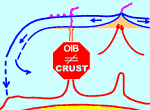 |
“Mantle
plumes” are NOT from ancient oceanic
crust |
Yaoling
Niu1 &
Michael J. O'Hara2
1Durham University,
Durham DH1 3LE, UK, yaoling.niu@durham.ac.uk
2University of Wales, Aberystwyth SY23 3DB, mio@aber.ac.uk
1. Introduction
Following the paper by Niu et
al. (2002), we point out
that there are many more difficulties than certainties in
models invoking ancient recycled oceanic crust as
the source material for ocean island basalt (OIB).
Our paper Niu & O'Hara (2004) titled “Mantle
plumes are not from ancient oceanic crust” in
the book Oceanic
Hotspots summarises our
work in progress. Our perspective contrasts
with that advanced in the classic paper, published
20 years earlier by Hofmann & White (1982),
titled “Mantle
plumes from ancient oceanic crust”. We
published a more comprehensive treatment a year later
(Niu & O'Hara,
2003) titled “Origin
of ocean island basalts: A new perspective from petrology,
geochemistry and mineral physics considerations”.
It is apparent from reading the papers by Sobolev
et al. (2005,
2007) on the same subject, that our views
have been overlooked.
In
this brief webpage, we summarise our views (Niu & O'Hara,
2003) in
an accessible form for readers. We do not ignore
the interesting issues raised by Sobolev
et al. (2005,
2007). Figures and page numbers
mentioned in the text (e.g.,
ECV 5-2) refer to those in (Niu & O'Hara,
2003).
2. Background and context
If we assume the Earth’s
primitive mantle (PM) to be compositionally uniform,
we must explain why the mantle source for mid-ocean
ridge basalts (MORB) is more depleted both isotopically
and in terms of incompatible elements than the mantle
source for OIB. By interpreting MORB mantle
depletion as resulting from continental crust extraction
in Earth’s early history (Armstrong, 1968; Gast,
1968; Hofmann, 1988), we could be satisfied with the
OIB source being less depleted than the MORB source.
However, OIB mantle source is not just less depleted,
but enriched in incompatible elements relative to the
PM. It also varies significantly in inferred abundances
and ratios of incompatible elements as well as radiogenic
isotopes from one island to another and from one group
of islands to another group. Therefore, the mantle
source for OIB is heterogeneous on all scales.
By accepting
the assumption that
the entire mantle is in the solid state and that solid-state
elemental fractionation is unlikely (Hofmann
& Hart,
1978), it is logical to suspect that processes known
to occur in the upper mantle and crust (e.g.,
partial melting and magma evolution, dehydration, alteration/metamorphism,
differential weathering, transport and sedimentation)
are the likely causes of elemental fractionation. These
shallow or near-surface fractionated materials are
then introduced into the mantle source regions of oceanic
basalts through subduction zones. Mantle compositional
heterogeneity is thus a general consequence of plate
tectonics because of crust-mantle recycling. Among
many contributions endeavoring to understand the origin
of mantle compositional heterogeneity in the context
of plate tectonics is the classic paper by Hofmann & White (1982).
They proposed that "oceanic crust
is returned to the mantle during subduction…
Eventually, it becomes unstable (at the core-mantle
boundary or CMB; see Christensen & Hofmann, 1994)
as a consequence of internal heating, and the resulting
diapirs become the source plumes of oceanic island
basalts (OIB) and hot spot volcanism." They
also stressed that this recycled ancient oceanic crust,
the plume material which is the source of OIB, is geochemically
more ‘‘enriched’’ in
K, Rb, U, Th, and light rare-earth elements relative
to the more "depleted" source
of MORB. While some details are considered conjectural,
the principal idea of the model has, since 1982, been
widely accepted by the solid Earth community as being
correct, except for those who share the view
of Niu & O'Hara (
2003).
3. Niu & O’Hara
(2003) demonstrated
that there is no obvious association between ancient
recycled oceanic crust and OIB source in terms
of petrology, geochemistry, and mineral physics
-
Melting
of oceanic crust with basaltic/picritic compositions
cannot produce high-magnesian lavas parental to
most OIB. Primitive OIB melts (> 15% MgO) are
probably more magnesian than bulk ocean crust (< 13%
MgO) (p. ECV 5-2).
-
" One may argue that
OIB are derived from melts of recycled oceanic
crusts mixed with predominantly peridotite
melts. This is possible and will apparently
relax the OIB MgO requirement, but then OIB
are no longer derived from recycled oceanic
crust alone" (p. ECV 5-2). Note
that the original theory by Hofmann & White (1982)
and Christensen & Hofmann (1994) is
incompatible with this possible scenario, but by
using it Sobolev
et al. (
2005, 2007) discovered new evidence in
support of Hofmann & White (1982)
and Christensen & Hofmann (1994) without
addressing points 3.1 and 3.2 in this list.
-
Oceanic
crust passing through subduction zone dehydration
reactions should be depleted in water-soluble
incompatible elements such as Ba, Rb, Cs, Th,
U, K, Sr, Pb relative to water-insoluble incompatible
elements such as Nb, Ta, Zr, Hf, Ti, etc. Residual
crust with such trace-element systematics is
unsuitable as a fertile source for OIB (Figures
2-3; p.
ECV 5-3,4,5). Melting or partially melting
such residual crust will never produce OIB or
any volcanic rocks ever sampled on the Earth’s
surface.
-
Ancient oceanic crust is too
depleted to produce the Sr-Nd-Hf isotopic signatures
of most OIB (Figure 1; p. ECV 5-2,3).
-
OIB Sr-Nd-Hf isotopes
preserve no signals that indicate previous subduction-zone
dehydration histories (Figures 4-5; p. ECV 5-5,7,8,9).
-
Subducted
oceanic crust at shallow lower-mantle conditions
forms mineral assemblages that are much (>2%)
denser than the ambient peridotitic mantle (Figure
6; p. ECV 5-9,10).
-
If subducted crust melts in
the deep lower mantle, this melt, depending on
its composition, may have still greater (up to
15%) density than solid peridotitic mantle. Therefore
ancient oceanic crust that has subducted into
the deep lower mantle will not return in bulk
to the upper mantle in either the solid (see
3.6 in this list) or molten state (Figure 7; p.
ECV 5-10,11,12).
-
Small fragments of subducted
oceanic crust could be returned to the upper
mantle source regions of oceanic basalts provided
they are carried along with streams of ascending
buoyant material. However, there is no convincing
evidence for the presence of bulk subducted crust
in the source regions of oceanic basalts, noting
points 3.3, 3.4 and 3.5 in this list (p. ECV 5-16).
-
It is probable
that subducting oceanic crust does not penetrate
the 660-km discontinuity, but may be stripped,
at least partly, off the slab and remain in the
transition zone. This may be a result of its lower
density compared with the peridotite mantle around
660 km (Figure 6; p. ECV 5-16). This scenario
would apparently make recycled oceanic crust a
less problematic potential source material for
OIB, but this model differs from the CMB-origin
model (Hofmann & White, 1982; Christensen & Hofmann,
1994).
Furthermore, physical difficulties remain. Subducted
crust is eclogitic in most of the upper mantle
and is probably far too dense to ascend to OIB
source regions (p. ECV 5-14).
4. Summary
Models invoking recycled
oceanic crust to explain the geochemistry of OIB must
be able to:
- demonstrate how such crust can, by melting, produce
high-magnesian lavas in many OIB suites, and
- explain the lack of subduction-zone dehydration
(metamorphic) signatures in OIB.
The latter
includes:
-
why OIB are enriched not only in water-insoluble
incompatible elements, but also water-soluble incompatible
elements,
-
why OIB are enriched in the progressively
more incompatible elements, and
-
why OIB show
significant correlated Sr-Nd-Hf isotopic variations,
all of which are magmatic (vs. metamorphic) fingerprints.
Models that require ancient subducted
crust as plume sources reactivated from the lower mantle
also require physical mechanisms to overcome the huge
negative buoyancy of subducted crust in both
the lower and the upper mantle (p. ECV 5-16)
5. Our perspective of the origin
of OIB sources (Niu & O'Hara,
2003)
The recycled deep portions of oceanic
peridotitic lithosphere are the best candidate for
the source feeding hot spot volcanism and OIB. These
deep parts of oceanic lithosphere are likely to
have been enriched in water-soluble incompatible elements
such as Ba, Rb, Cs, Th, U, K, Sr, Pb as well as all
other incompatible elements as a result of low-degree
melt metasomatism at the interface between the LVZ
and the cooling and thickening oceanic lithosphere.
These metasomatized lithospheric materials are peridotitic
in bulk composition. They can, by partial or locally
total melting, produce high-magnesian melts for primitive
OIB. Such materials (vs. crustal compositions) will
develop positive thermal buoyancy upon heating, if
subducted into the deep mantle, especially in the presence
of a peridotitic melt phase, making it possible for
the bulk material to ascend and feed OIB volcanism.
Concerning the papers by Sobolev
et al. (2005,
2007), we comment
that variations, of
the type reported, in Ni, Ca, Mn and Cr content in
olivine phenocrysts is a straightforward petrologic
consequence of peridotite melting processes and
mineral facies changes in the upper mantle. We are
investigating the extent to which these effects account
for the observations quantitatively.
4. Acknowledgements
We thank Gillian
Foulger for inviting this contribution.
References
-
Armstrong, R. L., A model for
the evolution of strontium and lead isotopes in
a dynamic earth, Rev. Geophys. Space Phys.,
6,
175-200, 1968.
-
Christensen, U. R. & A.
W. Hofmann, Segregation of subducted oceanic crust
in the convecting mantle, J.
Geophys. Res., 99, 19867-19884, 1994.
-
Gast, P. W., Trace element fractionation
and the origin of tholeiitic and alkaline magma
types, Geochim. Cosmochim. Acta, 32,
1055-1086, 1968.
-
Hofmann,
A. W. & W. M. White, Mantle plumes from ancient
oceanic crust, Earth Planet. Sci. Lett., 57,
421-436, 1982.
-
Hofmann, A. W., Chemical differentiation
of the Earth: The relationship between mantle,
continental crust, and oceanic crust, Earth
Planet. Sci. Lett., 90, 297-314,
1988.
-
Hofmann, A. W. & S. R. Hart,
An assessment of local and regional isotopic equilibrium
in the mantle, Earth Planet.
Sci. Lett., 38, 44– 62,1978.
-
-
-
-
Sobolev,
A. V., A.W. Hofmann, D. V. Kuzmin, G. M. Yaxley,
N. T. Arndt, S.-L. Chung, L. V. Danyushevsky,
T. Elliott, F. A. Frey, M. O. Garcia, A. A.Gurenko,
V. S. Kamenetsky, A. C. Kerr, N. A. Krivolutskaya,
V. V. Matvienkov, I. K. Nikogosian, A. Rocholl, I.
A. Sigurdsson, N. M. Sushchevskaya & M. Teklay,
The amount of recycled crust in sources of mantle
derived melts, Science, 20 March 2007 (10.1126/science.1138113).
-
last updated 15th
April, 2007 |