 |
|
Re-Os, Pt-Os
and Hf-W isotopes and tracing the core in mantle
melts |
Anders
Scherstén
Danmarks & Grønlands
Geologiske Undersøgelse (GEUS) , Øster
Voldgade 10 , DK-1350 København K , Denmark,anders.schersten@geol.lu.se
For a brief and
simple introduction, see "Journey
from the Centre of the Earth?" by Anders Scherstén,
Tim Elliott & Chris Hawkesworth; a NERC article.
Abstract
Positive 187Os/188Os
– 186Os/188Os correlations
in Hawaiian picrites and Gorgonan komatiites have been
interpreted as representing a small contribution from
the Earth’s core to their mantle sources [1-3].
If correct, this chemical core probe could help to constrain
core crystalisation history [2]. Furthermore, tracing
the core in oceanic lavas such as Hawaiian picrites
(“plume lavas” hereafter) may provide a
geochemical argument for the existence of mantle plumes
if they are predicted to originate at the core-mantle
boundary. Hf-W isotope systematics provide a test of
the core contribution model and core-mantle admixtures
are predicted to show weak but resolvable W-isotope
anomalies. Data from Hawaii and South African kimberlites
yield no anomalies and do not support the core contrbution
model put forward on the basis of Os-isotope systematics.
Pt-Re-Os
Planetary core formation
fractionates iron from the silicate mantle, and siderophile
(iron loving) elements are strongly enriched in the
core while lithophile elements remain in the primitive
silicate mantle. Core formation thus causes extreme
concentration contrasts between the mantle and the core
and even a very small contribution of core material
into the mantle will control the siderophile element
budget of the admixture.
If it is assumed that
the bulk Earth is chondritic, then the bulk core should
have near chondritic relative abundances of the highly
siderophile elements. The reason is simply that nearly
100% of the highly siderophile elements partitioned
into the core. For example, Pt/OsCore ~ Pt/OsChondrite.
The isotopic composition of osmium (Os; non-radiogenic
isotopes are, e.g., 188Os and 189Os;
click here for further information),
which changes due to the decay of rhenium (Re; 187Re
→ 187Os; half-life 41.6 Ga) and platinum
(Pt; 190Pt → 186Os;
half-life ~490 Ga), will thus be indistinguishable from
a chondritic reference or the Primitive Mantle (PM)
e.g., [4], which appears to be near-chondritic
in its platinum group element (PGE) and Re budget. Discussion
of the cause of the PM PGE composition is beyond the
scope of this webpage, but one hypothesis stipulates
a late veneer of chondritic material.
Crystalisation of the
inner core might, by analogy with siderophile element
fractionation in iron meteorites, fractionate the siderophile
elements [5]. Although this may be a matter of debate,
it is assumed here that Os is more compatible in the
inner core than Re, which is more compatible than Pt.
Inner core crystalisation would thus lead to an outer
core that has an excess of Pt and Re relative to Os,
i.e., suprachondritic Re/Os and Pt/Os ratios. Because
187Re and 190Pt decay to form
187Os and 186Os, depending on
the timing and extent of inner core fractionation, the
187Os/188Os and 186Os/188Os
isotope ratios in the outer core may have become more
radiogenic than the chondritic reference or the mantle.
It thus follows that if the interpretation of Brandon
et al. [1;2;6] is correct, that the outer core
has suprachondritic Os isotope ratios, then inferences
about the crystalisation history and composition of
the Earth’s core can possibly be made. One question
is; when might the inner core have started to crystalise?
Given the very long half-life of 190Pt (~490
Gyr) and the very low abundance of all isotopes of Pt
(~0.013%), the inner core must have begun fractionating
early in the Earth's history in order to produce more
radiogenic 186Os/188Os in the
outer core, and in "plume lavas" containing
outer core material.
An underlying assumption
is, of course, that Pt/Os and Re/Os were indeed fractionated
by inner core crystallisation such that the outer core
has suprachondritic ratios. This assumption may be at
odds with some recent experimental data [7] but supported
by others [8].
Brandon et al.
[2] infer an outer core 186Os/188Os
ratio of 0.119870. This value for the outer core can
be achieved for many different Pt/Os ratios and inner
core crystalisation timescales. Simply put, the earlier
and faster the inner core crystalises, the lower the
Pt/Os ratio must have been for the inferred present
outer-core ratio of 0.119870 to have been achieved:
- early, fast inner core crystalisation
– low outer core Pt/Os with slow 186Os/188Os
and 187Os/188Os increase (for
a present-day outer core 186Os/188Os
ratio of 0.119870), or
- late, slow inner core crystalisation
– high outer core Pt/Os with fast 186Os/188Os
and 187Os/188Os increase.
For a fixed Pt partition
coefficient, a lower Pt/Os ratio naturally means a higher
Os concentration in the outer core. A higher Os concentration
in the outer core translates to higher Os in a fixed
core-mantle admixture with a given percentage of core
material. Alternatively, from the point of view of surface
observations, for any fixed 186Os/188Os
ratio that is interpreted as a core-mantle admixture,
the core contribution will be smaller if the outer core
Os-concentration is higher. It should be noted, however,
that the higher the Os concentration postulated for
the outer core, the earlier inner core crystalisation
is required to begin as the Pt/Os ratio of the outer
core decreases.
If mantle plumes start
at the base of the mantle and the core began crystalising
early enough to develop suprachondritic 186Os/188Os
and 187Os/188Os ratios in the
outer core, then a small core-mantle admix erupted at
the surface would manifest itself in a suprachondritic
186Os/188Os ratio. It would be
expected that other siderophile elements would also
provide evidence for such an admixture.
Hf-W
Hafnium (Hf) and tungsten
(W) are refractory elements with a limited range in
chondritic Hf/W ratios [9;10]. It therefore seems justified
to assume that the bulk Earth Hf/W ratio is also chondritic.
W is siderophile and most of the Earth’s W is
in the core [11]. Hf, on the other hand, is lithophile
and absent in the Earth’s core. Planetary core
formation thus strongly fractionates Hf/W ratios such
that they are suprachondritic in silicate mantles and
zero in metallic cores. Whilst core formation depletes
the mantle of W, partial mantle melting will cause further
depletion as W behaves as an incompatible element during
mantle partial melting, much like thorium (Th) and lanthanum
(La). Like the core, the Earth's crust is therefore
also strongly enriched in W relative to the PM (Figure
1).
|
Figure
1: A cartoon of the Earth and its Hf-W budget.
The primitive mantle (PM) W/Th ratio of 0.19 +7/-5
[11] forms the basis for the concentration calculations,
which translates into 15 +5/-4 and ~500 ppb W
in PM and the bulk core (BC) respectively. Continental
crust (CC) generation depletes the mantle further.
Averaging this amount over the entire mantle volume
produces an 8 ppb W concentration (right half
of figure), while a layered mantle scenario produces
a strongly depleted upper mantle (DUM) and a virtually
undepleted lower mantle (LM) for WPM = 20 ppb
(left half of figure). |
182Hf decays
to 182W with a half-life of ~9 Myr [12],
which means that only Hf/W fractionation processes that
occurred within the first ~60 Myr of solar system evolution
can produce non-chondritic W isotope compositions through
182Hf decay. The W isotope composition is
expressed as ε182W, where silicate Earth
ε182W = 0 (Figure 2). The current best
estimate for the solar system initial value is ε182W
= -3.5 [10;13]. The present silicate Earth 182W/184W
ratio is 3.5 parts in ten thousand higher than this.
Chondritic meteorites have a relatively uniform ε182W
of -1.9. The evolution curve of W is shown in Figure
2. If it is assumed that the bulk Earth composition
is chondritic, core ε182W is calculated
to be about -2 by mass balance. Importantly, the ~2
ε182W difference between the core and
the silicate Earth (or PM) will remain constant throughout
Earth's history as 182Hf had essentially
all decayed away by ~60 Myr after solar system formation.
Later mantle fractionation will have had no effect,
and core contribution to the mantle is probably the
only process that could significantly alter the ε182W
composition of the PM later, towards lower ε182W.
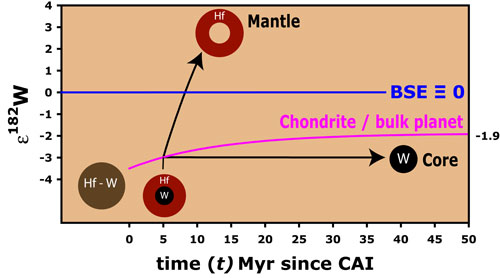
Figure 2: Tungsten
evolution diagram where t is time in Myr after the condensation
of calcium-aluminium rich inclusions and ε182W
is the deviation from silicate Earth (or PM) in parts
per ten thousand (ε182W = {[182W/184W]spl/[182W/184W]PM–1}
x 10,000). Thus, compositions with high 182W/184W
plot above the blue line and compositions with low 182W/184W
plot below. A chondritic unfractionated planet (brown)
would evolve along the chondritic evolution line (purple).
Core formation fractionates Hf and W such that the Hf/W
ratio of the core is zero (because it contains essentially
no Hf) and therefore remains unchanged with time (black
horizontal line). The silicate mantle will have a suprachondritic
Hf/W ratio and grow increasingly more radiogenic ε182W
depending on the timing and extent of Hf/W fractionation.
The bulk silicate Earth (BSE; or primitive mantle; blue)
has an ε182W that is zero by definition.
The
debate regarding a possible Hf-W contribution from the
core
Lavas from Hawaii and
South African kimberlites do not display resolvable
negative anomalies as would be predicted from a core
contribution (Figure 3). The immediate question is whether
this is due to insufficient sensitivity.
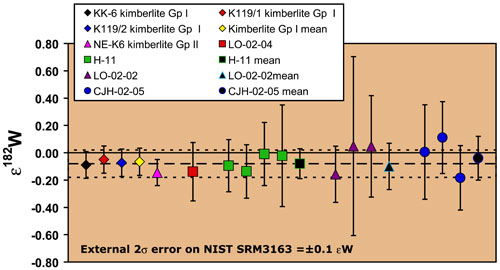
Figure 3: ε182W
values for Group I and Group II kimberlites and Hawaiian
picrites. For no sample is ε182W resolved
from the silicate Earth standard NIST SRM3163. A more
detailed discussion of this figure is available in [14].
Mixing
models
The sensitivity of Hf-W
for detecting a core contribution to proposed plume
lavas depends on the isotope composition and concentration
contrasts of the mixing reservoirs. The main variables
that affect the core-mantle contrast are the amount
of W mantle depletion due to core formation, whether
mantle depletion due to the formation of the continental
crust should be averaged over the entire mantle or only
the upper mantle, and whether there are significant
amounts of W-rich recycled crust in the source. Recycled
crust would be expected to have the same ε182W
as the mantle with which it mixes, but a larger absolute
concentration. A contribution from the crust would thus
act to decrease the concentration contrast between the
core and the mantle, thus decreasing the sensitivity
of the Hf-W system to a core contribution (c.f.
[15]).
Scherstén
et al.
[14] tested two end-member models for core-mantle
mixing (Figure 4). In Model 1, the average W depletion
due to core formation (PM = 15 ppb) was combined with
average depletion due to continental crust formation
over the entire mantle volume. They adopted an outer
core Os concentration of 300 ppb, which is the average
of inner core crystalisation Models 3 and 4, as described
in [2]. In Model 2, a minimum value for depletion due
to core formation was used (PM = 20 ppb) and it was
assumed that the continental crust was almost entirely
drawn from the upper mantle, while the lower mantle
that mixed with the core was virtually undepleted. The
inner-core-crystalisation Model 1 of Brandon et
al. [2] was used to maximise the outer core concentration
at 660 ppb. Thus, Model 1 suggests a large contrast
in W concentration between the outer core and the mantle,
and a high ε182W whereas Model 2 suggests
the reverse.
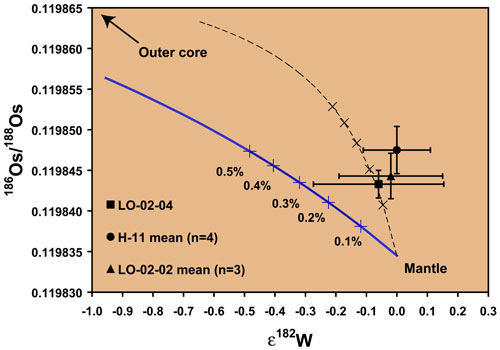
Figure 4: ε182W
– 186Os/188Os mixing models
for two core-contribution scenarios. The blue line represents
Model 1 while the dashed black line represents Model
2 (see text for explanation). Hawaiian picrites are
plotted with 2σ error bars.
Scherstén
et al.
[14] preferred Model 1 and argued that the more
extreme Model 2, which was developed to force a fit
with their Hawaiian data, is implausible given the large
number of end-member assumptions that have to be made.
As a result, they concluded that there is no detectable
contribution from the Earth’s core in hotspot
lavas. However, a recycled crustal component enriched
in W may increase the W content of the source such that
the sensitivity of W isotopes to a core component is
lost [14].
This possibility can be
assessed using concentration data, and using Th as a
proxy for W. Tungsten behaves as an incompatible element,
like Th, in the mantle silicate system. Melting thus
depletes the mantle of W. W/Th ratios in rocks from
all settings and age have very similar values, with
an average of 0.19 ± 0.6 [11]. This shows that
W/Th is not significantly fractionated by most geological
processes and that the observed ratio of 0.19 is likely
to be representative of the primitive mantle. A core
contribution would increase the observed W/Th ratio
in a melt by a small but insignificant amount (a 0.5%
admix would increase the W/Th ratio from 0.19 to 0.25),
but the measured Th-concentration of the melts can nevertheless
be used to estimate the W concentration of the source.
Using Th as a proxy for
W (DTh ~ DW), and assuming a source
W/Th ratio of 0.25 for the data from Norman &
Garcia [16] (a generous estimate that maximises
the estimated W in the source and minimises the effect
on the W isotopes of a core contribution), the Hawaiian
source is predicted to be moderately depleted with ~10
ppb W, and W isotopes should detect a core contribution
if it is present, according to the preferred mixing
curve shown in Figure 4 (Model 1).
If the conclusion of Scherstén
et al. [14]
is correct, that there is no contribution from the Earth’s
core to the Hawaiian source, then Os-isotope correlations
cannot be used to argue for the existence of mantle
plumes from the core-mantle boundary. On the other hand,
W isotope systematics cannot disprove their existence.
|
References
-
A.D. Brandon,
R.J. Walker, J.W. Morgan, M.D. Norman, and H.M.
Prichard, Coupled Os-186 and Os-187 evidence for
core-mantle interaction, Science 280,
1570-1573, 1998.
-
A.D. Brandon,
R.J. Walker, I.S. Puchtel, H. Becker, M. Humayun,
and S. Revillon, Os-186-Os-187 systematics of Gorgona
Island komatiites: implications for early growth
of the inner core, Earth and Planetary Science
Letters 206, 411-426, 2003.
-
A.D. Brandon,
M.D. Norman, R.J. Walker, and J.W. Morgan, Os-186-Os-187
systematics of Hawaiian picrites, Earth and
Planetary Science Letters 174,
25-42, 1999.
-
T. Meisel,
R.J. Walker, and J.W. Morgan, The osmium isotopic
composition of the Earth's primitive upper mantle,
Nature 383, 517-520, 1996.
-
R.J. Walker,
J.W. Morgan, and M.F. Horan, Os-187 Enrichment in
some plumes - Evidence for core-mantle interaction,
Science 269, 819-822,
1995.
-
A.D. Brandon,
Os-186-Os-187 systematics of Gorgona komatiites
and Iceland picrites, Geochimica et Cosmochimica
Acta 66, A100-A100, 2002.
- S. Keshav and J.A. Van Orman. Re-Os-Pt
Partitioning in sulfur-bearing solid/molten iron metal
at 3-22 GPa and 1300-1775 C: Is the Earth's outer
core so giving? AGU Chapman Conference " The
Great Plume Debate: The Origin and Impact of LIPS
and Hot Spots", abstract, p 83-84, 2005.
- D. Walker, Core participation in mantle
geochemistry: Geochemical Society Ingerson Lecture,
GSA Denver, October 1999, Geochimica et Cosmochimica
Acta, 64, 2897-2911, 2000.
-
T. Kleine,
K. Mezger, C. Munker, H. Palme, and A. Bischoff,
Hf-182-W-182 isotope systematics of chondrites,
eucrites, and martian meteorites: Chronology of
core formation and early mantle differentiation
in Vesta and Mars, Geochimica et Cosmochimica
Acta 68, 2935-2946, 2004.
-
Q.Z. Yin,
S.B. Jacobsen, K. Yamashita, J. Blichert-Toft, P.
Telouk, and F. Albarede, A short timescale for terrestrial
planet formation from Hf-W chronometry of meteorites,
Nature 418, 949-952, 2002.
-
H.E. Newsom,
K.W.W. Sims, P.D. Noll, W.L. Jaeger, S.A. Maehr,
and T.B. Beserra, The depletion of tungsten in the
bulk silicate Earth: Constraints on core formation,
Geochimica et Cosmochimica Acta 60,
1155-1169, 1996.
-
C. Vockenhuber,
F. Oberli, M. Bichler, I. Ahmad, G. Quitte, M. Meier,
A.N. Halliday, D.C. Lee, W. Kutschera, P. Steier,
R.J. Gehrke, and R.G. Helmer, New half-life measurement
of Hf-182: Improved chronometer for the early solar
system, Physical Review Letters 93,
2004.
-
T. Kleine,
C. Munker, K. Mezger, and H. Palme, Rapid accretion
and early core formation on asteroids and the terrestrial
planets from Hf-W chronometry, Nature 418,
952-955, 2002.
-
-
A.D. Brandon
and R.J. Walker, The debate over core-mantle interaction,
Earth and Planetary Science Letters 232,
211-225, 2005.
-
M.D. Norman
and M.O. Garcia, Primitive magmas and source characteristics
of the Hawaiian plume: petrology and geochemistry
of shield picrites, Earth and Planetary Science
Letters 168, 27-44, 1999.
|
last updated 22nd
December, 2005 |
|
|