 |
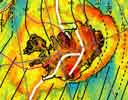 |
The
Iceland “Anomaly” – An Outcome
of Plate Tectonics
|
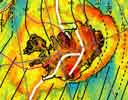 |
|
1Erik
Lundin & 2Tony Doré
1StatoilHydro
ASA, Research Centre, 7005 Trondheim, NORWAY
ERLUN@StatoilHydro.com
2Statoil
UK Ltd., Statoil House, 11a Regent Street, London
SW1Y 4ST, U.K.
agdo@statoil.com
Click here to
download a PDF version of this webpage
|
Click here to
go to News & Discussion of this page |
Abstract
Iceland is commonly considered
to be the surface expression of a plume originating
at the core-mantle boundary. Likewise, Paleocene magmatism
in the NE Atlantic (NEA) is typically ascribed to
thermal effects from the proto-Iceland plume, which
furthermore is often invoked as a decisive factor
in NEA breakup. We argue that neither the present-day
Iceland anomaly, nor its supposed ancient manifestation,
is related to a deeply-rooted plume. We also propose
that NEA breakup can be explained as a natural outcome
of plate tectonics, not requiring any plume weakening
of the lithosphere. In contrast to the common perception
that the Greenland-Faroes Ridge is a hot spot track
related to the Iceland plume, we consider it a symmetric
construction that formed above an upper-mantle upwelling
maintained at the plate boundary. We relate the two
pulses of NEA magmatism to separate tectonic phases
of North Atlantic breakup:
-
Early Paleocene magmatism
(c. 62-58 Ma) was governed by a short-lived attempt
at seeking a new rift path, intermediate in time
and space between the Labrador Sea – Baffin
Bay and the NEA-Eurasia Basin rifts,
-
The voluminous Early Eocene
magmatism (c. 56-53 Ma) along the NEA margins was
related to final breakup of Pangea and exploitation
of the collapsed Caledonian fold belt.
We consider both the NEA magmatism
and the current Iceland anomaly to represent “top
down” effects of plate tectonics. |
Implications of Greenland-Faroes
Ridge symmetry
In a recent review, Iceland was placed
in an exclusive group of seven hot spots, supposedly
related to plumes originating from the core-mantle boundary
(Courtillot
et al., 2003). Others argue that, while there
is good evidence from seismic tomography for an upper
mantle velocity reduction beneath Iceland, the anomaly
cannot be proven to reach the Earth’s core (e.g.,
Foulger et al., 2000,
2001).
The Iceland anomaly is located on the
aseismic Greenland-Faroes Ridge (GFR), proposed to be
all or part of a “hot spot track” (e.g.,
Morgan, 1971,
1981; Holbrook & Keleman, 1993; Lawver
& Müller, 1994) above a deeply rooted,
fixed plume. This view has encouraged workers to estimate
the position of the Iceland “hot spot ”
through time (e.g., Forsyth et al., 1986; Lawver
& Müller, 1994; Torsvik et al.,
2001a) (Figure 1). Such estimates assume a fixed point-like
“plume” located under South Central or West
Greenland during Early Paleocene. As Greenland moved
northwestward the proposed plume supposedly emerged
beneath the East Greenland margin and gradually entered
the NE Atlantic (Figure 1). There is, however, to our
knowledge no a priori evidence for a time-transgressive
path of the “hot spot” eastwards towards
present-day Iceland. Furthermore, a corollary to such
estimates is that the “hot spot” or plume
centre cannot have been located east of its current
position, which presents a problem since physiography
(Figure 1), crustal structure/thickness (Bott,
1983; Smallwood et al., 1999; Holbrook
et al., 2001; Foulger et al., 2002,
2003b)
and magnetic data (Figure 2) suggest symmetry of the
GFR about Iceland.
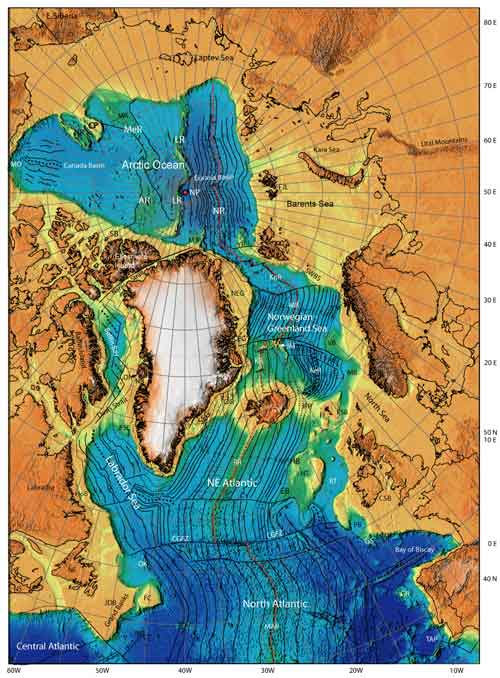
Figure 1. Location map. Bathymetry
and topography from Lundin (2002). Based on data from
Smith & Sandwell (1997) and Jakobsson et al. (2000),
overlain by our interpreted magnetic anomalies, fracture
zones and spreading axes (black dashed = extinct, red
dashed = active). MJP: Morris Jesup Plateau, YP: Yermal
Plateau. MJP is off N Greenland, and YP is just west
of Svalbard. KnR: Knipovich Ridge. Click on image to
enlarge.
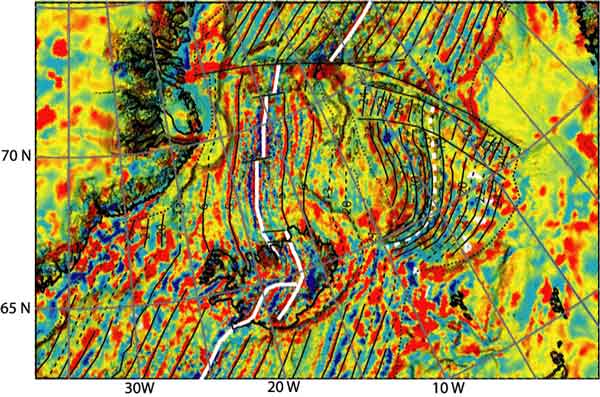
Figure 2a. Shaded relief image of
magnetic data (Verhoef et al., 1996) draped on bathymetry
(Smith & Sandwell, 1997). Solid white lines = active
spreading axes, dashed white line = abandoned Aegir
Ridge, thin solid black lines = interpreted magnetic
anomalies, thick solid black lines = fracture zones;
dotted black lines = Continent-Ocean Boundary (COB).
We attribute the patchy magnetic pattern to subaerial
lava extrusion (e.g., Bott, 1983), interacting with
the topography of pre-existing flows, and being further
complicated by erosion until the ridge subsided below
the wave base.
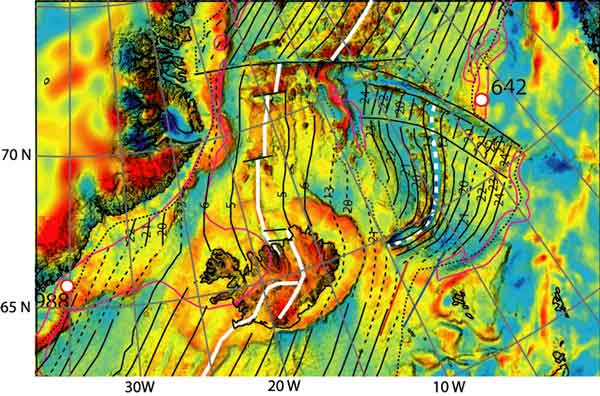
Figure 2b. Shaded relief image of
free air gravity (Andersen & Knudsen, 1998) draped
over bathymetry (Smith & Sandwell, 1997). Symbols
as in Figure 2a. Purple lines = distribution of seaward
dipping reflectors (from Planke & Alvestad, 1999).
Vink (1984) recognized the
paradox of explaining the development of the GFR in
a fixed hot spot framework, and proposed a model whereby
asthenosphere was channelled the shortest distance from
a presumed plume centre under Greenland to the nearby
Reykjanes Ridge. However, with such a model a pronounced
V-shaped hot spot-fed plateau should have formed, since
palaeomagnetic data reveal that North America, Greenland,
and Eurasia have moved significantly northward since
break-up (e.g., Torsvik et al., 2001b). To
a first order, the GFR is linear (Figure 1), contradicting
Vink's model. |
The Iceland anomaly through geological history
Following White et al. (1987) and White
(1988) most workers explain the Early Tertiary volcanism
of the North Atlantic Igneous Province (NAIP) in terms
of lithospheric impingement of the proto-Iceland mantle
plume. A wide variety of beliefs on the size and morphology
of the plume exist, from a single point (e.g.,
Forsyth, 1986; Lawver & Müller,
1994; Torsvik et al., 2001a) (Figure 1) to
a continental-scale mantle anomaly acting simultaneously
on areas separated by some 2000 km (Smallwood
& White, 2002).
NAIP magmatism can be divided into two phases (e.g.,
Saunders et al., 1997):
-
“Middle” Paleocene
(c. 62-58 Ma) continent-based magmatism in Britain
and West Greenland, and
-
the voluminous latest Paleocene
to earliest Eocene (c. 56-53 Ma) magmatism along
the NEA margins.
A crucial problem with the “fixed hot spot”
and the “global hot spot reference frame”
is the supposed position of the Iceland plume centre
beneath West Greenland at the onset of NAIP magmatism
in the Early Paleocene (c. 62 Ma). Such a position
is not consistent with the more or less simultaneous
onset of basaltic magmatism between this area and
southeastern NAIP, for example in the Hebrides (e.g.,
Jolley & Bell, 2002; Ritchie et al.,
1999). An equally tricky problem to explain is the
lack of magmatism in areas that should have been in
close proximity to the plume, such as the already-established
passive margin of SW Greenland.
Inconsistency between model and observations in the
NAIP has led to various adjustments of the Morgan
(1971) plume hypothesis, such as
-
invoking separate plumes (Morgan,
1983; Srivastava, 1983),
-
a plume split into two arms arriving
at different times (e.g., Holm et al.,
1993),
-
an ultrafast plume spreading
out immense distances along the base of the lithosphere
(Larsen et al., 1999),
-
channelling of plume material
from beneath Greenland into the NE Atlantic spreading
axis (Vink, 1984),
-
blocking of plume material by
a step at the base of the lithosphere (Nielsen
et al., 2002),
-
a complete reworking of the plume
concept, abandoning the popular image of a rising
lava-lamp style blob in favour of one of ascending
sheets thousands of kilometres long (Smallwood
& White, 2002).
This proliferation of models can be viewed as “a
sign of a hypothesis in trouble” (Foulger,
2003a). What seems certain is that a Hawaii-style
model for plate motion over a deeply-rooted and fixed
plume is now untenable as an explanation for both
the NAIP and Iceland.
It appears possible to interpret the melting anomaly
associated with formation of the NEA volcanic passive
margin and present-day Iceland as a thin-skinned phenomenon
that has been centred on the constructional plate
boundary since its inception. This idea, however,
leaves open the origin of the early phase of NAIP
magmatism, extending between the BVP and W Greenland.
Early workers (e.g., Hall, 1981) called this
the “Thulean Volcanic Line”. It is characterized,
at least in its Eurasian portion, by intense NW-SE
dyke swarms, mainly mafic in character (e.g.,
Dewey & Windley, 1988; England,
1988), extending from the Hebridean province in an
ESE direction to the Central North Sea (Kirton
& Donato, 1985) and SE to the Bristol Channel
(e.g., Blundell, 1957). The frequency and
consistent trend of the dykes indicate a NE-SW extensional
stress field across Britain during that part of the
Paleocene (England, 1988). The early NAIP
may therefore, represent a transient failed attempt
by NW Europe and Greenland to break up along a NW-SE
axis, an idea previously suggested by Dewey &
Windley (1988). Such extension would logically
have been a continuation of mid-late Cretaceous stress
fields associated with N Atlantic opening (e.g.,
Johnston et al., 2001). Further expressions may
include the fjord and dyke grain of the Faroes, the
fjord grain of East Greenland, and recently reported
NW-trending half-graben structures containing Upper
Cretaceous and Palaogene shallow marine sediments
in the Christian IV Gletcher area (just east of Kangerlussuaq)
(Larsen & Whitham, 2003). In the volcanic
area of West Greenland both the fjord grain and a
set of Paleocene extensional faults trend northwest
(Nøhr-Hansen et al., 2002).
The NE-SW extensional stress field was replaced as
stretching and subsequent separation refocused on
the NEA margin in the later Paleocene - Early Eocene.
Both the early NAIP and the subsequent, NEA volcanic
passive margin development can be explained in terms
of plate tectonic processes – i.e. breakup of
a crust already stretched by numerous preceding extensional
episodes, above a labile and melt-prone mantle.
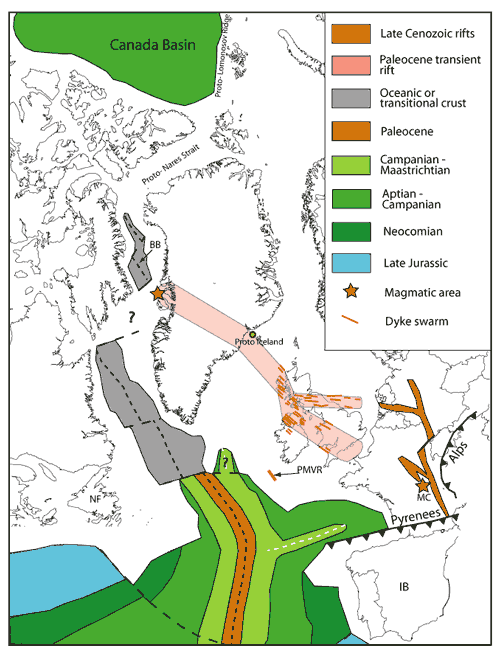
Figure 3. Plate reconstruction
to 60 Ma (Trond Torsvik, pers. com. 2003) with simplified
seafloor. The main dike trend in the British Volcanic
Province schematically shown to extend to the West
Greenland magmatic area, is invoked to utilize a zone
of weak extension. The Late Cenozoic European rift
system (from Ziegler, 1992) is included in order to
illustrate a more evolved stage extension, also related
to compression in the Pyrenees and the Alps. NF: Newfoundland,
BB: Baffin Bay, IB: Iberia
|
Final breakup of Pangea – linking the
North Atlantic and Arctic
Labrador Sea and Baffin Bay versus the Arctic
Opening of the Labrador Sea was a continuation of
the general northward propagation of the N Atlantic
that started between Newfoundland and Iberia in Hauterivian
time. Two schools of thought exist on the timing of
onset of spreading in the Labrador Sea; Roest
& Srivastava (1989) and Srivastava &
Roest (1999) interpret onset of spreading at
Chron 33 (c. 81 Ma) while Chalmers & Laursen
(1995) propose that seafloor spreading started in
Early Paleocene (Chron 27). Further north, in Baffin
Bay, magnetic data have recently been re-interpreted
to reveal the presence of magnetic Chron 26n or Chron
25n (middle Paleocene) (Oakey et al., 2003).
Regardless of the dispute about onset of spreading
in the Labrador Sea, it appears clear from the crustal
structure that seafloor never propagated beyond the
northern tip of Baffin Bay (e.g., Reid & Jackson,
1997).
We argue that when “seafloor spreading”
reached the northern tip of Baffin Bay in latest Cretaceous
or Early Paleocene time, it approached the by-then
c. 65 to 80 Ma old passive margin hinge zone to the
Canada Basin (approximately Hauterivian; Grantz
et al., 1990, Lawver & Baggeroer,
1983). The hinge zone probably acted as a barrier
to further propagation and triggered plate reorganization,
analogous to the manner the Neo-Tethyan hinge zone
hindered further propagation of the Red Sea –
Gulf of Suez rift (Steckler & ten Brink,
1986).
The Labrador Sea – Baffin Bay rift system preceeded
or overlapped in time with the transient Early Paleocene
rift through the BVP – W Greenland. Ultimately,
a new rift path in the NEA formed in Early Eocene
time, utilizing the collapsed Caledonian fold belt
and the associated Mesozoic rift system. Breakup in
the Arctic followed the Canada Basin shear margin
(Grantz et al., 1990), splitting off the
Lomonosov Ridge (a microcontinent), which is another
example of lithospheric strength control by the Canada
Basin on NEA-Arctic breakup.
During the following c. 20 Ma, simultaneous spreading
occurred along two arms of the North Atlantic –
the Labrador Sea/Baffin Bay and the NEA arms –
linked at a triple junction south of Greenland. The
resultant northward motion of Greenland induced the
Eurekan Orogeny (Oakey, 1994). The angle
of convergence between Greenland and the Candian Arctic
Islands was very high (Oakey, 1994), preventing
significant lateral motion along the Wegner Transform
(in Nares Strait) (e.g., Dawes & Kerr,
1982; Okulitch et al., 1990), in turn explaining
why the Labrador Sea/Baffin Bay arm of spreading was
unable to link with the Arctic Eurasia Basin. The
end of the Eurekan Orogeny coincided with the termination
of seafloor spreading in the Labrador Sea and Baffin
Bay at Chron 13 (c. 35 Ma).
The essential point from the foregoing discussion,
is that the abandonment of the Labrador Sea/Baffin
Bay arm of spreading, the transient Paleocene BVP-W
Greenland rift, and the final diversion of seafloor
spreading through the Caldeonian fold belt (the NEA
spreading arm) was a natural outcome of plate tectonic
reorganization, strongly influenced by lithospheric
strength distribution. Lithospheric weakening in the
NEA due to the arrival of a plume need not be invoked.
Linkage between the NE Atlantic and the Arctic
In large parts of the North Atlantic the magnetic
seafloor anomalies are well defined and of little
or no controversy. We follow prexisting interpretations
here. However, in the more complicated area surrounding
the Aegir and Kolbeinsey Ridges (e.g., Talwani
& Eldholm, 1977; Vogt et al., 1980;
Nunns, 1983; Jung & Vogt, 1997),
we have reinterpreted some of the continent-ocean
boundaries and magnetic anomalies (Figures 2a and
2b). We suggest that both the Aegir and Kolbeinsey
Ridges show classic signs of propagation (e.g.,
Vink, 1982), but in opposite directions. This
model for the Aegir and Kolbeinsey Ridges is rather
similar to the one by Nunns (1983), implying
simultaneous spreading on two complimentary and overlapping
spreading axes, and contrasts with the model implying
a ridge jump from the Aegir to the Kolbeinsey Ridge
(e.g., Talwani & Eldholm, 1977; Vink,
1984). Our interpretations of magnetic anomalies,
fracture zones, and COBs in the NEA and Norwegian-Greenland
Sea, have been used as the basis for a reconstruction
of magnetic grids (Lundin et al., 2002),
applying the method of Verhoef et al. (1990)
(Figure 4).
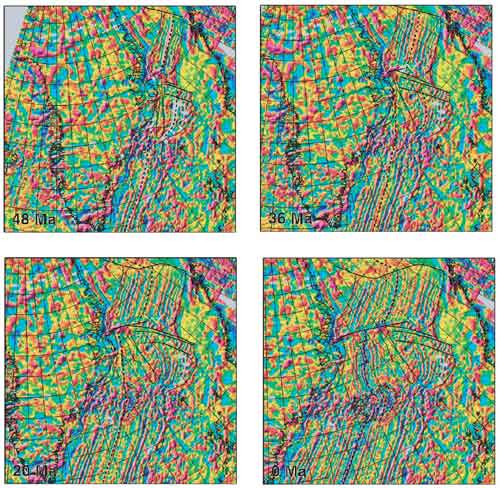
Figure 4. NE Atlantic and Norwegian-Greenland
Sea reconstruction of gridded magnetic data (Verhoef
et al., 1996), applying the method of Verhoef et al.
(1990). Reconstructed grid node positions were achieved
by rotating the grids according to plate reconstruction
parameters (Müller et al., 1997). The Euler poles
are listed in Table 1. These images were extracted
from an animation by Lundin et al. (2002). Click on
image to enlarge.
Greenland
versus Eurasia |
Jan
Mayen versus Eurasia |
Ma |
Lat |
Long |
Cum.
Rotation |
Lat |
Long |
Cum.
Rotation |
0 |
90 |
0 |
0 |
90 |
0 |
0 |
20 |
67.261 |
135.480 |
4.708 |
90 |
0 |
0 |
36 |
66.926 |
135.426 |
8.134 |
-64.619 |
168.090 |
10.397 |
48 |
57.236 |
131.360 |
9.372 |
-64.617 |
167.496 |
34.085 |
Table 1. Euler poles (interpolated
from Müller et al., 1997)
at the reconstruction steps shown in Figure 4. Eurasia
is held fixed.
|
Opening of the NEA and Arctic can be viewed as the
result of southward ridge propagation from the Arctic
and northward ridge propagation from the southern
North Atlantic, meeting in the area of proto-Iceland.
Hence, it is tempting to speculate on whether the
mantle upwelling at Iceland in some way relates to
the ridge convergence.
We suspect that “hot spot” upwellings,
at least at plate boundaries, are triggered and maintained
by the separating plates, as opposed to the other
way around. Other examples of Atlantic-Arctic “hot
spots” apparently forming at the plate boundary
are the Azores, Jan Mayen, Yermak, and possibly Tristan
da Cunha. All these “hot spots” have remained
at or near the constructive boundary since their inception,
possibly with the exception of Tristan da Cunha, which
has a gap in the “hot spot track” on the
west side of the S Atlantic, arguably marking a jump
from one side of the plate boundary to the other.
The Azores “hot spot” became active first
at c. 20 Ma (Gentle et al., 2003) and therefore,
clearly had no role in the creation of the Central
and North Atlantic spreading ridges (opening at c.
180 Ma and c.123 Ma respectively). According to our
interpretations, magmatism at the Morris Jesup and
Yermak Plateaus (Feden et al., 1979) can
be bracketed in time between opening of the Eurasia
Basin (c. 54 Ma) and Chron 13 (c. 35 Ma), when the
SW Barents Sea shear margin opened obliquely and inititated
a through-going spreading axis between the Arctic
and the NE Atlantic (the Knipovich Ridge). Little
is written about the Jan Mayen “hot spot”
(Morgan, 1981), but it must be young and
lies on the junction between the Mohns Ridge and the
West Jan Mayen Fracture Zone.
With respect to the cause of the voluminous NAIP
magmatism we recognize that more than one possibility
exists. The traditional view of elevated mantle temperature
remains attractive, although recent data on surface
heat flow suggests that Iceland is not anomalously
hot (Stein
& Stein,
2003). Even if mantle temperatures are elevated
we would argue that the hot mantle material does not
stem from the core-mantle boundary, but probably stems
from a shallower level in the Earth, such as the 660
km discontinuity (Hamilton,
2003). The possibility of a heterogeneous and
locally melt-prone upper mantle (e.g., Anderson,
1996, Foulger et al., 2004a,
2004b),
in particular related to the Caledonian fold belt,
is an attractive alternative process.
|
Conclusions
-
All evidence suggests that the
Iceland anomaly developed at the plate boundary
during breakup and has remained there throughout
its history.
-
The GFR is a symmetric subaerial
magmatic construction, formed above passively upwelling
upper mantle (cf. Foulger et al., 2000,
2001),
apparently of normal temperature ( Stein
& Stein,
2003). The GFR is not part of a classic time-transgressive
hot spot track, nor does such a track exist for
the Iceland “hot spot”.
-
We argue that the separating
plates have controlled the upwelling forming the
Iceland anomaly.
-
The early NAIP, characterized
by the BVP and conceivably extending to the West
Greenland volcanic area, is explained as a result
of weak NE-SW extension. This magmatism over a linear
domain 2,000 km long need not appeal to a mantle
plume of extraordinary shape and flexibility, but
can instead be viewed as a by-product of plate breakup.
-
The Iceland “plume”
is frequently cited as the causal factor in NE Atlantic
breakup, via lithospheric weakening. However, we
show from plate tectonic considerations that opening
of the NE Atlantic and Arctic Eurasia can be explained
as a natural consequence lithospheric strength control
on final breakup of Pangea, and need not appeal
to lithospheric weaking by a plume.
-
Linkage between the Arctic and
the N Atlantic can possibly be viewed as accomplished
by southward and northward propagating ridges. These
ridges overlapped in the region of proto-Iceland.
Conceivably, the Iceland mantle upwelling anomaly
is related to the convergence of these ridge tips.
-
We readily acknowledge that the
phenomena of melt production and regional uplift
around Iceland, and in the earlier NAIP, require
mantle upwelling. However, if these effects indeed
relate to existence of a deep-seated plume, an explanation
is required as to why the “hot spot”
has been fixed to the plate boundary throughout
its history. This observation is strongly discordant
with the assertion of Courtillot
et al.
(2003) that Iceland ranks as one of the world’s
most certain hot spots related to a plume rooted
at the core-mantle boundary. At the very least,
the time-transgressive “hot spot” from
Western Greenland to present-day Iceland, often
quoted as an inevitable outcome of the “hot
spot reference frame”, and used as an a
priori assumption in the literature, must be
seriously questioned.
|
News & Discussion
No
Plume Under Iceland
Iceland
plume: four articles, pro and con |
-
Andersen, O.B. & Knudsen,
P., 1998. Gravity anomalies from ERS-1 and Geosat
Geodetic Missions. Kart og Matrikelstyrelsen,
DK-2400 Copenhagen NV, Denmark.
-
Anderson, D.L. 1996. Enriched
asthenosphere and depleted plumes. Int. Geol.
Rev., 38, 1-21.
- Anderson, D.L.,
Top-down tectonics?, Science, 293,
2016-2018, 2001.
-
Blundell, D.J. 1957. A palaeomagnetic
investigation of the Lundy dyke swarm. Geol.
Mag., 94, 187-193.
-
Bott, M.H.P. 1983. The crust
beneath the Iceland-Faroe Ridge. In: Bott, M.H.P.,
Saxov, S., Talwani, M. & Thiede, J. (eds). Structure
and Development of the Greenland-Scotland Ridge:
New Methods and Concepts. Plenum Press, New
York, 63-75.
-
Chalmers, J.A. & Laursen,
H.K. 1995. Labrador Sea: The extent of continental
and oceanic crust and the timing of the onset of
seafloor spreading. Mar. Petr. Geol., 12,
205-217.
-
-
Dawes, P.R.& Kerr, W.J. 1982.
The case against major displacement along Nares
Strait. Meddelser om Grønnland, Geoscience,
8, 369-386.
-
Dewey, J.F. & Windley, B.F.
1988. Palaeocene-Oligocene tectonics of NW Europe.
In: Morton, A.C. & Parson, L.M. (eds). Early
Tertiary Volcanism and the Opening of the NE Atlantic.
Geol. Soc., London, Special Publications,
39, 25-31.
-
England, R.W. 1988. The early
Tertiary stress regime in NW Britain: evidence from
the patterns of volcanic activity. In: Morton, A.C.
& Parson, L.M. (eds). Early Tertiary Volcanism
and the Opening of the NE Atlantic. Geol.
Soc., London, Special Publications, 39,
381-389.
-
Feden, R.H., Vogt, P.R. &
Fleming, H.S. 1979. Magnetic and bathymetric evidence
for the “Yermak hot spot” northwest
of Svalbard in the Arctic Basin. Earth Planet.
Sci. Lett., 44, 18-38.
-
Forsyth, D.A, Morel, A.L., Huissier,
P., Asudeh, I. & Green., A.G. 1986. Alpha Ridge
and Iceland; products of the same plume? J.
Geodynamics, 6, 197-214.
-
Foulger,
G.R., Pritchard, M.J., Julian, B.R., Evans, J.R.,
Allen, R.M., Nolet, G., Morgan, W.J., Bergsson,
B.H., Erlendsson, P., Jakobsdottir, S., Ragnarsson,
S., Stefansson, R. & Vogfjörd. 2000. The
seismic anomaly beneath Iceland extends down to
the mantle transition and no deeper. Geophys.
J. Int., 142, F1-F5.
-
Foulger,
G.R., Pritchard, M.J., Julian, B.R., Evans, J.R.,
Allen, R.M., Nolet, G., Morgan, W.J., Bergsson,
B.H., Erlendsson, P., Jakobsdottir, S., Ragnarsson,
S., Stefansson, R. & Vogfjörd. 2001. Seismic
tomography shows that upwelling beneath Iceland
is confined to the upper mantle. Geophys. J.
Int., 146, 504-530.
-
-
-
- Foulger,
G.R. and D.L. Anderson. 2004a. A cool model for the
Iceland hotspot, J. Volc. Geotherm. Res.,
in press
-
-
Gentle, P., Dymont, J., Maia,
M. & Goslin, J. 2003. The Azores Plateau: an
example of ridge-hotspot interaction. 8th International
Congress of the Brazilian Geophysical Society,
Rio de Janeiro, Sep 14-18, 2003.
-
Grantz, A., May, S.D., Taylor,
P.T. & Lawver, L.A. 1990. Canada Basin. In:
Grantz, A., Johnson, L., & Sweeney, J.F. (eds).
The Arctic Ocean region, The Geology of North
America, v. L, Geological Society of America,
Boulder, Colorado, 379-402.
-
Hall, J.M. 1981. The Thulean
Volcanic Line. In: Kerr, J.W., Fergusson, A.J. &
Machan, L.C. (eds). Geology of the North Atlantic
borderlands. Canadian Society of Petroleum
Geologists, Memoir 7, 231-244.
-
-
Holbrook, W.S. & Keleman,
P.B. 1993. Large igneous province on the US Atlantic
margin and implications for magmatism during continental
breakup. Nature, 364,
433-436.
-
Holbrook, S.W., Larsen, H.C.,
Korenaga, J., Dahl-Jensen, T., Reid, I.D., Kelemen,
P.B., Hopper, J.R., Kent, G.M., Lizzarralde, D.,
Berstein, S. & Detrick, R.S. 2001. Mantle structure
and active upwelling during continental breakup
in the North Atlantic. Earth Planet. Sci. Lett.,
190, 251-266.
-
Holm, P.M., Gill, R.C.O., Pedersen,
A.K., Larsen, J.G., Hald, N., Nielsen, T.F.D. &
Thirlwall, M.F. 1993. The Tertiary picrites of West
Greenland: contributions from “Icelandic”
and other sources. Earth Planet. Sci. Lett.,
115, 227-244.
-
Jakobsson, N., Cherkis, N.Z.,
Woodward, J., Macnab, R. & Coaklay, B. 2000.
New grid of Arctic bathymetry aids scientists and
mapmakers. Eos, Trans. AGU, 81,
89-96.
-
Johnston, S., Doré, A.G.
& Spencer, A.M. 2001. The Mesozoic evolution
of the southern North Atlantic region and its relationship
to basin development in the south Porcupine Basin,
offshore Ireland. In: Shannon, P.M., Haughton, P.D.W.
& Corcoran, F.V. (eds), The Petroleum Exploration
of Ireland's Offshore Basins, Geol. Soc., London,
Special Publications, 188, 237-263.
-
Jolley, D.W. & Bell, B.R.
2002. The evolution of the North Atlantic Igneous
Province and the opening of the NE Atlantic rift.
In: Jolley, D.W. & Bell, B.R. (eds). The
North Atlantic Igneous Province, Tectonic, Volcanic
and Magmatic Processes. Geol. Soc., London,
Special Publications, 197, 1-13.
-
Jung, W-Y. & Vogt, P.R. 1997.
A gravity and magnetic study of the extinct Aegir
Ridge, Norwegian Sea. J. Geophys. Res.,
102, 5065-5089.
-
Kirton, S.R, & Donato, J.A.
1985. Some buried Tertiary dykes of Britain and
surrounding waters deduced by magnetic modelling
and seismic reflection methods. J. Geol. Soc.,
London, 142, 1047-1057.
-
Larsen, T.B., Yuen, D.A. &
Storey, M. 1999. Ultrafast mantle plumes and implications
for flood basalt volcanism in the Northern Atlantic
Region, Tectonophysics, 311,
31-43.
-
Larsen, M. & Whitham, A.G.
2003. Evidence for a major sediment input point
into the Faroe-Shetland basin from southern East
Greenland. 6th Petroleum Geology Conference,
Northwest Europe and global perspectives, Geol.
Soc., London, Oct 6-9, London.
-
Lawver, L.A. & Baggeroer,
A. 1983. A note on the age of the Canada Basin.
Alaska Geological Society Journal, 2,
57-66.
-
Lawver, L.A. & Müller,
D.R. 1994. Iceland “hotspot” track.
Geology, 22, 311-314.
-
Lundin, E.R. 2002. Atlantic –
Arctic seafloor spreading history. In: Eide, E.
A. (coord.), BATLAS – Mid Norway plate
reconstructions atlas with global and Atlantic perspectives.
Geological Survey of Norway, 40-47.
-
Lundin, E.R., Torsvik, T.H.,
Olesen, O. & Roest, W.R. 2002. The Norwegian
Sea, a meeting place for opposed and overlapping
Arctic and Atlantic rifts; implications for magmatism.
AAPG Hedberg Conference “Hydrocarbon Habitat
of Volcanic Rifted Passive Margins”,
September 8-11, 2002, Stavanger, Norway.
-
-
Morgan, W.J. 1981. Hotspot tracks
and the opening of the Atlantic and Indian Oceans.
In: Emiliani, C. (ed), The Oceanic Lithosphere,
John Wiley & Sons, New York, 443-487.
-
Morgan, W.J. 1983 Hotspot tracks
and the early rifting of the Atlantic. Tectonophysics,
94, 123-139.
-
Müller, R.D., Roest, W.R.,
Royer, J-Y, Gahagan, L.M. & Sclater, J.G. 1997.
Digital isochrones of the world's ocean floor. J.
Geophys. Res., 102, 3211-3214.
-
Nielsen, T.K., Larsen, H.C. &
Hopper, J.R. 2002. Contrasting rifted margin styles
south of Greenland: implications for mantle plume
dynamics. Earth Planet Sci. Lett., 200,
271-286.
-
Nunns, A.G. 1983. Plate tectonic
evolution of the Greenland-Scotland Ridge and surrounding
regions. In: Bott, M.H.P., Saxov, S., Talwani, M.
& Thiede, J. (eds). Structure and Development
of the Greenland-Scotland Ridge: New Methods and
Concepts. Plenum Press, New York, 11-30.
-
Nøhr-Hansen, H., Sheldon,
E. & Dam, G.A. 2002. A new biostratigraphic
scheme for the Paleocene onshore West Greenland
and its implications for the timing of the prevolcanic
evolution. In: Jolley, D.W. & Bell, B.R. (eds).
The North Atlantic Igneous Province, Tectonic,
Volcanic and Magmatic Processes. Geol. Soc.,
London, Special Publications, 197,
111-156.
-
Oakey, G.N. 1994. A structural
fabric defined by topographic lineaments: Correlation
with Tertiary deformation of Ellesmere and Axel
Heiberg Islands, Candian Arctic. J. Geophys.
Res., 99, B10, 20,311-20,321.
-
Oakey, G.N., Roest, W.R. &
Lundin, E.R. 2003. The structure and evolution of
Baffin Bay and its implication on the development
of the continental margins of northwest Greenland,
the Nares Strait, and Baffin Island, Canada. Eos,
Trans. AGU, Dec 8-12, San Francisco.
-
Okulitch, A.V., Dawes, P.R.,
Higgins, A.K., Soper, N.J. & Christie, R.L.
1990. Towards a Nares Strait solution: Structural,
studies on Southeastern Ellesmere Island and Northwestern
Greenland. Marine Geology, 93,
369-384.
-
Planke, S. & Alvestad, E.1999.
Seismic volcanostratigraphy of the extrusive breakup
complexes in the Northeast Atlantic: Implications
from ODP/DSDP drilling. In: Larsen, H.C., Duncan,
R.A., Allan, J.F. & Brooks, K. (eds). Proceedings
of the Ocean Drilling Program, Scientific Results,
163, 3-16.
-
Reid, I. & Jackson, H.R.
1997. Crustal structure of northern Baffin Bay:
seismic refraction results and tectonic implications.
J. Geophys. Res., 102,
523-542.
-
Ritchie, J.D., Gatliff, R.W.
& Richards, P.C. 1999. Early Tertiary magmatism
in the offshore NW UK margin and surrounds. In:
Fleet, A.J. & Boldy, S.A.R. (eds), Petroleum
Geology of Northwest Europe: Proceedings of
the 5th Conference, Geol. Soc., London, 573-584.
-
Roest, W.R. & Srivastava,
S.P. 1989. Sea-floor spreading in the Labrador Sea:
A new reconstruction, Geology, 17,
1000-1003.
-
Saunders, A.D., Fitton, J.G.,
Kerr, A.C., Norry, M.J. & Kent, R.W. 1997. The
North Atlantic Igneous Province. In: Mahoney, J.J.
& Coffin, M.L. (eds). Large Igneous Provinces,
Geophysical Monograph Series, American Geophysical
Union, Washington DC.
-
Smallwood, J.R., Staples, R.K.,
Richardson, R.K. & White, R.S. 1999. Crust generated
above the Iceland mantle plume: From continental
rift to oceanic spreading center. J. Geophys.
Res., 104, 22,885-22,902.
-
Smallwood, J.R. & White,
R.S. 2002. Ridge-plume interaction in the North
Atlantic and its influence on continental breakup
and seafloor spreading. In: Jolley, D.W. & Bell,
B.R. (eds) The North Atlantic Igneous Province:
Stratigraphy, Tectonic, Volcanic and Magmatic Processes.
Geol. Soc., London, Special Publications, 197,
15-37.
-
Smith, W.H.F. & Sandwell,
D.T. 1997. Global seafloor topography from satellite
altimetry and ship depth soundings, Science,
277, 1957-1962.
-
Srivastava, S.P. 1983. Davis
Strait: Structures, origin and evolution. In Bott,
M.H.P., Saxov, S., Talwani, M. & Thiede, J.
(eds) Structure and Development of the Greenland-Scotland
Ridge, Plenum Press, New York, 159-189.
-
Srivastava, S.P. & Roest,
W.R. 1999. Extent of oceanic crust in the Labrador
Sea. Mar. Pet. Geol., 16,
65-84.
-
Steckler, M.S., and ten Brink,
U. 1986, Lithospheric strength variations as a control
on new plate boundaries: examples from the northern
Red Sea region: Earth Planet. Sci. Lett.,
79, p. 20-132.
-
-
Talwani, M. & Eldholm, O.1977.
Evolution of the Norwegian-Greenland Sea, Geol.
Soc. Am. Bull., 88, 969-999.
-
Torsvik, T.H., Mosar, J. &
Eide, E.A. 2001a. Cretaceous-Tertiary geodynamics:
a North Atlantic exercise. Geophys. J. Int.,
146, 850-866.
-
Torsvik, T.H., Van der Voo, R.,
Meert, J.G., Mosar, J. & Walderhaug, H.J. 2001b.
Reconstructions of continents around the North Atlantic
at about the 60th parallel. Earth Planet. Sci.
Lett., 187, 55-69.
-
Verhoef, J., Usow, K.H. &
Roest, W.R. 1990. A new method for plate reconstructions:
the use of gridded data. Computers & Geosciences,
16, 51-74.
-
Verhoef, J., Roest, W.R., Macnab,
R., Arkani-Hamed, J. & Members of the Project
Team. 1996. Magnetic anomalies of the Arctic and
North Atlantic Oceans and adjacent land areas. GSC
Open File 3125, parts a and b (CD-ROM and project
report), Geological Survey of Canada, Dartmouth,
NS.
-
Vink, G.E. 1982. Continental
rifting and the implications for plate tectonic
reconstructions. J. Geophys. Res., 87,
10,677-10,688.
-
Vink, G.E. 1984. A hotspot model
for Iceland and the Vøring Plateau. J.
Geophys. Res., 89, 9949-9959.
-
Vogt, P.R., Johnson, G.L. &
Kristjansson, L. 1980. Morphology and magnetic anomalies
north of Iceland. J. Geophys., 47,
67-80.
-
White, R.S., Spence, G.D., Fowler,
S.R., McKenzie, D.P., Westbrook, G.K. & Bowen,
N. 1987. Magmatism at rifted continental margins.
Nature, 330, 439-444.
-
White, R.S. 1988. A hot-spot
model for Early Tertiary volcanism in the N. Atlantic.
In: Morton, A.C. & Parson, L.M. (eds). Early
Tertiary Volcanism and the Opening of the NE Atlantic.
Geol. Soc., London, Special Publications, 39, 3-13.
-
Ziegler, P-A. 1992. European
rift system. Tectonophysics, 208,
91-111.
|
last
updated 11th June, 2004 |
|
|