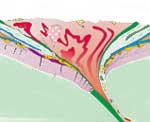 |
Accretionary-Style Plate Tectonics Explains the Preserved Geological Record Through Time, Including the Eoarchean |
Tim Kusky1,2 Brian Windley2,1 & Ali Polat3,1
1State Key Laboratory of Geological Processes and Mineral Resources, Center for Global Tectonics, Three Gorges Center for Geohazards, China University of Geosciences, Wuhan 430074, China; tkusky@gmail.com
2Department of Geology, University of Leicester, Leicester LE1 7RH, UK; brian.windley@btinternet.com
3School of the Environment, University of Windsor, Windsor, ON, Canada N9B; polat@uwindsor.ca
This web page is a summary of two recent papers, and the philosophy therein:
Windley, B.F., Kusky, T.M., and Polat, A., 2020. Onset of plate tectonics by the early Archean. Precambrian Research. Article Number: 105980. published Oct. 29, 2020. https://doi.org/10.1016/j.precamres.2020.105980
Kusky, T.M., Windley, B.F., and Polat, A., 2018. Geological evidence for the operation of plate tectonics throughout the Archean: Records from Archean paleo-plate boundaries, Journal of Earth Science, 29, 6, 1291-1303, https://doi.org/10.1007/s12583-018-0999-6
Introduction
There is a growing trend in the geosciences to interpret the planet's oldest rocks and possible tectonic modes by forward modeling from estimated but unknown starting conditions, to end up with a mobile lid planet where plate tectonics, with which we are familiar, operates today. Many of these models use different data sets, such as numerical modeling of presumed thermal and mechanical states, geochemical proxies, or just imagination, to suggest a wide variety of alternative Earth models for the early Earth, including plume-dominated heat loss through stagnant lids, heat pipes, squishy hot lids, catalytically-driven mantle overturn models, and the like. These models are useful constructs and many are scientifically valid possibilities for the behavior of some hypothetical planets, but few are testable from Earth's preserved rock record (see discussion in the papers above, and by Harrison (2020) and Burke (2016). Few are consistent with a holistic view of the complexities of intersystem relationships preserved in the rock record, but instead only explain a very limited, specifically-chosen internally-consistent data set that can be explained by the chosen model.
In our two papers (Kusky et al., 2018; Windley et al., 2020) we take a different approach, and test the preserved rock record to see if it is consistent with formation by processes known to be products of plate tectonics in the extant plate regime. This is a direct application of the scientific method where the null hypothesis (plate tectonics) is tested against the rock record, and if it passes the null hypothesis test by having a high degree of similarity between the geological features produced by plate tectonics in the modern and ancient records, then we conclude that plate tectonics is the most likely mechanism to have produced those features. An analogy can be made with a legal case, where the defendant (plate tectonics) is presumed innocent, until the data (the geological rock record) can prove it guilty i.e. not able to explain the features of the rock record using the well-established principles of tectonic analysis. In the cases where plate tectonics passes the null hypothesis test, there is no justifiable reason, using the scientific method, to call on other hypothetical models, many with no current way of being tested, to explain small parts of that record. We find overwhelmingly that nearly every feature in the geological record has a modern analog, and most importantly, that the well-established relationships between orogenic zones in modern and ancient tectonic settings are strikingly similar, and thus most likely to have been produced by plate tectonics.
Structure and Components of Orogenic Systems Through Earth History
In our analysis of the structure and components of orogenic systems through Earth history (Kusky et al., 2018; Windley et al., 2020), we ask not "when did plate tectonics begin", but rather, "when is the youngest time in the geologic record for which plate tectonics can be proven to not have been in operation." This method thus contrasts the forward modeling methods where different researchers propose a wide variety of models for Earth evolution, based on presumed starting conditions, and see when the conditions are suddenly permissive, or gradually merge into a plate regime. Instead, we look at the geological record, going back in time, to see how similar or different that record is in the past, from the present, to test how it changed, and if plate tectonics can still explain these features.
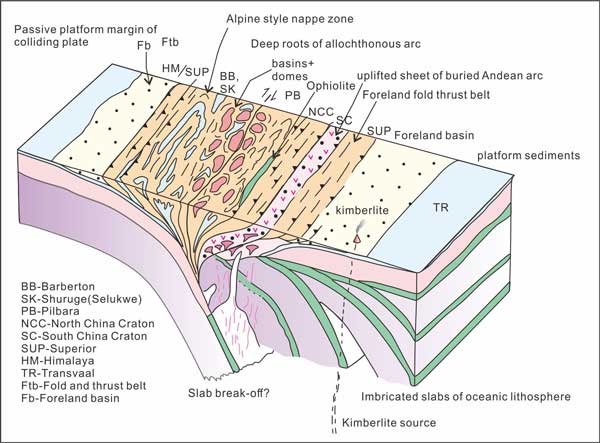
Figure 1: A composite model of an ideal orogen (from Kusky, 2020, modified from Kusky et al., 2018), incorporating elements of maps and cross-sections of orogens of all ages. Note the classical tectonic zonation from passive margin sequences, to foreland basins, fold-thrust belts, allochthonous ophiolites and arc terranes, into the high-grade metamorphic core of the orogen, typically containing gneiss domes and intervening synforms (such as Pilbara, Canadian Cordillera, etc.). This model can be applied to understand orogens of any age, and most structures found in mountain belts are shown on the figure (metamorphic core complexes not shown at this stage). Note that the different locations on the map and section correspond to real places in convergent and collision belts of all ages on Earth, including Barberton (BB), Shurugwe (SK), Pilbara (PB), North China Craton (NCC), South China (Yangtze) Craton, Superior Craton (SUP), Himalaya (HM), Transvaal (TR). Imbricated slabs of subducted ocean lithosphere form the root of the craton, and kimberlites that pass through these slabs can incorporate xenoliths of Archean eclogite from the subducted slabs, or fertile mantle peridotite.
In this type of analysis, we do not look at only one feature, such as the chemistry of a rock that may or may not be produced in an arc, we test entire orogenic systems such as the ideal orogen shown in Figure 1, and in more detail in Figure 2, and compare their features, finding that rifts look like rifts back to more than 3.0 Ga, subduction zones, with their paired belts of accreted oceanic material in fold/thrust/mélange systems, and arc-type magmatic belts, some with contemporaneous spatially and temporally linked paired metamorphic belts, are found up to about 4 Ga, the age of the oldest preserved rocks (Kusky et al., 2020; Huang et al., 2020). Large horizontal motions of adjacent plates can be demonstrated by paleomagnetic means, and sub-horizontal motion vectors on continental-scale strike-slip fault systems back to at least the Mesoarchean (Kusky et al., 2018; Brenner et al., 2020). The list of comparative tectonic settings goes on to include most that are known on the present Earth (Windley et al., 2020), leading us to the robust conclusion that plate tectonics passes the null hypothesis test, and therefore, it is incumbent upon those who wish to propose alternative non-plate models to show why they should be accepted, in lieu of plate tectonics, instead of those using a plate model to interpret the record. Some other groups have suggested that some provinces in the Archean have unique map patterns unlike those on the present Earth (such as dome and basin structures) but in Kusky et al. (2018), and Windley et al. (2020) we show this to be false, and they simply represent preservation of specific tectonic environments, such as roots of arcs.
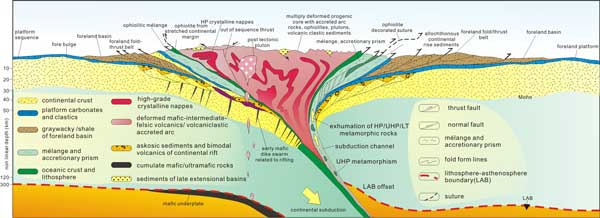
Figure 2: Cross section through an ideal collisional orogen (from Kusky et al., 2016), incorporating many of the structural, sedimentological, petrological, and metamorphic relationships in orogens of all ages. Archean accretionary orogenic remnants have since been involved in collisional orogenesis, and are thus found in younger collisional orogens. Click here or on Figure for enlargement.
Secular Changes in the Dominant Plate Tectonic Style
The planet has been cooling for billions of years (Arevalo et al., 2009), and has gone though many secular changes in mantle potential temperature, atmospheric and ocean chemistry, the amount of heat lost from the surface at any given time, and changes in the biology, surface weathering, and distributions and volume of continental vs. oceanic crustal material (Korenaga et al., 2018; Wang et al., 2020). Other changes may relate to magmas produced under slightly warmer or other mantle conditions, differences in the dips and thicknesses and temperatures of subducting slabs, and hence the types of arc magmas or metamorphic facies produced during orogenesis (Brown et al., 2020). However, many of these changes can be found within the variation on the modern Earth, or be within the limits where plate tectonics can still operate as the main planetary heat loss mechanism.
Earth presently, and in the recent past, has many examples of shallow hot subduction as well as steep cold subduction, subduction of thick, as well as thin oceanic plates, subduction erosion, subduction accretion, and the list goes on (Windley et al., 2020). Despite this huge variation in the ranges of conditions in the present plate regime, the relative balance of different types of subduction, and other features, may have changed with time. One example of a well-documented change in the geologic response to changing thermal conditions on Earth is the development of a dichotomy in potential subduction-related low dT/dP with high dT/dP metamorphic assemblages sometime in the Archean (Brown & Johnson, 2018, 2019). There is well-documented spatially and temporally linked paired metamorphism in plate-scale accretionary wedge-type fold thrust belts in the Neoarchean (Huang et al., 2020) and relicts of such relationships proposed for the Eoarchean (Nutman et al., 2020). Other changes may include the decrease in komatiite abundance with time, reflecting decreasing mantle temperatures, and changes in rock types induced by differences in the biology, atmospheric chemistry, weathering, and distribution of landmasses in early times (Harrison, 2020; Wang et al., 2020). These topics are discussed, with examples from the geological record, in terms of plate tectonics, in Windley et al. (2020).
Accrectionary Cycle and Wilson Cycle Plate Tectonics Through the Ages
Keeping the secular changes discussed above in mind, Windley et al. (2020) propose an early Earth dominated by accretionary orogen style tectonics. Intra-oceanic arcs grew in the early oceans, forming chains of arcs in archipelagoes that gradually collided and grew into thicker and wider continental-style landmasses. These developed more continental margin-style arcs by around 3.2-3.0 Ga, with the first supercontinent size landmasses emerging around 2.7-2.5 Ga, recorded in the first widespread preservation of continental rift sequences and intracontinental dike swarms.
Thus, Windley et al. (2020) conclude that plate tectonics has been operating on Earth since the beginning, albeit in earlier forms dominated by accretionary orogen cycle tectonics, with the gradual growth of Wilson cycle tectonics around the Archean-Proterozoic boundary. From that time onward Earth has had both accretionary and Wilson cycle plate tectonics. This is recognized in the accretionary orogens of the circum-Pacific and older examples in the Arabian Nubian Shield. It is also seen in the Altaids and younger Wilson Cycle orogens such as in the Alpine-Himalayan systems and Appalachian-Caledonian orogens, from which they were originally defined.
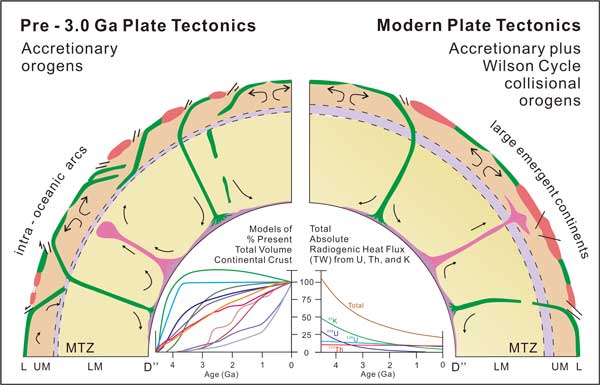
Figure 3: Schematic diagram illustrating two types of plate tectonics (from Windley et al., 2020): pre-3.0 Ga, dominated by intra-oceanic arcs and accretionary orogens during Accretionary Orogen Style Plate Tectonics, and post-2.7-2.5 Ga modern plate tectonics, which includes both Accretionary Orogen Style and Wilson Cycle Style Tectonics. On the early Earth plate tectonics was dominated by the formation of juvenile crust at oceanic spreading centers and juvenile oceanic arcs. Accretionary orogens formed at sites of subduction, with accretion of oceanic sediments and upper layers of oceanic lithosphere, as well as subduction erosion, slab and sediment melting, and arc magmatism from hydrous melting of the mantle wedge. Arcs gradually grew, amalgamated, and formed archipelagos large enough to host continental margin arcs. These contained contributions from slab dehydration and melting of the mantle wedge plus older crustal materials, with a gradual rise of continental magmatism around 3.2-3.0 Ga in the geological record, as well-shown by the archives of the Kaapvaal craton. Large continental land-masses emerged around 2.7-2.5 Ga, with the first widespread appearance of rift-passive margin sequences, break-up of large continents (supercontinents?) and collision of these dispersed fragments in Alpine-style collisional orogens marking the start of the Wilson Tectonic Cycle. In the modern tectonic regime, Earth has two types of plate tectonics, including Accretionary Cycle Plate Tectonics, and Wilson Cycle Plate Tectonics. Crustal growth curves in inset are from various authors, summarized by Hawkesworth et al. (2020). Total heat flux curve in inset from Arevalo et al. (2009). L-lithosphere, UM-upper mantle, MTZ-mantle transition zone (drawn at 410-660 km modern, 430-640 km at 3.0 Ga), LM-lower mantle, D'', the core-mantle boundary zone. Note that we draw these boundaries in the pre-3.0 Ga Earth model similar to those at present (with a slightly thinner mantle transition zone), since at present there is no well-constrained model for placing these boundaries elsewhere.
In summary, we urge geologists to remember their roots. Geology is a field-based science and any model or hypothesis must stand the test of the preserved geological record. Of course we should not be limited by the macroscopically observable rock record, and must incorporate all relevant observations, testable data, and possible scenarios raised by numerical modeling or thought experiments. At the same time, hypotheses must be consistent with observations of the preserved rock record and used to guide further thought and models. However, models should not limit the observations to a specialists "knowledge of the particulars" without considering the entire system (Sengör, 2019). The older rock record is preserved in complexly deformed and metamorphosed orogenic remnants (as in Figures 1 and 2), which must be well-understood before isolated geochemical or geochronological samples, or numerical models of complex systems can be interpreted. The complex system recorded in the history in the rock record has been firmly established by centuries of studies. Thus, while hypotheses and theories must always be tested, there is no validity to methods whereby models are haphazardly constructed to explain isolated data points. Similarly there is no validity in proposing multiple alternative reality models that can potentially explain isolated pieces of data, without considering the entire system.
References
-
Arevalo, R., McDonough, W. F., Luong, M., 2009. The K/U ratio of the silicate Earth: Insights into mantle composition, structure and thermal evolution. Earth and Planetary Science Letters, 278, 361–369. https://doi.org/10.1016/j.epsl.2008.12.023.
-
Brenner, A.R., Fu., R.R., Evans, D.A.D., Smirnov, A.V., Trubko, R., and Rose, I.R., 2020, Paleomagnetic evidence for modern-like plate motion velocities at 3.2 Ga. Science Advances 6, eaaz8670. https://doi.org/10.1126/sciadv.aaz8670.
-
-
-
-
Burke, K.C., 2016. Theorems in pure mathematics can be proved right but the models used in applied mathematics, natural and social science, as well as in engineering, can at most be “not yet proved wrong”. Canadian J. Earth Sci. 53, 1440–1442. https://doi.org/10.1139/cjes-2016-0129.
-
Harrison, T.M., 2020, Hadean Earth, Springer, ebook. https://doi.org/10.1007/978-3-030-46687-9, 291 pp.
-
-
Huang, B., Kusky, T., Johnson, T., Wilde, S., Wang, L., Polat, A., Fu, D., 2020. Paired metamorphism in the Neoarchean: a record of accretionary-to-collisional orogenesis in the North China Craton, Earth and Planetary Science Letters 543, 116355. https://doi.org/10.1016/j.epsl.2020.116355.
-
-
-
Kusky, T.M., Polat, A., Windley, B.F., Burke, K.C., Dewey, J.F., Kidd, W.S.F., Maruyama, S., Wang, J.P., Deng, H., Wang, Z.S. Wang, C., Fu, D., Li, X.W., and Peng, H.T., 2016, Insights into the tectonic evolution of the North China Craton through comparative tectonic analysis: A record of outward growth of Precambrian continents, Earth-Science Reviews, 162, 387-432, http://dx.doi.org/10.1016/j.earscirev.2016.09.002.
-
Kusky, T.M., Windley, B.F., and Polat, A., 2018, Geological evidence for the operation of plate tectonics throughout the Archean: Records from Archean paleo-plate boundaries, Journal of Earth Science, 29, 1291-1303, https://doi.org/10.1007/s12583-018-0999-6.
-
Kusky, T.M., Wang, J.P., Wang, L., Huang, B., Ning, W.B., Dong, F., Peng, H.T., Deng, H., Polat, A., Zhong, Y.T., Shi, G.Z., 2020. Mélanges through time: Life cycle of the world’s largest Archean mélange compared with Mesozoic and Paleozoic subduction-accretion-collision mélanges. Earth-Sci. Rev. 209, 103303. https://doi.org/10.1016/j.earscirev.2020.103303.
-
Nutman, A.P., Bennett, V.C., Friend, C.R.L., Yi, K., 2020. Eoarchean contrasting ultra-high-pressure to low-pressure metamorphisms (<250 to >1000°C/GPa) explained by tectonic plate convergence in deep time. Precambrian Research 344, 105770. https://doi.org/10.1016/j.precamres.2020.105770
-
-
Wang, Z.S., Liu, Y.S., Zong, K., Lin, J., and Kusky, T.M., 2020. Mantle degassing related to changing redox and thermal conditions during the Precambrian supercontinent cycle, Precambrian Research 350, 105895. https://doi.org/10.1016/j.precamres.2020.105895
-
last updated 6th December, 2020 |