean Drilling Program, Scientific Results, 104, pp. 912-920.
- Skogseid, J., Planke, S., Faleide, J.I., Pedersen, T., Eldholm, O. and Neverdal, F., 2000. NE Atlantic continental rifting and volcanic margin formation. In: A. Nøttvedt (Editor), Dynamics of the Norwegian margin. Geological Society Special Publications, pp. 295-326.
- Tsikalas, F., Faleide, J.I., Eldholm, O. and Blaich, O.A., 2012. The NE Atlantic conjugate margins. In: D.G. Roberts and A.W. Bally (Editors), Phanerozoic Passive Margins, Cratonic Basins and Global Tectonic Maps, pp. 141-201.
- van Wijk, J.W. and Cloetingh, S.A.P.L., 2002. Basin migration caused by slow lithospheric extension. Earth and Planetary Science Letters, 198(3-4): 275-288.
- Yamasaki, T. and Gernigon, L., 2009. Styles of lithospheric extension controlled by underplated mafic bodies. Tectonophysics, 468(1-4): 169-184.
- Zastrozhnov, D., Gernigon, L., Gogin, I., Abdelmalak, M.M., Planke, S., Faleide, J.I., Eide, S. and Myklebust, R., 2018. Cretaceous-Paleocene Evolution and Crustal Structure of the Northern Vøring Margin (Offshore Mid-Norway): Results from Integrated Geological and Geophysical Study. Tectonics, 37(2): 497-528.
last updated 31st
January, 2020 |