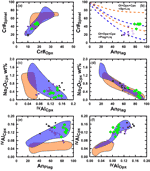 |
Experimental quantification of P-T conditions
of mantle refertilisation at shallow depth
under spreading ridges
and formation of plagioclase + spinel lherzolite
|
Françoise Chalot-Prat1, Trevor J. Falloon2, David H. Green2,
& William O. Hibberson3
1CRPG-CNRS - Université de Lorraine, 15 rue Notre Dame des Pauvres, BP20, 54501 Vandœuvre les Nancy, France chalot@crpg.cnrs-nancy.fr
2Arc Centre of Excellence in Ore Deposits and School of Earth Sciences, University of Tasmania, Private Bag 79, Hobart, Tasmania 7001, Australia, trevor.falloon@utas.edu.au ; David.H.Green@utas.edu.au
3Research School of Earth Sciences, The Australian National University, Canberra, Act0200, Australia
This webpage is a summary of:
Chalot-Prat, Françoise, Trevor J. Falloon, David H. Green & William O. Hibberson, An experimental study of liquid compositions in equilibrium with plagioclase+spinel lherzolite at low pressures (0·75GPa), J. Pet., 51, 11, 2349-2376, 2010;
Chalot-Prat, Françoise, Trevor J. Falloon, David H. Green, William O. Hibberson, Melting of plagioclase + spinel lherzolite at low pressures (0.5 GPa): an experimental approach to the evolution of basaltic melt during mantle refertilisation at shallow depths, Lithos, 172–173, 61–80, 2013.
We studied the first-order melting process of differentiation in the Earth, and the major process of rejuvenation of the upper mantle after melting related to plate spreading.
We conducted experiments at high pressure (0.75 and 0.5 GPa) and high temperature (1260 - 1100°C) to obtain magma compositions in equilibrium with the mineral assemblages of a plagioclase + spinel lherzolite. These PT conditions prevail at 17 - 30 km below axial oceanic spreading ridges. We used a “trial and error” approach, in a complex system involving nine elements: Cr + Na + Fe + Ca + Mg + Al + Si + Ti + Ni. This approaches as closely as possible a natural mantle composition, Cr being a key element in the system.
Our objectives were:
- to determine experimentally the compositions of melts in equilibrium with plagioclase+spinel lherzolite in the uppermost mantle, with emphasis on the role of plagioclase composition in controlling melt compositions;
- to compare these melt compositions with those of mid-ocean-ridge basalts (MORBs) to test the hypothesis that MORBs are produced at shallow depth (≈15-30 km);
- to quantify liquid- and mantle residue compositional paths at decreasing T and low P to understand magma differentiation by “percolation-reaction” at shallow depth in the mantle;
- to compare experimental mantle mineral compositions to those of refertilised oceanic mantle lithosphere outcropping at the axis of oceanic spreading ridges. This would enable quantification of the pressure (i.e. depth) and temperature of the refertilisation process that leads to formation of plagioclase and indicates the minimum thickness of the lithosphere at ridge axes.
We produced a liquid in equilibrium with five mantle phases (olivine, orthopyroxene, clinopyroxene, plagioclase and spinel) to obtain high proportions and large pools of glass (>50 µm in diameter) in contact with the five mantle phases. We used a piston-cylinder apparatus, oxide mixtures representative of mantle residuum (Mg# ~ 90, enough Cr 2O 3 to get Cr-Al spinel, CaO/Na 2O for plagioclase An80/40/0) and liquids (Mg# ~ 79 - 68, Na 2O/SiO 2 similar to tholeiitic-basalt-to-phonolite). We used mostly three small capsules within one larger capsule to study the effects of composition variation at the same P-T.
Our data on liquids in equilibrium with the five phases of a plagioclase+spinel lherzolite at 0.75 and 0.5 GPa enable us to improve our understanding of natural magmatic rocks. In the normative basalt tetrahedron (Figure 1) we observe that liquids in equilibrium with the five phases plot on two parallel cotectic lines from silica-oversaturated (basaltic andesite at 0.75 GPa or andesite at 0.5 GPa) to silica-undersaturated compositions (trachyte; Figure 2) as plagioclase varies from An95 to An0. Plagioclase composition exerts major control on mantle solidus temperatures and melt compositions.
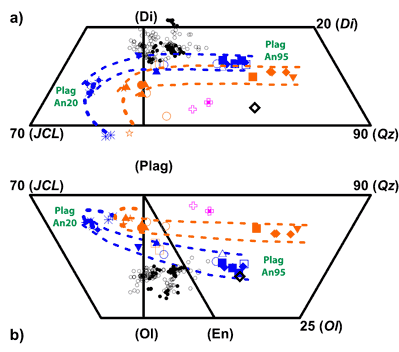
Figure 1: Glass compositions at 0.5 and 0.75 GPa projected into the normative basalt tetrahedron, illustrating the relatively narrow compositional variation of the liquids on the plagioclase+spinel lherzolite five-phase+liq cotectic and their transition from quartz-normative to nepheline-normative compositions i.e. crossing the Di-Ol-Plag plane. 0.5 and 0.75 GPa data: orange and blue fields respectively. Pink open cross and pink open cross, pink at centre: glass compositions at 0.375 GPa. The dashed lines are drawn to limit each of the five-phase+liq fields. Each of them terminates at the most silica-undersaturated glasses, and the heavy lines link to the Ca-free data points along their respective Ol+Opx+Plag+Sp+Liq cotectics with decreasing CaO in orthopyroxene and glass. Small black open circles: selected MOR glasses (61<Mg#<69) from the PETDB (www.petdb.org); small black solid circles: selected MOR glasses (61<Mg#<69) from Danyushevsky (2001). Black open diamond: mid-Atlantic Ridge glass data from Kamenetsky et al. (2011). More explanations in Chalot-Prat et al. (2010; 2013).
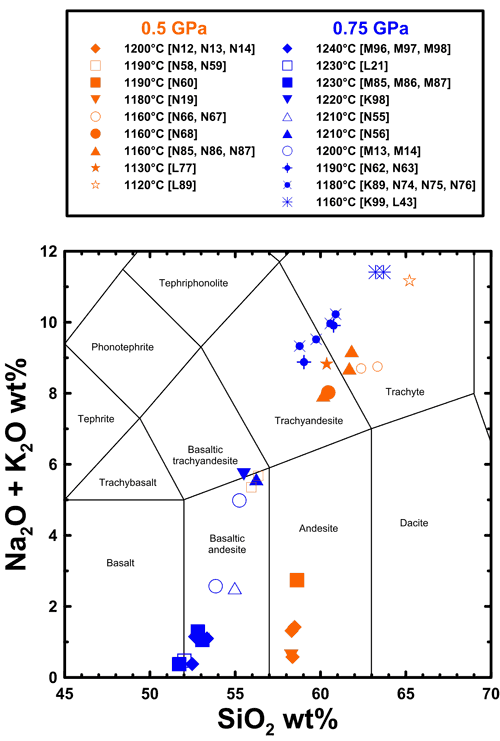
Figure 2: Glass compositions at 0.5 and 0.75 GPa in the TAS diagram, illustrating the narrow compositional variation of the liquids on the plagioclase+spinel lherzolite five-phase+liq cotectic from silica-oversaturated (basaltic andesite at 0.75 GPa or andesite at 0.5 GPa) to silica-undersaturated compositions (trachyte) as plagioclase varies from An95 to An0. 0.5 and 0.75 GPa data: orange and blue fields respectively.
The cotectic lines can be interpreted either as defining partial melting compositions or evolution trends for melts invading and metasomatizing refractory peridotite by reactive porous flow:
- In the first case, they enable mantle melting depth to be estimated for natural primitive magmatic rocks by comparison. The strong mismatch between natural glasses from MORBs (black dot and circle) and the experimental liquids at 0.75 and 0.5 GPa confirms that genesis of parental MORB occurs deeper than 30 km beneath the ridge axes. Our data do not support models of mantle upwelling at low potential temperature (1280°C) that produce low melt fractions at low pressures, leaving residual plagioclase-spinel lherzolite.
- In the second case, they provide a way to estimate metasomatic mantle melt compositions at shallow depth. Both compositional paths of experimental melts at 0.75 and 0.5 GPa are then representative of liquids interacting with mantle residues to give plagioclase + spinel lherzolites at 15 - 30 km.
The pressure-dependent IVAlCpx and IVAlOpx substitution (Figure 3f) and its correlation with Na2OCpx (Figure 3c) and AnPlag (Figure 3e) may be used to infer the pressure of crystallisation of natural mantle assemblages. Also, if the plagioclase in natural mantle rocks is saussuritised (pseudomorphed by hydrogrossular and/or epidote), the correlation of Na2OCpx content with AnPlag provides a useful way to estimate the original plagioclase composition. The pressure of crystallization of the natural mantle assemblage can then be inferred from IVAlCpx vs inferred AnPlag.
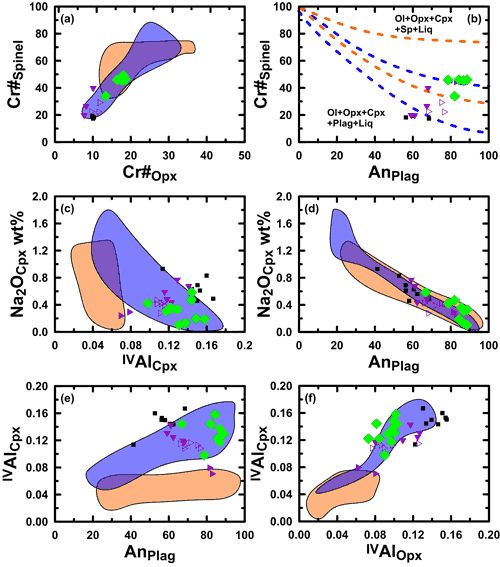
Figure 3: Mineral vs mineral correlations to compare the Lanzo plagioclase ± spinel lherzolites data from Piccardo et al. (2007), our experimental data at 0.5 and 0.75 GPa data, the plagioclase + spinel experimental data at 1 GPa from Falloon et al. (1997; 1999; 2008) and the plagioclase ± spinel lherzolite experimental data from Borghini et al. (2010; 2011). Lanzo data: green diamond; 0.5 and 0.75 GPa data: orange and blue fields respectively; 1 GPa data: black filled square; Borghini data: 0.7-0.8 GPa-mauve inverted filled triangle; 0.5-0.6 GPa-mauve right-pointing open triangle; 0.2-0.3 GPa-mauve right-pointing filled triangle.
Our experimental data on the five phases of a plagioclase+spinel lherzolite at 0.75 and 0.5 GPa are thus tools for estimating mantle refertilisation depth in natural plagioclase + spinel lherzolites. The Lanzo plagioclase ± spinel refertilised lherzolites (green diamonds, from Piccardo et al., 2007) clearly match our 0.75 GPa data, suggesting that refertilisation of the oceanic lithosphere occurs at depths of 25 - 30 km below the mid-ocean ridge axis.
Key Points
- Liquid compositions on the plagioclase + spinel lherzolite five-phase + liquid cotectic evolve from silica-oversaturated at the calcic end to silica-undersaturated at the sodic end. The lower the pressure, the greater the silica oversaturation.
- The plagioclase solid solution has a dominant role in determining the solidus temperature of plagioclase + spinel lherzolites, at a given pressure at shallow depth.
- Mantle mineral compositions are mostly pressure dependent, excluding the co-variance of Na2OCpx and AnPlag, which is pressure independent and enables estimation of AnPlag (if the plagioclase is saussuritised), knowing Na2OCpx of the natural mantle Cpx.
- The most exciting result of this high-TP experimental work, which imparts even more confidence and significance to our data, is the fit of the five phases of an experimental plagioclase + spinel lherzolite with those of natural plagioclase ± spinel lherzolites.
- The mantle refertilisation pressure of natural plagioclase ± spinel lherzolites can now be estimated between 0.75 and 1 GPa. Thus, a minimum thickness (30 km) of the oceanic mantle lithosphere can be inferred, with important consequences for plate tectonics.
References
-
Borghini, G., Fumagalli, P., Rampone E., 2010. The Stability of Plagioclase in the Upper Mantle: Subsolidus Experiments on Fertile and Depleted Lherzolite. Journal of Petrology 51, 229 – 254
-
Borghini, G., Fumagalli, P., Rampone E., 2011. The geobarometric significance of plagioclase in mantle peridotites: a link between nature and experiments. Lithos 126, 42-53.
-
-
-
Danyushevsky, L.V., 2001. The effect of small amounts of H2O on crystallization of mid-ocean ridge and backarc basin magmas. Journal of Volcanology and Geothermal Research 110, 265-280.
-
Falloon, T.J., Green, D.H., O’Neill, H.S. C., Hibberson, W.O., 1997. Experimental tests of low degree peridotite partial melt compositions, implications for the nature of anhydrous near-solidus peridotite melts at 1GPa. Earth and Planetary Science Letters 152, 149-162.
-
Falloon, T.J., Green, D.H., Danyushevsky, L.D., Faul, U.H., 1999. Peridotite melting at1.0 and 1.5 GPa: an experimental evaluation of techniques using diamond aggregates and mineral mixes for determination of near-solidus melts. Journal of Petrology 40, 1343-1375.
-
Falloon, T.J., Green, D.H., Danyushevsky, L.D., McNeill A.W., 2008. The composition of near-solidus partial melts of fertile peridotite at 1 and 1.5 GPa: implications for the petrogenesis of MORB. Journal of Petrology 49, 591-613.
-
Kamenetsky, V.S., Maas, R., Sushchevskaya, N.M., Norman, M.D., Cartwright, I., Peyve, A.A., 2011. Remnants of Gondwanan continental lithosphere in oceanic upper mantle: Evidence from the South Atlantic Ridge. Geology 29, 243-246.
-
Piccardo, G.B., Zanetti, A., Müntener, O., 2007. Melt/peridotite interaction in the Southern Lanzo peridotite: field, textural and geochemical evidence, Lithos, 94, 181–209.
last updated 12th December, 2013 |