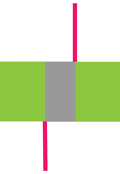 |
The Iceland Microcontinent and a Continental Greenland-Iceland-Faroe Ridge |
Gillian R. Foulger1, Tony Doré1, C. Henry Emeleus1,§, Dieter Franke2, Laurent Geoffroy3, Laurent Gernigon4, Richard Hey5, Robert E. Holdsworth1, Malcolm Hole6, Ármann Höskuldsson7, Bruce R. Julian1, Nick Kusznir8, Fernando Martinez5, Ken J.W. McCaffrey1, James H. Natland9, Alexander L. Peace10, Kenni Petersen11, Christian Schiffer12, Randell Stephenson6 & Martyn Stoker13
1Department of Earth Sciences, Durham University, Science Laboratories, South Rd. DH1 3LE, UK; g.r.foulger@durham.ac.uk ; agdore@gmail.com ; R.E.Holdsworth@durham.ac.uk ; b.r.julian@durham.ac.uk ; k.j.w.mccaffrey@durham.ac.uk
2Bundesanstalt für Geowissenschaften und Rohstoffe (Federal Institute for Geosciences and Natural Resources), Germany; Dieter.Franke@bgr.de
3Université de Bretagne Occidentale, Brest, 29238 Brest, CNRS, UMR 6538, Laboratoire Domaines Océaniques, 29280 Plouzané, France; Laurent.Geoffroy@univ-brest.fr
4Norges Geologiske Undersøkelse (NGU), Geological Survey of Norway, Leiv Erikssons vei 39, N-7491 Trondheim, Norway; Laurent.Gernigon@ngu.no
5Hawaii Institute of Geophysics and Planetology, School of Ocean and Earth Science and Technology, University of Hawaii, Honolulu, HI 96822 USA; hey@soest.hawaii.edu ; fernando@hawaii.edu
6School of Geosciences, Geology and Geophysics, Meston Building, King’s College, University of Aberdeen, Aberdeen AB24 3UE, UK; m.j.hole@abdn.ac.uk ; r.stephenson@abdn.ac.uk
7Háskóli Íslands (University of Iceland), Sturlugötu 7, 101 Reykjavík, Iceland ; armh@hi.is
8School of Environmental Sciences, University of Liverpool, Jane Herdman Building, Liverpool L69 3GP, UK; n.kusznir@liverpool.ac.uk
9Rosenstiel School of Marine and Atmospheric Science, University of Miami, Miami FL 33149, USA; jnatland@msn.com
10School of Geography and Earth Sciences, McMaster University, 1280 Main Street West, Hamilton, Ontario, Canada L8S 4K1; peacea2@mcmaster.ca
11Department of Geoscience, Aarhus University, Høegh-Guldbergs Gade 2, DK-8000 Aarhus C., Denmark; kenni@geo.au.dk
12Department of Earth Sciences, Uppsala University, Villavägen 16, 75236 Uppsala, Sweden; christian.schiffer@geo.uu.se
13Australian School of Petroleum, University of Adelaide, Adelaide, South Australia 5005 Australia; martyn.stoker@gmail.com
§deceased
This webpage summarizes parts of: Foulger, G. R., T. Doré, C. H. Emeleus, D. Franke, L. Geoffroy, L. Gernigon, R. Hey, R. E. Holdsworth, M. Hole, Á. Höskuldsson, B. Julian, N. Kusznir, F. Martinez, J. H. Natland, A. L. Peace, K. Petersen, C. Schiffer, R. Stephenson, and M. Stoker (2019), The Iceland Microcontinent and a continental Greenland-Iceland-Faroe Ridge, Earth-Science Reviews, in press.
Introduction
Although the Greenland-Iceland-Faroe Ridge (GIFR) and surrounding areas were centrally involved in development of the Plate Tectonic hypothesis, e.g., with the discovery of symmetric magnetic anomalies across the Reykjanes Ridge, over the subsequent years many observations from the region were never satisfactorily explained by this hypothesis (Figure 1). The most striking of these is the very existence of the GIFR, which formed a subaerial landbridge connecting Greenland to Europe until the North Atlantic Ocean was ~1000 km wide. Such a feature was, capriciously, predicted by pre-Plate Tectonic "fixism" theory, but not by Plate Tectonic "mobilism".
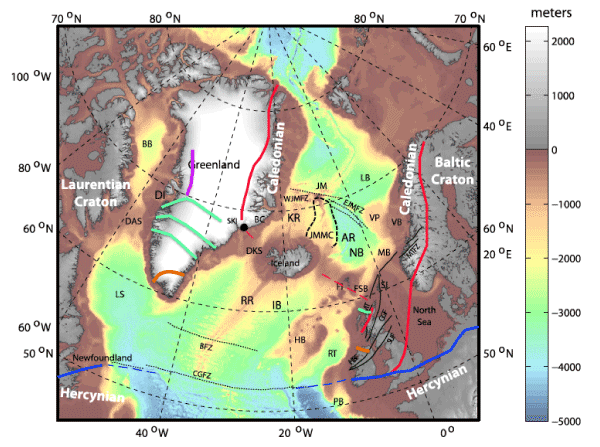
Figure 1: Regional map of the Northeast Atlantic Realm. Bathymetry is shown in color and topography in land areas in gray. DAS: Davis Strait, CGFZ: Charlie-Gibbs Fracture Zone, RR: Reykjanes Ridge, KR: Kolbeinsey Ridge, JMMC: Jan Mayen Microcontinent Complex, AR: Aegir Ridge, FI: Faroe Islands, Red lines: boundaries of the Caledonian orogen and associated thrusts, blue lines: northern boundary of the Hercynian orogen, both dashed where extrapolated into the younger Atlantic Ocean. Green lines: Nagssugtoqidian orogenic belt, magenta line: Rinkian orogeny, orange line: Ketilidian orogeny.
Following the near-universal acceptance of Plate Tectonics, the existence of this peculiar landbridge was simply attributed to ad hoc excess volcanism. This attribution did not go on to become a natural component of a holistic, successfully forward-predictive theory, however. It eventually became just one of many ad hoc attributions introduced to account for unusual, non-compliant observations in the region. These include the following.
- The GIFR crust has an extraordinary 30-40-km thickness, as measured using seismology [see Foulger et al., 2003 for a review].
- The 20-30-km-thick Icelandic lower crust has a seismic velocity VP of 7.0-7.3 km/s and a density of ~3150 kg/m3. These physical properties do not correspond to any reasonable basaltic or peridotic petrology [Gudmundsson, 2003; Menke, 1999].
- Refracted Moho seismic phases are extremely rare in Iceland.
- Mantle melting models are unable to explain the 30-40-km GIFR crustal thickness, given the moderate mantle source temperatures obtained by independent petrological and geophysical methods [Clarke & Beutel, 2019; Foulger et al., 2005, Figure 7; Hole & Natland, 2019; Ribe et al., 1995; Shulgin & Artemieva, 2019].
- Icelandic rocks have a clear continental petrological and geochemical signature. These are shown by high-TiO2, FeO(T) and rare-earth element signatures, voluminous felsic and intermediate rocks (10% of Icelandic lavas are intermediate or felsic), elevated 87Sr/86Sr and Pb isotope ratios, water contents as high as 920 ppm beneath Iceland, and Proterozoic and Mesozoic zircons [Natland, 2007; Foulger, 2006].
- Where the mid-Atlantic Ridge extensional plate boundary crosses the GIFR, extension, shearing and volcanism are distributed throughout a zone ~375 km wide from east to west, ~30% of the length of the GIFR. This contrasts starkly with the narrowness of the tectonically active submarine plate boundary (Figure 2).
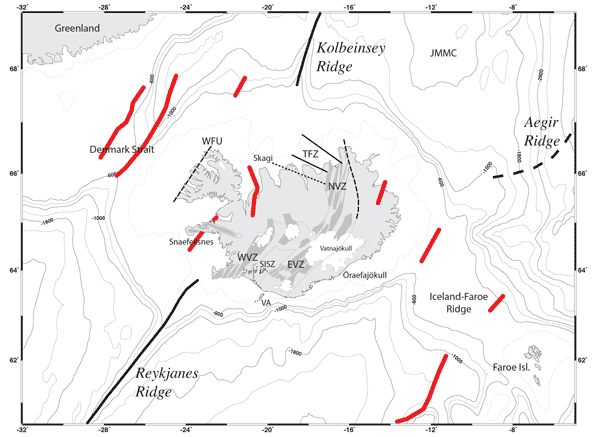
Figure 2: The Greenland-Iceland-Faroe Ridge (GIFR) and surrounding areas showing bathymetry and tectonic features. JMMC: Jan Mayen Microcontinent Complex. Thick black lines: axes of Reykjanes and Kolbeinsey Ridges, thin gray lines on land: outlines of neovolcanic zones, dark gray: currently active extensional volcanic systems, dashed black lines: extinct rifts on land, thin black lines: individual faults of the South Iceland Seismic Zone (SISZ), white: glaciers. WVZ, EVZ, NVZ: Western, Eastern, Northern Volcanic Zones, TFZ: Tjörnes Fracture Zone comprising two main shear zones and one (dotted) known only from earthquake epicenters. Thick red lines: extinct rift zones from Hjartarson et al. (2017).
Explaining individual observations that do not readily fit an existing theory with ad hoc auxiliary hypotheses that do not address the fundamental theoretical core and can be arbitrarily changed when new information is acquired (e.g., the migration path of a mantle plume), is not scientifically progressive. A model that can account for all the data from the NE Atlantic Realm in a self-consistent way has long been required. Not only should such a model account for existing observations, it should also be forward predictive and testable. We attempt such a model here.
We propose that the key to the long-standing puzzles associated with the GIFR is that a large proportion of its deeper crust is continental [Foulger et al., 2019]. Specifically, the 20-30-km-thick lower crust is magma-dilated continental mid- and lower crust and the 5-10-km-thick upper crust forms an igneous cap. This "mixed lithology" theory for the composition of the GIFR crust contrasts with earlier theories:
- Prior to the early 1990s: The "thin crust" model postulated that only the 5-10-km-thick upper GIFR crust was magmatic and that the material beneath it was hot, peridotic mantle [e.g., Gebrande et al., 1980].
- After the early 1990s: The "thick crust" model was generally adopted. This postulated that the crust was 30-40 km thick and that this layer was entirely magmatic, i.e. thick oceanic crust [e.g., Bjarnason et al., 1993].
Neither of these earlier theories could explain the observations listed above, but a mixed lithology for the lower crust can, as we describe below.
Continental breakup forming the Northeast Atlantic Realm
In order to explain how continental material could span a 1,200-km-wide ocean, the history of continental breakup must first be briefly summarized. Opening of the Northeast Atlantic Ocean in the early Cenozoic was not a simple, abrupt, isolated event. It was the culmination of a >300 Myr period of episodic rifting that lasted from the Late Palaeozoic through the Mesozoic [Schiffer et al., 2019]. Starting in the Paleocene, it disintegrated an inhomogeneous collage of cratons sutured by cross-cutting orogens (Figure 1). This lithospheric inhomogeneity influenced to a first-order the pattern of breakup, guiding where, whether, and how it occurred.
The Northeast Atlantic Ocean began to form at ~56-52 Ma when a new arm of the mid-Atlantic ridge propagated, from the (now) Charlie-Gibbs Fracture Zone, northward through the North American Craton, splitting south Greenland from Europe (Figure 3). This propagation stalled at the confluence of the ~1.8 Ga Nagssugtoqidian orogen and the western frontal thrust of the ~ 400 Ma Caledonian orogeny (Figure 1).
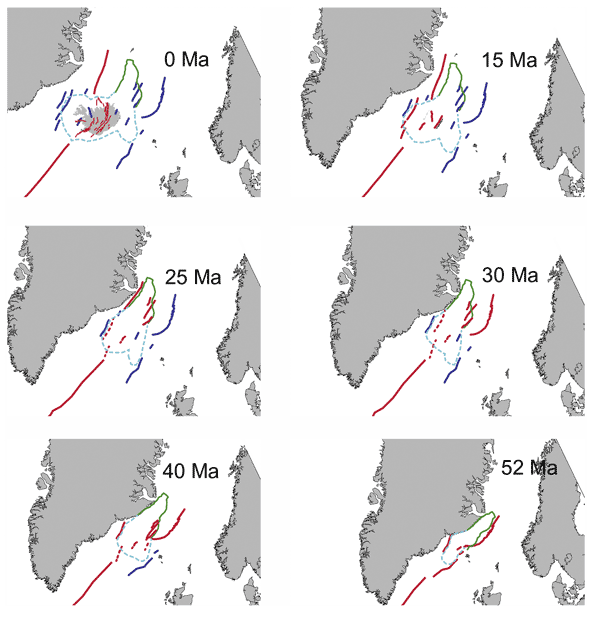
Figure 3: Speculative reconstruction of the sequence of extensional deformation on the GIFR and surroundings. Outline of land areas and locations of known extensional axes are from Figure 2 with the latter shown as solid lines. Red: active, blue: extinct. Dashed lines show speculative positions of ridges at times when observational data are lacking. Green solid line: approximate boundary of Jan Mayen Microplate Complex. Pale blue dashed line: approximate boundary of Iceland Microcontinent. This, and the Jan Mayen Microplate Complex, expand with time as a result of magma inflation and ductile flow, which also occurs from beneath the flanking continental areas.
In the same time frame (54-52 Ma) a spreading ridge formed further to the north–the Aegir Ridge–and propagated south [Gernigon et al., 2019]. This ridge formed ~100-150 km east of the Reykjanes Ridge, and propagation also stalled at the confluence of the Nagssugtoqidian and Caledonian orogenies. Because the orogenic confluence zone is ~300 km wide, the two stalled rift tips remained widely separated.
This piecemeal beginning of breakup trapped a block of continental crust ~300 km long in the NS direction and ~100-150 km wide in the EW direction. We call this ~30,000-45,000 km2 block the Iceland Microcontinent (Figures 3 & 4). As the ocean widened, the Iceland Microcontinent and flanking continental areas extended, by ductile flow and magma inflation, in a distributed, unstable manner along multiple axes. These axes repeatedly migrated or jumped laterally with deformation between them taken up in diffuse shear zones. The presence of the Iceland Microcontinent is likely a key contributory factor to the development of the exceptionally wide GIFR which may, to a first approximation, be likened to two pairs of passive volcanic margins–one to the west and one to the east of the Iceland Microcontinent [Geoffroy et al., 2015].
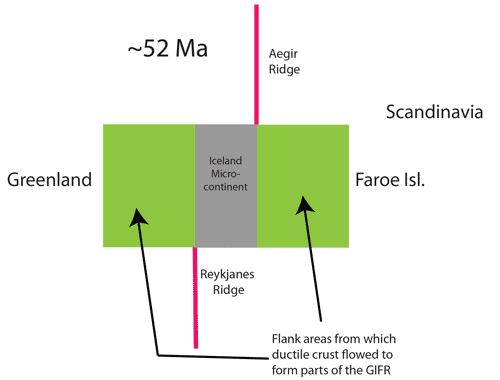
Figure 4: Schematic diagram illustrating the Iceland Microcontinent.
This style of extension continues to the present day in Iceland which should be viewed not as an isolated, anomalous island, but as the 40% of the GIFR that is still volcanically active and standing above sea level. In Iceland extension occurs along several, parallel rift zones, the main ones being the Reykjanes Peninsula Zone and the Western, Eastern, Middle and Northern Volcanic Zones (Figure 2). Between them deformation is taken up mostly by shear in complex fracture zones—the South Iceland Seismic Zone and the Tjörnes Fracture Zone (Figure 2). Where these are ephemeral they fail to develop clear topographic signatures.
There is evidence from offshore areas that this style of extension characterized the GIFR for its entire ~52-Myr history. Numerous northerly trending synclines, likely extinct extensional zones, have been mapped on submarine parts of the GIFR [Hjartarson et al., 2017] (Figure 2).
Thermo-mechanical modeling
We tested the plausibility of unusually prolonged survival of intact continental crust beneath the GIFR with two-dimensional thermo-mechanical modeling. We explored the visco-elastic-plastic response of an ancient orogen under simple extension [Petersen & Schiffer, 2016]. For the structure of the confluence of the Nagssugtoqidian and Caledonian orogenies we assumed a Himalaya-type stack of multiple terranes, entrained subduction zones, and continental material underlain by fossil slabs trapped in the lithosphere. We likened this to a 50-km-thick crust underlain by an additional 20-km-thick slab of high-velocity lower crust with an assumed mafic composition. For the lithosphere adjacent to the orogenic confluence we assumed a pre-rift continental crustal thickness of 40 km.
Modeling showed that stretching at the onset of breakup destabilizes the lithospheric mantle and the negatively buoyant high-velocity lower crust starts to delaminate after ~12 Myr. As a result, asthenosphere with a potential temperature of ~1325°C and crust with an initial temperature of ~600-800°C are rapidly juxtaposed. This increases heat flow in the crust, keeping it ductile so it continues to extend in the delocalized, “wide rift mode” of Buck [1991]. The lower crust beneath Iceland is known from post-diking stress relaxation and glacial rebound to have a viscosity of ~ 1019 Pa s [Foulger et al., 1992; Heki et al., 1993; Sigmundsson, 1991]. The loci of extension repeatedly migrate laterally and this mode of deformation continues as long as lower crust is available. Full lithosphere breakup has not yet occurred by 51 Myr (Figure 5)—the loci of extension only stabilize after ~70 Myr.
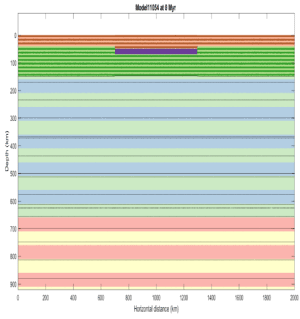
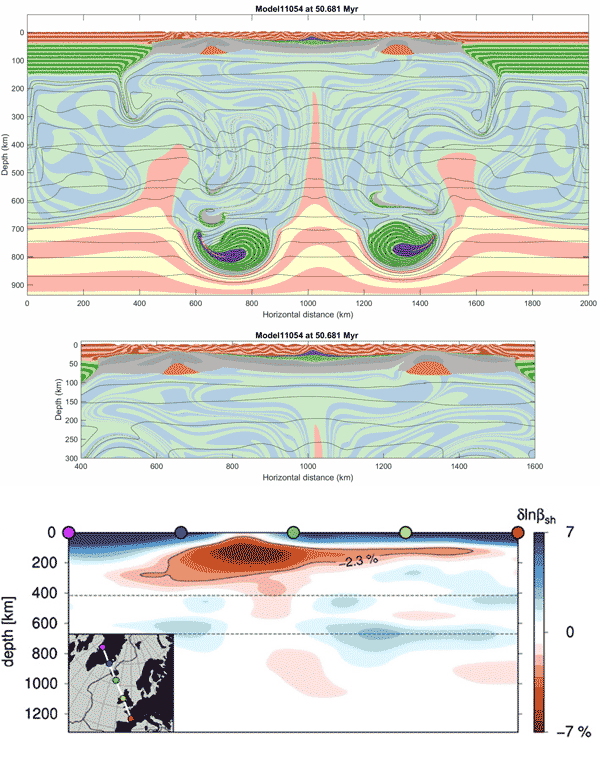
Figure 5 : Results of thermo-mechanical model ~51 Myr after the onset of extension. Upper panel: Starting model, Second panel: Lithology. Third panel: Expanded view of top 300 km. Lower panel: Cross section through the full-waveform inversion tomographic model of Rickers et al. (2013).
The present-day structure predicted by this model compares well with the full-waveform inversion tomographic model of Rickers et al. [2013] (Figure 5, lower panel). Features in close correspondence to those we predict include the high-wave-speed bodies at the bottom of the transition zone, a narrow, weak, vertical, low-wave-speed body between them and a broader, stronger, low-wave-speed body in the top ~200 km underlying the entire ocean.
Paradoxes explained
This new model can account for the many observations from the GIFR and surrounding regions that have hitherto been paradoxical:
- The high stand and great crustal thickness of the GIFR are explained as the crustal structure and composition we propose is similar to the Inner-SDRs of the Northeast Atlantic that support the shallow continental shelves. The exceptional width of the GIFR is partly explained by the capture of the Iceland Microcontinent between the propagating Reykjanes and Aegir Ridge tips and partly by the thick, fertile orogenic crustal structure and fabric.
- The crust-like seismic velocities coupled with densities approaching those of the mantle, that cannot be explained by any single lithology, can be explained by a mixture of young igneous intrusions and continental mid- and lower crust.
- The issue of a 30-40 km thick melt layer that cannot be accounted for by mantle melting models immediately becomes moot.
- The continental petrological and geochemical signature, and abundant intermediate and felsic rocks, are readily accounted for as MORB melts rise through and assimilate continental crust before reaching the surface.
- The contrast in deformation and extension in Iceland compared with marine sea-floor spreading is accounted for as GIFR deformation and extension style is intermediate between continental and oceanic.
Explanations are also immediately obvious for several other unusual features. It is clear why, despite many lateral rift jumps, the plate boundary north of the GIFR has failed to connect with the Reykjanes Ridge to the south to form a classical ridge-transform plate boundary. The decorrelation of oceanic tectonic history north and south of the GIFR is a natural consequence of decoupling of the two areas by the independently deforming GIFR. Instability on the Reykjanes, Aegir and Kolbeinsey Ridges close to Iceland is understandable because they are destabilized by the persistent disequilibrium on the adjacent GIFR. To the north, early distributed extension throughout the southern Jan Mayan Microplate Complex and the south Aegir Ridge gave way to sea-floor spreading focused on the Kolbeinsey Ridge. To the south, the Reykjanes Ridge underwent two major reorganizations, the last of which is currently in progress and associated with growth of diachronous "chevron ridges" of thickened oceanic crust. The onsets of rift propagators, with which these chevron ridges are associated, at the north end of the Reykjanes Ridge close to Iceland, are synchronized with major rift migrations in Iceland [ Martinez et al., 2019].
Generic implications
The model we describe above suggests that the continental breakup that formed the Northeast Atlantic Ocean was chaotic, strongly influenced by pre-existing structures, and engineered by propagating rifts and transtensional reactivation of old structures. Frequent migrations of axes of extension and ductile flow of the deeper continental crust distributed continental material widely in the new ocean. Much of this is capped by several kilometers of igneous rocks, including regions overlain by Inner-SDRs, the GIFR, parts of the Jan Mayen Microplate Complex and the Faroe Platform. Tectonic disequilibrium is reflected in frequent rift migrations, ephemeral shear deformation zones, and volcanism distant from the dominant spreading ridge.
Such a model is applicable to other regions. The neaby Davis Strait resembles a small version of the GIFR. Analogous features are found in the South Atlantic Igneous Province, the Seychelles, Madagascar and Kerguelen regions in the Indian Ocean, and the Galapagos region in the Pacific Ocean [Foulger, 2010, p 100-101; Peace et al., 2019]. At subduction zones, buoyant continental fragments are expected to accrete to the overriding plate. The GIFR is actively demonstrating today the tectonic processes that build volcanic passive margins. The possibility that continental, or mixed-lithology crust may be more widespread in the oceans than hitherto thought has intriguing implications.
References
-
Bjarnason, I. T., W. Menke, O. G. Flovenz, and D. Caress (1993), Tomographic image of the mid-Atlantic plate boundary in south-western Iceland, J. Geophys. Res., 98, 6607-6622.
-
Buck, W. R. (1991), Modes of continental lithospheric extension, J. Geophys. Res., 96, 20161-20178.
-
-
-
-
-
-
Foulger, G. R., C. H. Jahn, G. Seeber, P. Einarsson, B. R. Julian, and K. Heki (1992), Post-rifting stress relaxation at the divergent plate boundary in Northeast Iceland, Nature, 358, 488-490.
-
Foulger, G. R., T. Doré, C. H. Emeleus, D. Franke, L. Geoffroy, L. Gernigon, R. Hey, R. E. Holdsworth, M. Hole, Á. Höskuldsson, B. Julian, N. Kusznir, F. Martinez, J. H. Natland, A. L. Peace, K. Petersen, C. Schiffer, R. Stephenson, and M. Stoker (2019), The Iceland Microcontinent and a continental Greenland-Iceland-Faroe Ridge, Earth-Science Reviews, in press.
-
Gebrande, H., H. Miller, and P. Einarsson (1980), Seismic structure of Iceland along RRISP-Profile I, J. Geophys., 47, 239-249.
-
-
Gernigon, L., D. Franke, L. Geoffroy, C. Schiffer, G. R. Foulger, and M. Stoker (2019), Crustal fragmentation, magmatism, and the diachronous opening of the Norwegian-Greenland Sea, Earth-Science Reviews, in press.
-
Gudmundsson, O. (2003), The dense root of the Iceland crust, Earth planet. Sci. Lett., 206, 427-440.
-
-
Hjartarson, A., O. Erlendsson, and A. Blischke (2017), The Greenland–Iceland–Faroe Ridge Complex, in The NE Atlantic Region: A Reappraisal of Crustal Structure, Tectonostratigraphy and Magmatic Evolution, edited by G. Peron-Pinvidic, J. R. Hopper, M. S. Stoker, C. Gaina, J. C. Doornenbal, T. Funck and U. E. Arting, pp. 127-148, Geological Society, London, Special Publications.
-
-
-
Menke, W. (1999), Crustal isostasy indicates anomalous densities beneath Iceland, Geophys. Res. Lett., 26, 1215-1218.
-
-
Peace, A. L., D. Franke, A. Doré, G. R. Foulger, N. Kusznir, J. G. McHone, J. Phethean, S. Rocchi, S. Schiffer, M. Schnabel, and J. K. Welford (2019), A review of Pangaea dispersal and Large Igneous Provinces – In search of a causative mechanism, Earth-Science Reviews, in press.
-
-
Ribe, N. M., U. R. Christensen, and J. Theissing (1995), The dynamics of plume-ridge interaction, 1: Ridge-centered plumes, Earth planet. Sci. Lett., 134, 155-168.
-
Rickers, F., A. Fichtner, and J. Trampert (2013), The Iceland–Jan Mayen plume system and its impact on mantle dynamics in the North Atlantic region: Evidence from full-waveform inversion, Earth planet. Sci. Lett., 367, 39-51.
-
Schiffer, C., A. G. Doré, G. R. Foulger, D. Franke, L. Geoffroy, L. Gernigon, B. Holdsworth, N. Kusznir, E. Lundin, K. McCaffrey, A. Peace, K. D. Petersen, R. Stephenson, M. S. Stoker, and K. Welford (2019), Structural inheritance in the North Atlantic, Earth-Science Reviews, in press.
-
Shulgin, A., and I. M. Artemieva (2019), Thermochemical Heterogeneity and Density of Continental and Oceanic Upper Mantle in the European-North Atlantic Region, J. Geophys. Res., 124, 9280-9312.
-
Sigmundsson, F. (1991), Post-glacial rebound and asthenosphere viscosity in Iceland, Geophys. Res. Lett., 18, 1131-1134.
last updated 30th
November, 2019 |