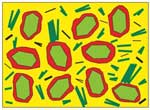 |
Sr-enriched glassy picrites from the Karoo Large Igneous Province are evolved, not primitive magmatic rocks |
L. D. Ashwal1, A. Ziegler2, S. Glynn1,3, T. Truebody1,4 & R. Bolhar1
1School of Geosciences, University of the Witwatersrand, Private Bag 3, WITS 2050, Johannesburg, South Africa; lewis.ashwal@wits.ac.za ; sarah.glynn@wits.ac.za ; tristan.truebody68@gmail.com ; robert.bolhar@wits.ac.za
2Microscopy and Microanalysis Unit, University of the Witwatersrand, Private Bag 3, WITS 2050, Johannesburg, South Africa; alexander.ziegler@wits.ac.za
3GFZ German Research Centre for Geosciences, Telegrafenberg, 14473 Potsdam, Germany
4Amatshe Mining, Johannesburg, South Africa
This webpage is a summary of: Ashwal, L.D., Ziegler, A., Glynn, S., Truebody, T. and Bolhar, R., 2021. Sr-enriched glassy picrites from the Karoo Large Igneous Province are evolved, not primitive magmatic rocks. Geochemistry, Geophysics, Geosystems, e2020GC009561.
Large Igneous Provinces (LIPs) typically consist of a volumetrically dominant, compositionally uniform low-Ti tholeiitic suite, and a subordinate, geographically restricted, compositionally diverse, incompatible-rich high-Ti suite, as first recognized by Cox (1967) for the Karoo LIP. This can be illustrated using our compilation of nearly 8000 Karoo rock analyses (Figure 1), which shows that the LILE-enriched high-Ti suite of magmatic rocks is restricted mainly to the arms of three intersecting dike swarms and linear belts of lavas (Figure 2). An intriguing feature is that the high-Ti suite of Karoo mafic extrusive and intrusive rocks includes picrites (defined as having >12 wt. % MgO) with remarkable enrichments in LILE: up to 1900 ppm Ba, 2400 ppm Sr, 550 ppm Zr, 3800 ppm P, and 4.8 wt. % each of K2O, and TiO2 (Figure 2), which seems perplexing for such olivine-rich lithologies that are commonly perceived as some of the most primitive of melts in the Karoo LIP (e.g., Harris et al., 2015).
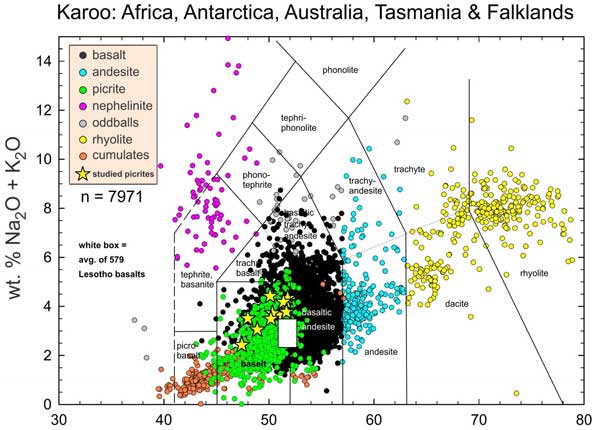
Figure 1. Total alkalis vs. silica (TAS) diagram showing compilation of 7971 whole rock major element analyses of Karoo magmatic rocks from South Africa, Lesotho, Eswatini (formerly Swaziland), Zimbabwe, Botswana, Mozambique, Namibia, Antarctica, Australia, Tasmania and the Falkland Islands. Data sources: Duncan et al. (1984) database (n = 4251), GEOROC database (n = 1868), published papers (n = 1575), unpublished data of J.S. Marsh (n = 277). The vast volumetric majority of Karoo mafic rocks are low-Ti tholeiites, which almost entirely plot inside the white box, representing the average of 579 Lesotho basalts (1σ standard deviation). We have excluded the low-Ti Karoo sills and dykes from our average because these intrusives cooled more slowly than the lavas and may have experienced some mineral accumulation. In contrast, the high-Ti rock suite is extremely compositionally variable, but has been over-sampled and over-analysed, out of proportion to its volume. Compositions of the six high-Ti alkali picrites we studied (yellow stars, some samples were re-analyzed) exceed the chemical variability of the low-Ti suite of tholeiitic basalts. Karoo picritic rocks (green symbols), defined as having >12 wt. % MgO, are themselves compositionally variable, with Na2O + K2O up to 5.4 wt. %. Note that a large number of basaltic rocks, defined as having SiO2 = 45-57 wt. % and MgO <10 wt. % (black symbols), plot underneath the symbols for picrites, which are highlighted in this diagram. Samples designated as “oddballs” include shoshonite, nordmarkite and lamprophyre. Click here or on Figure for enlargement.
We obtained detailed mineralogical, geochemical and textural measurements and observations for six Karoo picrites aimed at:
- determining the phase(s) hosting the LILE,
- characterizing the compositionally diverse interstitial glasses, and
- constraining the magmatic evolution and petrogenesis of these rocks.
Our samples show the following compositional ranges:
- MgO = 11.5 – 21.3 wt. %,
- TiO2 = 2.6 – 4.0 wt. %,
- Sr = 826 – 1536 ppm,
- Ba = 667 – 1320 ppm, and
- Zr = 302 – 587 ppm.
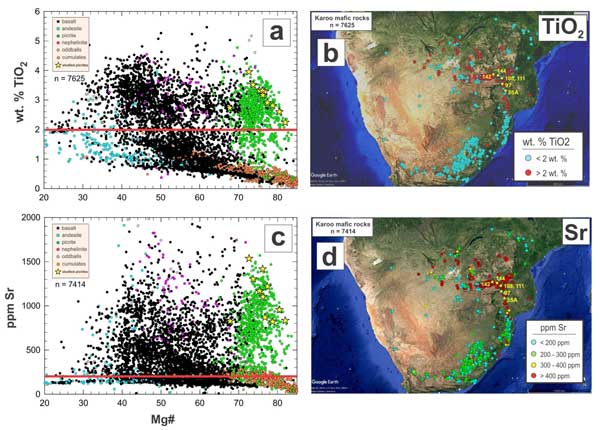
Figure 2. Left panel: Plots of Mg# vs wt. % TiO2 (a) and ppm Sr (c) for Karoo mafic rocks (felsic rocks such as rhyolite and dacite are omitted). Compositions of the six alkali picrites studied in this paper are shown as yellow stars (some samples were re-analyzed). Horizontal red lines separate the high-Ti and low-Ti rock suites, commonly chosen at TiO2 = 2 wt. % and Sr = 200 ppm. Right panel: Google Earth images of southern Africa showing locations of Karoo mafic rocks color-coded according to wt. % TiO2 (b) and ppm Sr (d). Locations of our samples, with sample numbers, are shown as yellow stars. High-Ti suite rocks are mainly concentrated in the arms of three intersecting dike swarms and linear belts of lavas in the Lebombo-Mwenezi, Sabi and Tuli regions. Click here or on Figure for enlargement.
The chemical variability of these six samples (yellow stars in Figures 1 and 2) exceeds that of the 579 Lesotho tholeiitic basalts constituting the dominant volume of Karoo magmas (white box in Figure 1). The picrites consist of 10 – 30 % euhedral to subhedral olivine phenocrysts and glomerocrysts in a groundmass of brown glass (0 – 44 modal %), augite (18 – 33 %), feldspar (0 – 39 %), ilmenite (2 – 8 %) and apatite (0.5 – 1 %). Vesicles and amygdales are absent. Olivines (cores: Fo82-87) have been variably serpentinized (5 – 18 %) along irregular fractures and phenocryst rims up to 250 mm across. Secondary alteration products are completely absent in all groundmass phases, including the glasses.
Some samples have the unusual feature of containing two compositionally distinct glasses, which are distinguishable optically, in BSE images and in X-ray maps. The dominant glass in the groundmass of these samples (65 – 90 % of total glass) is lighter brown in color, has a lighter gray BSE response, and is designated as Type 1 glass. The darker brown glass, with darker gray BSE color, is lesser in volume (9 – 35% of total glass), mainly occurs adjacent to olivine phenocrysts, and is designated as Type 2 glass. Boundaries between the two glasses are sharp, but irregular. Glass compositions vary, but are generally evolved, ranging from basaltic trachyandesite to dacite. Most are very rich in alkalies (~2 – 13 wt. % Na2O + K2O). SIMS analyses show that both glasses are rich in H2O (up to 3.8 wt. %) but are nearly devoid of CO2. Minor volumes of Type 2 glass adjacent to olivine phenocrysts have distinctly lower K2O, MgO and FeO, higher H2O, and much higher Na2O and Sr, relative to the dominant Type 1 glass phases in the groundmasses. The glasses are highly enriched in LILE, with Sr = 3000 – 3400 ppm, Ba = 3000 – 3300 ppm, Zr = 1000 – 1200 and Ce = 400 – 450 ppm. Type 2 glass adjacent to olivine has extraordinarily high Sr (up to 9500 ppm).
The picrites we studied do not represent liquid compositions per se, and are best interpreted as mixtures of variable amounts of accumulated olivine phenocrysts and evolved interstitial melts, represented by bulk groundmass compositions. Parental liquid compositions may be estimated as about 50% each of bulk-rock compositions and bulk groundmass compositions. Average calculated parental melts have basalt-like SiO2 (50.8 ± 0.5 wt. %), but lower Al2O3 (9.5 ± 0.6 wt. %) and Na2O (1.6 ± 0.8 wt. %), higher MgO (9.3 ± 0.5 wt. %), and much higher K2O (2.9 ± 1.3 wt. %) relative to the average Lesotho tholeiite (Figure 1). Most trace elements, including all incompatible elements (avg. Sr = 1290 ± 329 ppm, Ba = 1217 ± 314 ppm, Zr = 493 ± 98 ppm) and even some compatible elements (avg. Ni = 520 ± 152 ppm, Cr = 551 ± 214 ppm, Co = 87 ± 25 ppm) are much higher than those of average Lesotho tholeiite. Fractional crystallization modelling of parent melts yields a crystallization sequence of olivine – clinopyroxene ± Fe-rich spinel ± ilmenite – feldspar, although most picrites quenched to form glass before the onset of feldspar crystallization. Liquid evolution trajectories match glass compositions for most major and trace elements.
The physical and chemical evolution of the picrites is best understood in terms of an open-system magma hybridization petrogenetic model. Variably accumulated olivine phenocrysts surrounded by evolved Sr-rich (to 9470 ppm) Type 2 melts formed by extensive olivine fractionation were infiltrated by chemically distinct Type 1 melts. Upon eruption, Type 2 melt quenched to minor glass around olivine phenocrysts, and the dominant Type 1 glass acquired its evolved composition by quench-crystallization of groundmass mineral phases (Figure 3). Our glassy picrite samples may thus represent unique examples of the magmatic hybridization processes that have often been proposed based on isotopic relationships (e.g., Ellam et al., 1992).
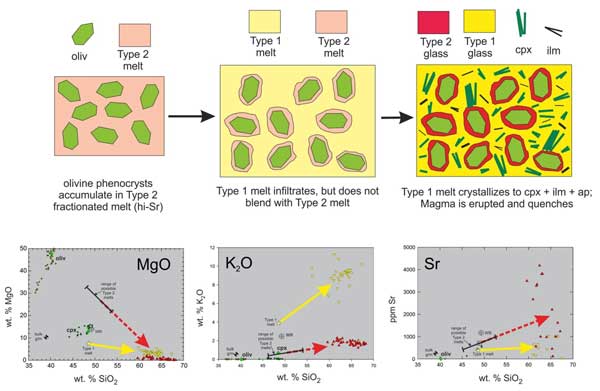
Figure 3. Top panel shows schematic diagrams illustrating open-system petrogenetic model based on sample K-111, which contains two distinct glasses. An accumulation of olivine phenocrysts surrounded by Sr-rich Type 2 melt formed by extensive fractional crystallization (left) is infiltrated by, but does not blend with, genetically unrelated Type 1 melt (center). Upon eruption (right), Type 2 melt quenches to Type 2 glass around olivine phenocrysts, and Type 1 melt quench-crystallizes to clinopyroxene + ilmenite + apatite, and residual Type 1 melt quenches to Type 1 glass. Bottom panel shows plots of wt. % SiO2 vs MgO, K2O and Sr, illustrating liquid evolution paths for Type 2 melt by olivine removal (red arrows) and Type 1 melt by quench crystallization of bulk groundmass minerals (yellow arrows). Note that the compositions of Type 2 melts cannot be constrained precisely because the amount of prior olivine fractionation is not known and are shown as a range of possible compositions. Click here or on Figure for enlargement.
Parental liquids of the glassy alkali picrites are extraordinarily enriched in LILE, with values far higher than almost all typical continental crustal rock types. The enrichment, therefore, must be an inherent property of these melts, with or without crustal contamination, a conclusion supported by isotopic data (Ellam et al., 1992; Harris et al., 2015). We concur with those authors (e.g. Jourdan et al., 2007) who conclude that the low-volume, high-Ti Karoo magmatic rocks, including the alkali picrites in this study, were sourced mainly or entirely from the SCLM. But the bulk of the SCLM below the Kaapvaal Craton must be depleted, cold, buoyant, stable and infertile, having been formed by massive extraction of basaltic and komatiitic magmas during the early- to mid-Archean. Therefore, if the incompatible-rich, high-Ti Karoo magmas were derived from SCLM, their sources must be relatively small-volume enriched domains, representing either ancient subducted crustal materials (e.g. oceanic crust) or local regions metasomatized by fluids or melts. In contrast, Ashwal (2021) argued on the basis of volume constraints that the enormous quantity of low-Ti Karoo tholeiites cannot have been sourced from the SCLM, but instead were generated from sub-lithospheric (i.e. plume) sources.
The enrichments in MgO, incompatible elements and volatile components in the picrites we studied, their parental melts and mantle sources are suggestive of a possible link with other small-volume magmatic rocks, such as kimberlites, lamproites and carbonatites. A direct petrogenetic relationship between these magmatic rocks and the alkali picrites is uncertain, although their mantle sources may share some properties such as enrichment in alkalies, incompatible elements, MgO, TiO2 and volatiles. An important difference is that CO2 is an important component in kimberlites, lamproites and carbonatites, whereas our results show that it is nearly absent in the picrites we studied. In any case, the Karoo glassy picrites should not be considered as inherently primitive magmatic rocks.
References
- Ashwal, L.D. (2021). Sub-lithospheric mantle sources for overlapping southern African Large Igneous Provinces. South African Journal of Geology, special volume in honour of Maarten de Wit, in press.
- Cox, K.G., Macdonald, R. and Horning, G. (1967). Geochemical and petrographic provinces in the Karroo basalts of southern Africa. Amer. Mineral., 52, 1451-1474.
- Ellam, R.M., Carlson, R.W. and Shirey, S.B. (1992). Evidence from Re-Os isotopes for plume-lithosphere mixing in Karoo flood basalt genesis. Nature, 359, 718-720.
- Harris, C., le Roux, P., Cochrane, R., Martin, L., Duncan, A.R., Marsh, J.S., le Roex, A.P. and Class, C. (2015). The oxygen isotope composition of Karoo and Etendeka picrites: high δ18O mantle or crustal contamination? Contrib. Mineral. Petrol., 170:8, doi: 10.1007/s00410-015-1164-1
- Jourdan, F., Bertrand, H., Schärer, U., Blichert-Toft, J., Féraud, G. and Kampunzu, A.B. (2007). Major and trace element and Sr, Nd, Hf, and Pb isotope compositions of the Karoo Large Igneous Province, Botswana-Zimbabwe: lithosphere vs mantle plume contribution. Journal of Petrology, 48, 1043-1077.
last updated 13th April, 2021 |