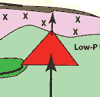 |
West
Greenland Glass in Picrite – Low-pressure
Cotectic Differentiates or Independent Compositions? |
James
H. Natland
RSMAS/MGG University of Miami,
Miami, FL 33149
Click here to
download a PDF version of this webpage
Introduction
I reply to “West
Greenland was hot–how else can you make 22,000 km3 of
picrites?” Larsen & Pedersen (2009).
The data set of Larsen & Pedersen (2000) is superb,
and more closely bounds the problem of temperature than any other
I have seen. I have enjoyed working with it. I welcome their comments
on Natland (2008).
Title
The title of Larsen & Pedersen (2009) speaks
to the tug that picrites have on the petrological conscience, extant
at least since O’Hara (1968), but perhaps even before
(Drever & Johnston, 1957), namely that all picrites
represent great heat and high temperature. This idea today is rather
entrenched. However, Clague et al. (1995) proposed that
most picritic basalt from Kilauea volcano and offshore Puna Ridge
resulted from mixing between crystal-rich sludges with abundant accumulated
olivine and strongly differentiated liquids that had evolved by crystallization
of plagioclase, clinopyroxene and olivine along a low-pressure cotectic.
The picrites, then, erupted at temperatures of host liquids (glasses),
far along a multi-phase liquid line of descent, and Clague et
al. (1995) calculated these temperatures using geothermometry.
I advocate this mechanism for Baffin Bay and West Greenland for exactly
the same reasons.The disposition of melt and magma chambers was considered
by Clague et al. (1995) and it is no different, no less
complex a problem, than it is now; any solution to it must be contrived.
Mountains of picrite notwithstanding, the problem
of the temperature of onset of crystallization of olivine phenocrysts
requires precise consideration of the compositions of glass and minerals.
What if deduced temperatures turn out to be low in all of them? There
is plenty of low-temperature picrite at Hawaii, and abundant dunite
xenoliths represent crystallization of a large volume of olivine-saturated
liquids near the bases of volcanoes (Clague & Denlinger,
1994). The olivine in these crystallized at <1200°C, although
olivine crystallized at higher temperature may now be re-equilibrated. Clague
et al. (1995) was a serious warning shot across the bow of traditional
interpretations. I have simply applied their approach to Baffin Bay
and West Greenland.
Associated Silicic Rocks
Noting the presence of interbedded silicic tuffs
and lavas was primarily a caution based on a prior interpretation
of Iceland (Natland, 2007), except that for Baffin Bay and
West Greenland, a geochemical signal of silicic contamination is
more likely. The setting is intra-cratonic rather than intra-oceanic.
If the Vaigat formation has no interbedded silicic lavas, but the
overlying Maligât formation does, then the possibility remains
that even lavas of the Vaigat formation might have experienced a
small amount of assimilation of silicic continental crust, or mixing
with rhyolitic partial melts derived from that crust. The situation
recalls that of Theistareykir Volcano in Iceland, which was selected
by Maclennan et al. (2003) for study partly because of absence
of interbedded silicic lavas. Theistareykir nevertheless carries
a small signal of silicic contamination, which I modeled as basalt-rhyolite
mixing (Natland, 2007). The presence of a small silicic
contaminant in lava of the Vaigat formation is, however, not relevant
to the question of temperatures.
ΔNb
Low ΔNb (Fitton et al., 1997) in West
Greenland basalt is a surprise, but again this has nothing to do
with temperature. My comment was directed mainly to the many who
prefer consistency of geochemistry in their geodynamic modeling,
and who argue that high ΔNb necessarily supports presence of
an enriched mantle source. Iceland is the standard of comparison,
the type locality, if you will, of high-ΔNb. The surprise was
discovering that the “plume-head” picrites of West Greenland,
representing the onset of the North Atlantic Igneous Province in
the plume hypothesis, and Iceland itself, the “plume tail”,
are different.
Whereas Fitton et al. (1997) and more recently Starkey
et al. (2009) see shifts in ΔNb as indicative of mantle
source properties in this region, I believe instead that it is mainly an
indicator of silicic contamination (Natland, 2007). That
is, assimilation of silicic material or basalt-rhyolite mixing
can explain interbedded basalts like these that have both positive
and negative ΔNb (Natland, 2007). Without assimilation
of, or mixing with, rhyolite I argued that Icelandic basalt, all
of which Fitton et al. (1997) define as having ΔNb > 0,
would more closely resemble mid-ocean-ridge basalt (MORB;
ΔNb < 0). Accepting that an underlying geochemical continuity
should exist across the North Atlantic Igneous Province, and noting
that assimilation and mixing are both well documented in the field
and by geochemistry, West Greenland supports my point. ΔNb,
then, is non-diagnostic or, more bluntly, useless as a source discriminant
especially in continental rift settings. I explained correlations
of ΔNb
with, e.g.,
light-rare-earth enrichment ([La/Sm]N) and isotopes of
Sr and Nd at Iceland by the same mechanism (Natland, 2007).
Crystallization along a Low-Pressure Cotectic
The fact remains that no basalt glass from Baffin
Bay and West Greenland (nor from Iceland) so far analyzed exhibits
olivine control. This has to be a serious drawback for backtrack
calculations, undercutting its cardinal assumption. As first emphasized
by Wright & Fiske (1971) for Kilauea, olivine control
is only demonstrated when its consequences are evident on two-oxide
variation diagrams for ALL oxides determined by chemical analysis
of major elements. Failure of demonstration on any single diagram,
for example TiO2-MgO, precludes it. Natland (2008) provided
more than one variation diagram showing lack of olivine control among
basaltic glass margins to picrites of Baffin Bay and West Greenland.
A single diagram might coincidentally have data points aligned along
some postulated olivine-control line, but other diagrams could, and
in this case do, rule it out.
An important step in evaluation of variation diagrams
is to show single-mineral or multiple-mineral control of crystallization
differentiation; then the rocks belong to the same liquid line of
descent and have a common parent. However, a sequence of basalt,
perhaps sampled over a short time from eruptions of the same volcano
(e.g., Kilauea) usually shows subtle parental variations.
These will produce some dispersion on variation diagrams, and different
places may contrast sharply (e.g., Wright, 1971),
although general underlying shallow differentiation control can usually
be seen. Glass from picrites of Padloping Island, Baffin Bay, and
West Greenland (BWG glass) illustrates this nicely.
BWG glass exhibits MORB-like cotectic variation
and shows no sign of olivine control. For example, both TiO2 and
total iron as FeOT increase as MgO decreases from 9.2 to 6.7%; in
the same course of differentiation, both CaO and Al2O3 decrease.
These variations cannot be accomplished simultaneously without plagioclase
on the liquidus. This was sufficiently shown on the diagrams in Natland (2008). Olivine
control has only been demonstrated in extrusive glasses from Hawaii’s
Kilauea volcano and Puna Ridge (Clague et al.., 1995); variation
diagrams for those glasses have a sharp inflection where olivine-controlled
differentiation joins the low-pressure cotectic at about 7% MgO content
(Figure 1A). Figure 1B shows that BWG glasses have no such inflection,
and that their overall trend is similar to trends seen in Indian
Ocean MORB (Figure 1C). Although Indian MORB have fairly wide CaO
variation at given MgO, they can be broken down into three types
based on differences in parental Na2O (Na8 =
Na2O at 8% MgO content; Klein & Langmuir,
1987), which have different characteristic phenocryst assemblages
in glass with highest MgO contents (Natland, 1991); viz:
- Type 1 = plagioclase, clinopyroxene and olivine;
- Type 2 = plagioclase and olivine;
- Type 3 = olivine alone.
All three types carry Cr-spinel.All three have trends
in Figure 1C requiring cotectic crystallization of mineral
assemblages dominated by plagioclase, in order for CaO to decrease
as MgO decreases, consistent with the phenocryst assemblages. From
Figure 1B, BWG glasses most resemble Indian Ocean Type 1 MORB, overlapping
Type 2. Note especially that glass-bearing basalt from DSDP Sites
212 and 236, labeled in Figure 1B, carries abundant plagioclase
and clinopyroxene phenocrysts (Natland, 1991).
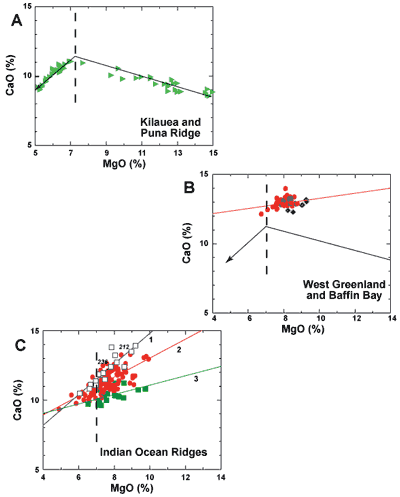
Figure 1: MgO versus CaO for basaltic
glass from A. Kilauea and Puna Ridge (Clague et al.., 1995);
B. West Greenland (Pedersen & Larsen, 2000) and Padloping
Island, Baffin Bay (Francis, 1985; Robillard et al., 1992);
and C. Central and Southwest Indian Ridges, and the Red
Sea (Lamont-Doherty Petrology Data Base, PetDb). Note
slightly different X-axis coordinates in A. In
A, the bold line with an arrow is hand-drawn to show olivine
and cotectic crystallization, with a break in slope at
7% MgO (vertical dashed line, repeated in B and C).In B,
blue West Greenland glasses were used by Larsen & Pedersen
(2000) for their backtrack calculations; red are all other
glasses. Padloping Island glasses are dark blue diamonds.The
red line is a linear regression through all data. In C,
open squares are Indian Ocean Type 1 MORB; red dots = Type
2; green squares = Type 3. Lines are linear regressions
through each type. |
More generally, no MORB in the Indian Ocean is picritic,
even if the glass has MgO > 9% and Mg# (= 100* Mg/[Mg+Fe2+]) > 70.
The three types have a general tectonic association: Type 1 occur
mainly in what might be called early rift settings such as the Red
Sea and locations near the Seychelles continental fragment, Type
2 are widely distributed along the modern Carlsberg and Central Indian
Ridges, and Type 3 occurs mainly along the Southwest Indian Ridge
near the Indian Ocean triple junction where spreading rates are very
slow. BWG glass, then, most strongly resembles early-rift Indian-Ocean
MORB, but verging on more typical Indian MORB compositions.
Most picrites also have negative Eu anomalies (West
Greenland -0.53 ± 0.26 – 14 samples; Padloping Island
-0.34 ± 0.27 – 36 samples; Starkey et al.,
2009). This can only happen with fractionation of plagioclase, as
in the MORB suite. Assuming the anomalies indicate matrix proportions
of rare-earth elements, similar to glass, and comparing to MORB with
similar Eu anomalies, the rock groundmasses have ~7-8% MgO contents,
like those of quenched BWG glass. Plagioclase must have been
on their liquidus, consistent with reductions in CaO and Al2O3 as
MgO content decreased along a cotectic, and prior to incorporation
of olivine phenocrysts.
Since BWG glass follows a low-pressure multi-phase
liquid line of descent similar to MORB, we can calculate the usual
parental parameters for MORB (Klein & Langmuir, 1987).
Figure 2 shows how extremely similar they are to MORB when comparing
Si8, Ti8 and Fe8 to Na8.
BWG glass partially or largely overlaps Types 1 and 2 Indian Ocean
MORB on all diagrams, especially glass from Padloping Island. West
Greenland glass has generally lower Si8 and somewhat higher
Ti8 than the most nearly comparable Indian MORB. In these
respects, BWG glass is also very similar to basalt glass from Iceland,
with West Greenland glass predominantly corresponding to higher-TiO2 Iceland
basalt (defined as having Ti8 > 1.4%; Natland,
2007), and most glass from Padloping Island resembling low-TiO2 Iceland
basalt. Picritic or not, Baffin Bay and West Greenland basalt glass
is no different from that of moderately differentiated Icelandic
basalt, and neither is very different from early-rift Indian Ocean
MORB.
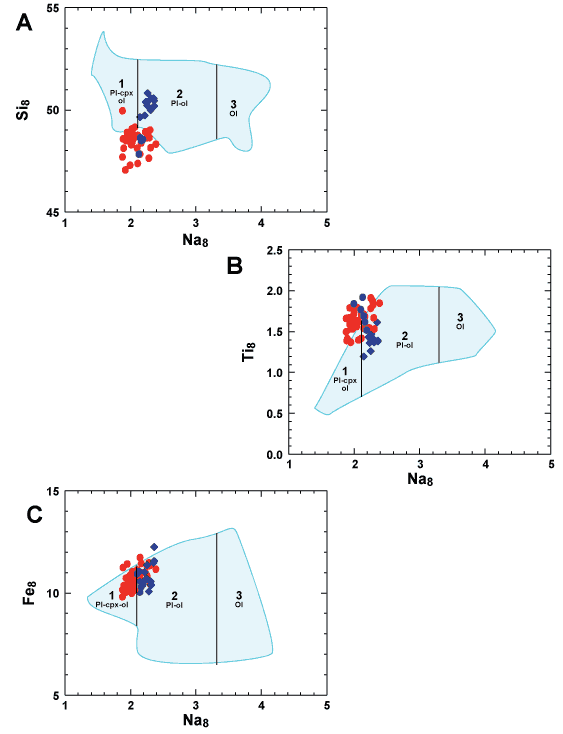
Figure 2: Parental attributes
of glass from West Greenland and Padloping Island compared
with data fields for the Indian Ocean MORB array. Na8 versus
A. Si8; B. Ti8 and C. Fe8 were
calculated according to Klein & Langmuir (1987). Symbols
and data sources are as in A. Indian Ocean MORB are subdivided
into Types 1, 2 and 3 (cf., Figure 1C) at values of Na8 given
by the vertical lines (Natland, 1991). |
Figure 3 shows diagrams of CaO versus Al2O3 for
the same three data sets, except blown up in comparison to Figure
4 of Larsen & Pedersen (2009).The
diagrams are to the same scale, but have different values along the
abscissas and ordinates. In Figure 3A, for Kilauea and Puna Ridge,
the inflection between olivine-controlled and cotectic crystallization
is very sharp. No such inflection occurs either for BWG glass (Figure
3B) or Indian MORB. Again, BWG glass resembles fairly primitive to
moderately differentiated Type 1 Indian MORB (light blue data field),
including strongly plagioclase-phyric basalt from DSDP 212 and 236.
Whereas the data cluster for BWG glass is compact and without a strong
trend, it cannot be olivine-controlled (thin arrow). Instead plagioclase
and clinopyroxene must combine to produce trends like those shown
by the bold arrows, which go in the opposite direction and are oblique
to an olivine-control line drawn through the data. Vertical dispersion
of data points in Figure 3B results mainly from parental diversity,
glass from Padloping Island having higher Al2O3 than
most glass from West Greenland. This simply means that although all
glasses are cotectic compositions, they do not fall along the same
cotectic liquid line of descent. They do not have a common parent.
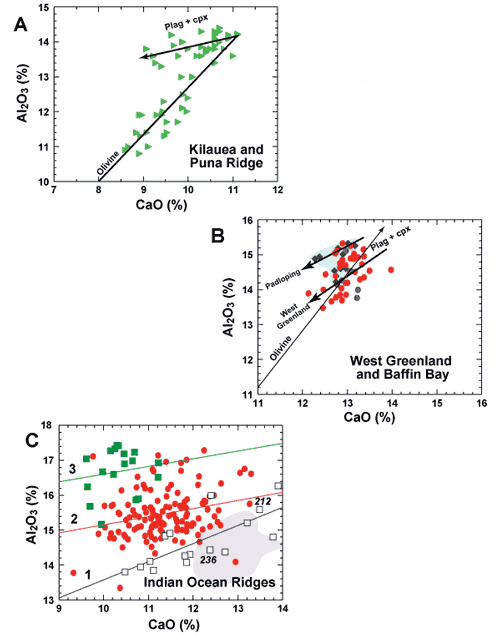
Figure 3: The contrast
between olivine-controlled and cotectic glass compositions:
CaO versus Al2O3 for basalt glass
from A. Kilauea and Puna Ridge; B. West Greenland and Padloping
Island, Baffin Bay; and C. Indian Ocean Ridges. Symbols
and data sources are as in Figure 1. Lines with arrows
in A and B are generalized hand-drawn trends (see text
for discussion). In C lines are linear regressions through
each data set for Types 1, 2 and 3 Indian MORB. Note that
although the diagrams are to the same scale, values of
abscissas and ordinates are not the same. In C, the area
for Baffin-West Greenland glasses in B is shown as a light
purple field. Data points for two sites of the Deep Sea
Drilling Project, DSDP 212 and 236, are labeled. |
From these considerations, I reiterate that analyzed
glass from Baffin Bay and West Greenland is unsuitable for backtrack
calculations to estimate temperature. They are cotectic compositions.
The further out along the cotectic trend a glass is in terms of iron
enrichment, the greater will be the tendency to overestimate both
crystallization temperatures of any olivine in the glass, and then
potential temperature.
The Larsen & Pedersen (2009)
Alternative
Larsen & Pedersen (2009) suggest that every analyzed glass of the Vaigat Formation is precisely at
the end of olivine control–just at the point where clinopyroxene,
plagioclase, or both join the liquidus, and no further along it.
In other words, each is an independent composition upon eruption,
derived from some high-MgO liquid that intrinsically had
different TiO2, total FeO, CaO, Al2O3,
and Na2O contents–all the oxides conventionally
used on variation diagrams to reveal shallow cotectic crystallization,
as I have just done. That is, the contents of these oxides varied at
the source in such a way as to mimic shallow cotectic crystallization
after all olivine had separated out polybarically upon ascent from
the mantle, but yet which reached proportions of 20-30% accumulation
in the same hosts.
I find it implausible that no cotectic variation
occurred, that every BWG glass formed uniquely from an independent
picritic parental composition almost as we see it, and that only
some olivine needs to be added back to it to have it in its original
state. Even less plausibly, the glass compositions now are precisely
such as to fool us into thinking that they exhibit a shallow, MORB-like,
plagioclase-dominated variation complete with negative Eu-anomalies.
Now variation diagrams are a powerful means of getting
at differences in parental compositions at the source, by sorting
out the effects of shallow crystallization. I claim to see shallow
effects in BWG glass whereas Larsen & Pedersen (2009) deny
them. To justify their temperature backtrack calculations, they pass
over the manner in which petrologists have treated obvious trends
on such diagrams for more than 40 years. I simply say that whether
or not any antecedent magma crystallized at high temperature, the
host glasses cannot be used for backtrack calculations. They are,
by the principle of least astonishment, cotectic liquid compositions.
Two questions remain, how do you get at temperature, and where is
the plagioclase?
Melt Inclusions
Larsen & Pedersen (2009) and
I agree that melt inclusions are too complicated to use for backtrack
calculations. However, to the extent that those with > 6%
total iron as FeO roughly mimic low-temperature cotectic crystallization, and occur
in forsteritic olivine, they might give clues about temperature.
I pointed out that all of them from West Greenland and most of
them from Baffin Bay have lower iron than host glasses to olivine.
Then, on the assumption that the inclusions are olivine-controlled
liquids, backtrack estimates suggest lower temperatures
than inferred from host glasses. The assumption of olivine control
is unproven, but the general point remains that low-iron melt
stems contributed to the overall composition of the final aggregated
picrite, and olivine as forsteritic as Fo92.8 contains
these inclusions. Low iron means low temperature in backtrack
calculations. The inclusions confirm that the particular olivine
that holds them did not crystallize along a common liquid line
of descent leading to the current host. The relevant liquid seen
as an inclusion was demonstrably different.
Magma Mixing
Figure 4 shows spinel compositions for several West Greenland
basalts of the Vaigat Formation (Larsen & Pedersen,
2000, supplemental data). The data may be viewed in aggregate,
or alternatively that they reveal populations of spinel with different
compositions in the same rocks, and thus are evidence for mixing
between primitive and differentiated liquids. One population, Type
A, seen in three of the samples shown, has high Cr# and high Mg#,
and is occluded within forsteritic olivine. (>Fo90).
Another population in the same three samples, Type B, has both
lower Cr# and Mg# and occurs within less forsteritic olivine. It
resembles spinel from Indian Ocean Type 1 and 2 basalt (red boundary).
Rare spinel with high TiO2, and falling outside both
populations in Figure 3, occurs in two samples and crystallized
in strongly differentiated liquids.
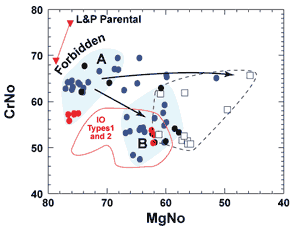
Figure 4: A portion
of the standard diagram of Mg# versus Cr# for Cr-spinel
in four samples from West Greenland (Larsen & Pedersen,
2000), to show that spinel compositions comprise two
principal clusters of data. Samples are Vaigat Formation
136943, blue dots; 332771, black dots; 362148 red dots;
and 326789, open squares. Blue backgrounds indicate Type
A, spinel with high Cr# and Mg# in forsteritic (>Fo90)
olivine, and Type B, spinel with lower Cr# and Mg# in
more iron-rich olivine. Sample 326789 (dashed boundary)
lacks Type A spinel, and includes some that have both
lower Mg# (higher iron) and higher TiO2 than
Type B spinel. Lines with arrows indicate possible trends
for magma mixing. Type B spinel are similar to spinel
in Types 1 and 2 Indian MORB (red boundary; J. Natland,
unpublished data). Also shown are two spinel compositions
(inverted brown triangles) calculated using Poustovetov & Roeder
(2000) from a high-MgO parental composition derived by
backtracking (Larsen & Pedersen, 2000). These have
higher Cr# and Mg# than any Type A spinel, including
spinel within the olivine used for the backtrack calculation. |
One sample lacks forsteritic olivine, all its spinel is Type B
and it occurs in olivine as iron rich as Fo84. This
is fully consistent with the host liquid lying along a low-pressure
cotectic, as also indicated by the similarity between Type B spinel
compositions and those predicted from host glass (Natland,
2008) using the procedure of Poustovetov & Roeder (2000).
This demonstrates that the host liquids were along a low-pressure
cotectic, and that most spinel in the samples (Type B) crystallized
from melts like the host liquids.
I suggest now that mixing with something containing very primitive
olivine and spinel (Type A) and primitive affiliated melt, also
occurred. Olivine-rich crystal sludges were incorporated into differentiated
basaltic liquid. Preliminary calculations for Padloping Island
based on variation diagrams using new data (Starkey et al., 2009),
suggest that the average composition of the primitive melt there
had about 0.8% TiO2, 8% FeOT and 10.5% MgO
(which is in equilibrium with ~ Fo90 olivine), consistent
with compositions of melt inclusions in Fo88-92 olivine.
The liquid represented about 50% of a mixture that otherwise contained
olivine of average composition Fo87.8 (240 analyses
from Larsen & Pedersen, 2000, Supplemental Data; 15
analyses from Francis, 1985). Somewhat more magnesian
precursors to this average primitive liquid precipitated olivine
with >Fo90 compositions. Picritic whole-rock analyses
thus do not strictly define simple olivine addition control trends;
mixing involved olivine and two liquids, as originally
suggested for Kilauea by Clague et al. (1995). This by
itself precludes using picrite FeO-MgO variation as the basis for
an incremental olivine-addition backtrack procedure to construct
olivine fractionation curves and estimate parental compositions
and temperatures. The present host glasses were also not precisely
cotectic liquids, but hybrids between cotectic liquids and more
primitive liquids; the hybrids thus lie within the olivine primary
phase volume and no longer have plagioclase on the liquidus.
The sample with only Type B spinel is an example of a differentiated
picrite of the kind common in Hawaiian, Juan Fernandez and Samoan
basalt (Natland, 2003; 2008).
Such picrite belies the notion that even a full mountain of picrite
necessarily represents high temperature eruption in its totality.
Of the 20,000 km3 of Vaigat Formation picrite, how much
of it is like this?
Primitive Olivine and Spinel
Since the backtrack-technique is questionable, we must focus on
the origin of primitive spinel and coexisting forsteritic olivine
to try to understand temperature. I suggested (Natland,
2008) that very forsteritic olivine could represent something
that crystallized very long ago, and which might be present as
a reconstituted xenocryst, perhaps originally in an ultramafic
cumulate, or as part of peridotite restite left over from formation
of Archaean continental crust. There is no doubt that the latter
material, with appropriately magnesian olivine, is present in the
region (Bernstein et al., 2006, 2007). I noted the suggestion
of prior workers (especially Francis, 1985) that the most
forsteritic olivine at Padloping Island (Baffin Bay) is kink-banded
or it has subgrains, thus is xenocrystic.
In the Vaigat formation, however, olivine with composition >Fo90 has
magmatic compositions (with small but significant amounts of CaO
and Cr2O3), has glass inclusions, no subgrains,
and is not kink-banded (Larsen & Pedersen,
2009). This is all to the good. Thus we may use compositions
of coexisting olivine and spinel (Larsen & Pedersen,
2000 Supplemental data) to understand the compositions of the liquids
that produced them. The compositions of parental liquids estimated
by Larsen & Pedersen (2000) predict spinel compositions
with both higher Cr# and Mg# than any spinel they analyzed. Spinel
in the very olivine used in the calculation is not as magnesian
as predicted. My estimate from spinel was that both the olivine
and the spinel crystallized from somewhat less magnesian liquids,
with ~15% MgO rather than ~20% MgO. This makes a difference of
about 150° less in crystallization temperature, and it mainly
means that the liquids chosen for backtrack calculations have too
much iron, as they should, since they are along a low-temperature
cotectic.
In Figure 4, the difference between the locations of most primitive
spinel (highest Cr# and Mg#) and spinel predicted from postulated
parental compositions represents the “forbidden zone” in
Figure 4 of Natland (2008).
It is not an interval of “intratelluric crystallization”,
as suggested by Larsen & Pedersen (2009),
for the reason just given. The gap simply means that the glass
used in the computation had too much iron, as indicated above,
thus higher MgO content is needed to get a liquid into equilibrium
with the forsteritic olivine in the sample. Lower the initial iron,
as indicated by spinel and the melt inclusions, and then even assuming
the propriety of the backtrack procedure, which I do not, the estimated
parental MgO drops to about 15%. This still gives fairly high temperatures
(~1350°C), but nowhere near the 1500-1550°C of Pedersen & Larsen (2000).
Xenocrysts?
Are mantle xenocrysts represented in Vaigat Formation picrites?
I remain struck by the coincidence that the glasses are MORB-like
and yet neither open-ocean MORB nor abyssal peridotite have olivine
with composition >Fo91. No MORB glass is olivine
controlled (Presnall & Gudfinnsson, 2008). Something
very specific to Baffin Bay and West Greenland produced such olivine
in an otherwise ocean-crust setting. A plume head bringing each
and every geochemical variation from depth is kind of a magical
solution, but like any deux ex machina, not a very satisfactory
explanation. I think the actual mechanism was more prosaic.
Thus olivine of composition Fo91-93 does occur in harzburgite-dunite
xenoliths of Archaean lithosphere in this region. Is it unreasonable
to suppose that such olivine, present here but not beneath spreading
ridges, is in some way the source of very forsteritic olivine in
West Greenland picrite? Unfortunately, that olivine has low CaO.
I offer three additional possibilities to account for the higher
CaO in BWG olivine phenocrysts, forewarning at the outset that
all are contrived. The three might act in tandem.
First, from experiments, olivine is known to re-equilibrate rapidly
with melt in which it is immersed (Gaetani & Watson,
2000). Although those experiments mainly dealt with effects of
re-equilibration on FeO, effects on other elements cannot be ruled
out. Here is an example. My own data on compositions of olivine
in Samoan xenoliths and phenocrysts show quite variable and overlapping
CaO contents from 0.03-0.51% in residual harzburgite (Type 1; Frey & Prinz,
1978) and magmatic dunite-pyroxenite (Type 2) ultramafic xenoliths.
CaO in most olivine (<Fo90) in the dunite-pyroxenite
suite precisely matches that seen in West Greenland olivine of
composition >Fo90 (0.25-0.45%), but even olivine
in some residual harzburgite has high CaO. Were some of the Samoan
olivine crystals in xenoliths re-equilibrated at their source by
percolating magma?
Second, could the Archaen upper lithosphere in this region contain
old ultramafic cumulates having Fo91-93 olivine, precipitated
perhaps from komatiitic magma? Presumably that olivine originally
had magmatic concentrations of CaO. Would that have been lost upon
re-equilibration to “mantle conditions”, or did it
remain the way it usually does in the ultramafic portions of ancient
layered igneous intrusions?
Thus forsteritic olivine in West Greenland picrites may have begun
as xenocrysts originally precipitated from ancient ultramafic magmas
and retaining the properties of magmatic minerals. The sources
were in shallow and relatively (over billions of years) cold sub-continental
lithosphere that was broken and fragmented during the early stages
of continental rifting, and traversed by MORB.
Finally, what would be the course of differentiation of primitive
MORB magma injected into and reacting with very refractory peridotite
encountered during continental rifting? MORB liquid reacts with
abyssal peridotite by precipitating olivine as dunite and extracting
pyroxene (e.g., Dick & Natland, 1996). In
general, provided the mass of transported liquid remains high,
(i.e., it is constantly resupplied and doesn’t diminish in
volume during the course of differentiation), the peridotite buffers
the Mg# of the liquid, and pulls it away from cotectic precipitation
of olivine and plagioclase. Concentrations of incompatible oxides
and trace elements (TiO2, Zr, etc., but perhaps also
Cr, depending on oxidation state?) will increase depending on the
volume of mantle that reacted with the magma. Primitive plagioclase-saturated
Type 1 or Type 2 Indian MORB reacting with Archaean harzburgite,
then, might shift to a composition in an olivine primary phase
volume, in which case forsteritic olivine and associated Cr-spinel,
but no other phases, would crystallize. The serious questions are
whether olivine phenocrysts of composition Fo91-93 and
affiliated spinel with both high Cr# and Mg# could form by such
a mechanism, and what would be the pressure and temperature of
their crystallization?
The key to evaluating this is very careful petrographic and mineral
study. It is not enough to state that the most magnesian olivine
in West Greenland picrite lacks subgrains or kink bands, and is
euhedral or skeletal, thus is obviously magmatic. The question
turns specifically on the morphology and composition of crystals
with composition Fo91-93, and the manner and composition
of their intergrowths of spinel and melt-inclusions. Are the olivine
grains broken? Are there internal grain boundaries? Do they have
euhedral overgrowths surrounding irregularly shaped cores? Are
the melt inclusions in embayments in outer zones of the mineral
grains (zoning itself is well documented by Larsen & Pedersen,
2000), or do they extend into their cores? Are the embayments skeletal
in origin, and formed at elevated undercooling, or did they originate
by resorption? For all the data that exist on these rocks, these
questions have not yet been answered. If they can be answered,
then all questions about backtrack techniques will be moot.
Cr2O3 in Olivine
Cr2O3 in olivine is not routinely measured
to high precision; Larsen & Pedersen (2000, 2009)
show its potential utility. At this point, I will simply say that
temperatures estimated for olivine using this parameter (Li
et al., 1995) can only coincidentally correspond to the backtrack
estimates of Larsen & Pedersen (2000); those I consider
to be unreliable for the reasons I have emphasized above.
Li et al. (1995) performed experiments in the four-component
systems, MgO-SiO2-Cr-O, most notably without FeO. The
temperature isopleths they calculated were for this system only;
the general tendency of additional components to lower equilibrium
temperatures remains a possibility. At that, Figure 2 of Larsen & Pedersen (2009),
which shows Fo (%) vs. Cr2O3 in olivine,
does not formally show higher Cr content in West Greenland olivine
than anywhere else, although Li et al. (1995) summarize
scant information for MORB olivine, saying that it has < 0.02%
Cr2O3. The general conclusion of Larsen
& Pedersen (2009),
that high Cr2O3 in West Greenland olivine
indicates high temperature, may be correct, but it needs to be
qualified with better understanding of how natural systems function,
more explicit comparisons to hypothetical lower-temperature places
like spreading ridges, and a question about how much hotter than
usual West Greenland olivine equilibration temperatures actually
had to be, in order to get the elevated Cr2O3 concentrations
in olivine. In short, what uncertainty is there to isopleths
in Figure 3 of Larsen & Pedersen (2009)?
A Suggestion
Figure 5 presents a generalized sketch showing possible schematic
relationships between a Paleocene magma chamber emplaced in high-level
peridotite but below granite at West Greenland. The difference
in elevation might represent the difference between the floor of
a rift valley lake, and a volcano emplaced at high levels on a
structural accommodation zone. It is based on geometrical models
proposed to explain why picrite occurs in structural depressions
such as Siqueiros fracture zone (Natland, 1989) and in
deep water at the tip of Kilauea’s Puna Ridge (Clague
et al., 1995), whereas differentiated lava predominantly occurs
higher.
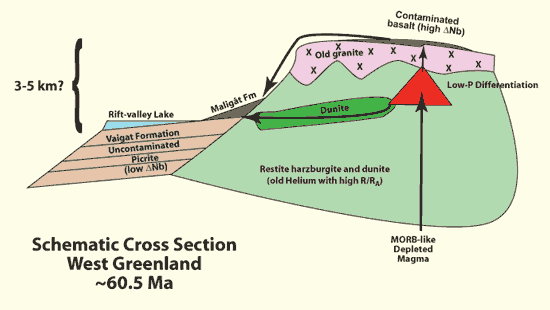
Figure 5: Schematic
cross section through the rifted West Greenland margin
during the Paleocene (~60. 5 Ma Storey et al., 2004).
See text for discussion. |
At West Greenland, I suggest that basaltic differentiation reached
and proceeded along the low-temperature multi-phase cotectic in
the magma chamber (red). A body of dunite (dark green) is shown
to lie along a curving listric fault extending into the adjacent
depression (cf., Clague & Denlinger, 1994), but it
may be more than one body. If it was there to begin with, it provides
xenocrysts. Alternatively, magma is emplaced along it as olivine-rich
sills (Boudier et al., 1996; Kelemen et al.,
1997), with olivine concentrating by flowage differentiation or
forming by reaction of the magma with harzburgite. When picrite
erupts, differentiated magma from the near the base of the chamber
that is uncontaminated by silicic continental crust flows along
the listric fault, encounters olivine-laden crystal sludges, scavenges
or entrains olivine and spinel, and pushes out onto the floor of
the depression. There it flows into lakes to form pillows and aquagene
tuffs with quenched glass. Basalt contaminated by continental crust
erupts above the magma chamber. Later, some of it flows far enough
to reach the depression (Maligât Formation), but only after
much picrite has erupted (Vaigat Formation). Helium with
high (old) 3He/4He (R/Ra) is incorporated
into primitive but otherwise uncontaminated magma migrating through
restite lithosphere (Natland,
2008). The ultimate source of primordial helium and its mobility
remain controversial (Starkey et al., 2009).
Finally, I suggest that the magma chamber was not a massive body
of circulating melt, a type of Skaergaard intrusion, but instead
was largely crystalline, much like that at spreading ridges, and
in which the network of crystals effectively served as a filter
to skein out phenocrysts (Natland & Dick, 1996). Crystallization
differentiation proceeded by precipitation of crystalline material
within the network of pre-existing crystals, and which had low
melt porosity. Compaction from overburden and tectonic deformation
assisted migration of the interstitial melt, and it vacated the
magma body into the listric fault zone by means of a type of filter
pressing. It left without phenocrysts, and shifted away from any
additional precipitation of plagioclase by continued reaction with
mantle rock. The sign of cotectic crystallization in BWG glass
is thus a response to cryptic variation during adcumulus growth;
it is no different from the mechanism that so consistently produces
eruption of strongly differentiated yet aphyric basalt on the summit
of the East Pacific Rise (Natland & Dick, 1996).
Helium
Where the main concern is temperature, I can only touch on noble
gases. Starkey et al. (2009) concluded that the helium
isotopic ratios of picrite from this region, which reach some extraordinarily
high values of 3He/4He, have nothing to do
with other trace elements and isotopes; those instead could reflect
other source variations (Starkey et al., 2009) and/or,
as I believe, crustal contamination. Starkey et al. (2009)
consequently invoke an independent non-lithospheric source for
helium with high-3He/4He. To my mind, this
is a matter of where and when. The key to very high-3He/4He
is age. Wherever the He is, it retains an isotopic ratio consistent
with something that happened very early in Earth history, perhaps
nearly from the accretionary dawn of time. I also consider that
old helium was likely separated from U and Th, and in general from
the magmaphilic isotopes of Sr, Nd and Pb, by magmatic processes.
The problem here is not one of the partition coefficient between
helium and olivine, as people most commonly tend to treat it, but
of partitioning between melt and vapor; then the nucleation of
bubbles on growing crystals; and finally the separation from molten
liquid of crystal-rich rocks (cf. Natland, 2003).
So picture some very old event–a magma ocean? komatiites?
formation of continental crust?–and the upper mantle that
supplied those rocks. In short, picture a process of separation
of He from U and Th in crystal cumulates or segregations, that
is, in rocks that are entirely possible to lie now at deep levels
beneath Archaean continental crust that is billions of years old.
That is where helium with very high-3He/4He,
and isolated from U and Th, could still be. Being a mobile volatile,
it may no longer be precisely where it was to begin with, but that
is why helium with high-3He/4He is found
in some residual mantle xenoliths (Poreda & Farley,
1992) and why it seeps into formation fluids in cold sedimentary
basins (Castro
et al.,
2009). Thus it was in the pathway of migrating BWG picrites
during the Paleocene (Figure 5). Olivine is a helium time capsule,
and the bubbles within it are easily cracked by migrating magma
and even by simple fracturing.
Summary
I hold to my views. Backtrack calculations using olivine-liquid
FeO-MgO equilibria to estimate temperatures of crystallization
of olivine in liquids postulated once to have been in equilibrium
with the mantle are suspect. Even for Baffin Bay and West Greenland,
where picrite abounds, the actual details of their crystallization
and mixing histories confound the basic assumptions behind the
backtrack technique, viz:
- that all olivine in a picritic sample crystallized along
a single olivine-controlled line of descent;
- that olivine-addition control lines are strictly that;
- that host liquids to picrites themselves represent olivine-controlled
liquids.
These assumptions result in calculation of parental liquids with >20%
MgO contents and crystallization temperatures in excess of 1500°C,
but they are wrong. The procedure of Poustovetov & Roeder (2000)
that relates liquid and spinel compositions to temperature instead
suggests maximum MgO content of ~15% for West Greenland picrite,
and about 1350°C for the temperature of crystallization of
spinel intergrown with the most magnesian olivine found in any
West Greenland sample (Fo92.8). A technique utilizing
Cr2O3 in olivine suggests higher temperatures
than those estimated from spinel crystallization, but differences
between the two techniques are not yet resolved. Additional detailed
petrographic and compositional studies of olivine Fo91-93 and
its inclusions of spinel and glass are warranted.
I suggest instead that BWG picrites result principally from injection
of fairly typical depleted MORB into and through ancient subcontinental
lithosphere and perhaps lower continental mafic crust during initial
continental breakup. Interaction with that lithosphere and crust
slightly perturbed the bulk compositions of the magmas and added
abundant olivine phenocrysts. The geochemical signal is mainly
that of depletion, but with some effects (e.g., change
in ΔNb and 3He/4He; Figure 5) incorporated
from the rocks through which the magmas passed.
Acknowledgments
Comments by Dean Presnall, Don Anderson and Dave Clague and a
review by Natalie Starkey are greatly appreciated. Gill Foulger
thoughtfully curbed some excesses.
References
- Bernstein, S., Hanghoj, K., Kelemen, P. B., and Brooks, C.
K., 2006. Ultra-depleted, shallow cratonic mantle beneath West
Greenland: Dunitic xenoliths from Ubekendt Ejland, Contributions
to Mineralogy and Petrology 152, 335-347,
doi:10. 1016/S0012-821X(97)00175-I.
- Bernstein, S., Kelemen, P. B., and Hanghoj, K., 2007. Consistent
olivine Mg# in cratonic mantle reflects Archean mantle melting
to the exhaustion of orthopyroxene. Geology 35,
4570462: doi:10. 1130/G23336A. I.
- Boudier, F., Nicolas, A., and Ildefonse, B., 1996. Magma chambers
in the Oman Ophiolite: fed from the top and the bottom, Earth
and Planetary Science Letters 144, 239-250.
- Castro,
M. C., Lin, M., and Hall, C. M., 2009. A primordial, solar
He-Ne signature in crustal fluids of a stable continental region. Earth
and Planetary Science Letters 279: 174-184.
- Clague, D. A., and Bunch, T. E., 1976. Formation of ferrobasalt
at East Pacific mid-ocean ridge spreading centers. Journal
of Geophysical Research 81: 4247-4256
- Clague, D. A.,and Denlinger, R. P., 1994. Role of olivine cumulates
in destabilizingh the flanks of Hawaiian volcanoes. Bulletin
Volcanologique 56: 425-434.
- Clague, D. A., Moore, J. G., Dixon, J. E., and Friesen, W.
B., 1995. Petrology of submarine lavas from Kilauea’s Puna
Ridge, Hawaii, Journal of Petrology 36:
299-349.
- Dick, H. J. B. and Natland, J. H., 1996. Late-stage melt evolution
and transport in the shallow mantle beneath the East Pacific
Rise. In Mével, C., Gillis, K., Allan, J., and
Meyer, P. (eds. ), Proceedings of the Ocean Drilling Program,
Scientific Results 147: College Station,
TX (Ocean Drilling Program), 103-134.
- Drever, H. I. & Johnston, R. 1957. Crystal growth of forsteritic
olivine in magmas and melts. Transactions of the Royal Society,
Edinburgh 63: 289-315.
- Fitton, J. G., Saunders, A. D., Norry, M. J., Hardarson, B.
S., and Taylor, R. N., 1997. Thermal and chemical structure of
the Iceland plume, Earth and Planetary Science Letters 153:
197-208.
- Francis, D., 1985. The Baffin Bay lavas and the value of picrites
as analogues of primary magmas, Contributions to Mineralogy
and Petrology 89: 144-154.
- Frey, M. A.,and Prinz, M.,1978. Ultramafic inclusions from
San Carlos, Arizona; petrologic and geochemical data bearing
on their petrogenesis. Earth and Planetary Science Letters 38:
129-176.
- Gaetani, G. A., and Watson, E. B., 2000. Open system behavior
of olivine-hosted melt inclusions, Earth and Planetary Science
Letters 183: 27-41.
- Kelemen, P. B., K. Koga, and N. Shimizu, 1997. Geochemistry
of gabbro sills in the crust–mantle transition zone of
the Oman Ophiolite: implications for the origin of the oceanic
lower crust, Earth and Planetary Science Letters 146:
475-488.
- Klein, E., and Langmuir, C. H., 1987. Global correlations of
ocean ridge basalt chemistry with axial depth and crustal thickness, Journal
of Geophysical Research 92: 8089-8115.
- Larsen, L. M., and Pedersen, A. K, 2000. Processes in high-Mg,
high-T magmas: Evidence from olivine, chromite and glass in Paleogene
picrites from West Greenland Journal of Petrology 41:
1071-1098.
- Larsen, L. M. and Pedersen, A.
S., 2009 ,West Greenland was hot – how else can you make
22,000 km3 of picrites? Paper posted at http://www.
mantleplumes. org/GreenlandHot.html
- Li, J. -P., O’Neil, H. St C. & Seifert, F. 1995.
Subsolidus phase relations in the system MgO-SiO2-Cr-O
in equilibrium with metallic Cr, and their significance for the
petrochemistry of chromium. Journal of Petrology 36,
107–132.
- Maclennan, J., McKenzie, D., Grönvold, K., Shimizu, N.,
Eiler, J. M., and Kitchen, N., 2003. Melt mixing and crystallization
under Theistareykir, northeast Iceland. Geochemistry, Geophysics,
Geosystems 4: 11, 8624, 40 p., doi:10.
1029/2003GC000558.
- Natland, J. H., 1989. Partial melting of a lithologically heterogeneous
mantle: Inferences from crystallization histories of magnesian
abyssal tholeiites from the Siqueiros Fracture Zone. In: Saunders,
A. D. and Norry, M. (eds. ), Magmatism in the Ocean Basins,
Geological Society of London, Special Publication 42:
41-77.
- Natland, J. H., 1991. Indian ocean crust. In Floyd,
P. A. (ed. ), Ocean Basalts:London (Blackie & Sons),
289-310.
- Natland, J. H., 2003. Capture of mantle helium by growing olivine
phenocrysts in picritic basalts from the Juan Fernandez Islands,
SE Pacific, Journal of Petrology 44:
421-456.
- Natland, J. H., 2007. ΔNb and the role of magma mixing
at the East Pacific Rise and Iceland. In Foulger, G.
R., and Jurdy, D. M., eds., Plates, Plumes and Planetary
Processes, Geological Society of America Special Paper 430:
413-449, doi: 10. 1130/2007. 20430(21).
- Natland, J. H. 2008. Eruptive temperatures
of the Paleogene picrites of West Greenland and Baffin Bay.
Paper posted at http://www. mantleplumes. org/Greenland.html
- Natland, J. H. and Dick, H. J. B., 1996. Melt migration through
high-level gabbroic cumulates of the East Pacific Rise at Hess
Deep:Inferences from rock textures and mineral compositions. In Mével,
C., Gillis, K., Allan, J., and Meyer, P. (eds. ), Proceedings
of the Ocean Drilling Program, Scientific Results 147:
College Station, TX (Ocean Drilling Program), 21-58.
- O’Hara, M. J., 1968. The bearing of phase equilibria
studies on the origin and evolution of igneous rocks, Earth-Science
Reviews 4: 6-133.
- Poreda, R. J., and Farley, K. A., 1992. Rare gases in Samoan
xenoliths. Earth and Planetary Science Letters 113:
129-144.
- Poustovetov, A., and Roeder, P. L., 2000. The distribution
of Cr between basaltic melt and chromian spinel as an oxygen
geobarometer, Canadian Mineralogist 39:
309-317.
- Presnall, D. C., and Gudfinnsson, G. H., 2008. Origin of oceanic
lithosphere, Journal of Petrology 49:
615-632, doi:10. 1093/petrology/egm052
- Robillard, I., Francis, D., and Ludden, J. N., 1992. The relationship
between E- and N-type magmas in the Baffin Bay lavas. Contributions
to Mineralogy and Petrology 112: 230-241.
- Starkey, N. A., Stuart, F. M., Ellam, R. M., Fitton, J. G.,
Basu, S., and Larsen, L. M., 2009. Helium isotopes in early Iceland
plume picrites: Constraints on the composition of high-3He/4He
mantle. Earth and Planetary Science Letters 277:
91-100 doi:10. 1016/j. epsl2008. 10. 007.
- Storey, M., Pedersen, A. K., Stecher, O., Bernstein, S., Larsen,
H. C., Larsen, L. M., Baker, J. & Duncan, R. A. 2004. Long-lived
postbreakup magmatism along the East Greenland margin: Evidence
for shallow-mantle metasomatism by the Iceland plume. Geology 32:
173–176.
- Wright, T. L, 1971. Chemistry of Kilauea and Mauna Loa lava
in space and time, United States Geological Survey Professional
Paper 735.
- Wright, T. L., and Fiske, R. S., 1971. Origin of differentiated
and hybrid lavas of Kilauea volcano, Hawaii. Journal of Petrology 12:
1-65.
last updated 7th March, 2009 |