 |
Non-Radial
Dikes of the Central Atlantic Magmatic Province
Reveal Tectonic Source and Evolution
of the Break-Up of Pangaea |
Erin K. Beutel
Dept. Geology & Environmental
Geosciences, College of Charleston, beutele@cofc.edu
This webpage is a synopsis of the paper: Beutel,
E.K., Magmatic rifting of Pangaea linked to onset
of South American plate motion, Tectonophysics, 468,
149-157, 2009.
Background
In 1971, P.R. May published a paper
suggesting that the diabase dike swarm along the south
and central eastern margin of North America was radial.
This was based on aeromagnetic data showing NW-SE,
N-S, and NE-SW trending dikes geographically separated
along the coast. These dikes are associated with the
break-up of Pangaea and the emplacement of the Central
Atlantic Magmatic Province (CAMP) and have been
proposed to be the result of a plume (e.g., Ernst & Buchanan,
1997; Wilson, 1997; Marzoli et al., 1999; Dalziel
et al., 2000; Storey et al., 2001; Janey & Castillo,
2001) despite numerous papers to the contrary
(e.g., King & Anderson,
1998; McHone, 2000; van Wijk et al.,
2001; Beutel et al., 2005; Nomade et al.,
2007).
However, despite much reiteration
that the dike swarm is radial, field observations and
State Survey maps from the Carolinas suggest that the
swarm is not radial, and in fact overlaps to a considerable
degree in South and North Carolina along the SE
margin of North America (Figure 1). Furthermore, these
dikes have been shown to have been emplaced in a regular
order, not simultaneously, within a 2-Ma time window
around 200 Ma (Hames et al., 2000; Salters
et al.,
2003; Beutel et al., 2005; Nomade et al.,
2007). Cross-cutting relationships consistently show
that the NW-trending dikes were emplaced first over
230-Ma NE-trending rifts, followed by the N-trending
and finally by the NE-trending dikes (Ragland,
1983; McHone et al., 1987; McHone et al.,
1988; Schlishe 2002; Schlishe et al.,
2003). There appears to be a geographic and probably
orientation (and therefore timing) relationship between
the dikes (Ragland, 1983;
de Boer & Snider, 1997; Salters
et al.,
2003; Beutel et al., 2005; Nomade et al.,
2007). New, more detailed studies are currently
underway (A. Marzoli, personal communication).
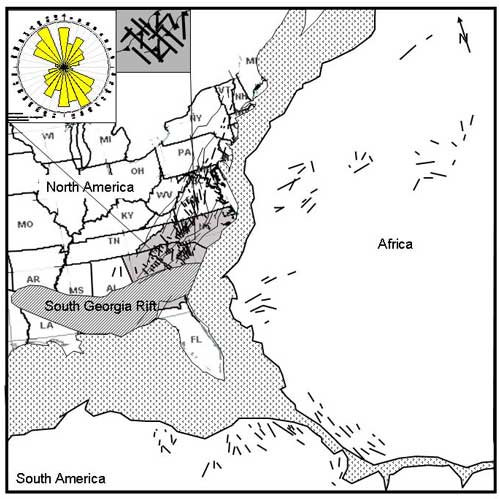
Figure 1: Dikes of
eastern North America modified from Beutel
(2009).
This map shows more complicated dike patterns than
previously depicted. Rose diagram shows dike directions
from State Survey Geologic Maps and represents confirmed
true dike orientations.
Given the non-radial nature of the
dikes, the rapidly changing stress field necessary
to create three distinct dike-swarm orientations within
2 Ma became the focus of my research. The rift- and
dike-orientations suggest that at ~ 230 Ma the stress
field was predominantly NW-SE extensional, but that
at ~ 200 Ma it rotated by 90° to become
NE-SW extensional (Figure 2). Then within 2 Ma it changed
again and became E-W extensional, and finally NW-SE
extensional again (Figure 2). While local and even
regional stress fields may vary widely due to faulting
and strain partitioning, the continental scale of the
emplaced dikes and required stress field suggests that
major tectonic forces must have controlled the stress
field changes within the continent.
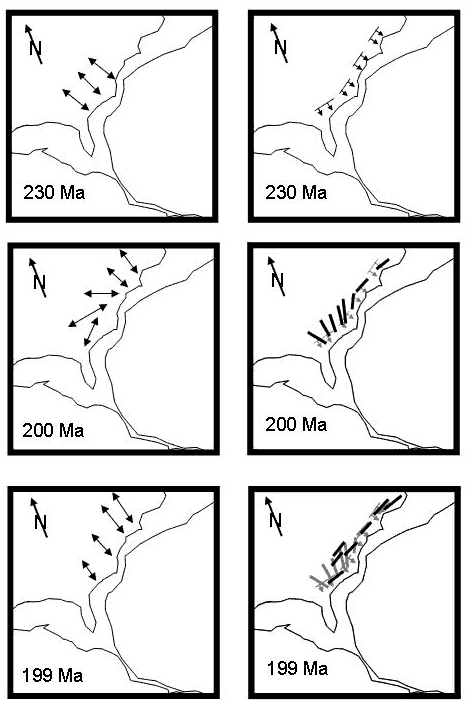
Figure 2: Stress evolution
based on observed extensional features from Beutel
(2009).
Left column shows the likely orientation
of extensional stress based on the observed features
in the right column. Right column shows the age and
orientation of extensional features observed in the
field. Top box in right column shows normal faults
while the middle and lower boxes show the overlying
dikes.
Modeling Stress Fields
To determine
the plausibility of tectonic changes being able
to cause rapid, 90° changes in the stress field
orientation throughout a continent, an evolving model
of Pangaea break-up was created using the finite-element
program FElt (Gobat & Atkinson,
1996). The models constructed were based on the
most likely tectonic scenarios. For example, it was
deemed unlikely that a single, large continent would
rotate in one direction for millions of years and
then switch and rotate through 90° in 2 Ma while
attached to at least two other large continents.
Consideration was also given to application of
the stresses as well as the physical properties of
the continents and their connections. Continental
stresses, those assumed to move large continents,
were applied to a generalized area associated with
the continental keel such that the motion driving
the continents would be best distributed over the
entire continent. Some models were run with only
marginal stresses applied, but these were to
be insufficient to move the entire continent and
would have resulted in the formation of extensional
margins along the outside edges of the continents.
The interiors of the continents were modeled as thicker
than the margins and the suture zones between the
continents were modeled as weaker than the surrounding
lithosphere. Models were run with the sutures the
same strength as the surrounding lithosphere, but
while similar, continent-scale stress fields were
successfully modeled, the lack of a defined suture
zone created a stress field that would likely not
have followed the actual breaks in the continents.
Model Results
Numerous
models with variations in plate motions and suture
locations and strengths were studied (Figure 3).
What follows is the most likely history of stress evolution
that explains how the continents move today and moved
in the past, and the 90° rotation in stress field
orientation within 2 Ma shown by the diabase dikes
associated with continental break-up.
At about
230 Ma North America began to move to the NW. This
created NE-trending extensional basins along the SE
margin of North America. Resistance to this motion
appears necessary to cause large-magnitude stresses
(compared to those applied). This resistance was simulated
by fixing Africa. It could also have been accomplished
by moving Africa to the SW and by having it resistant
to movement as a result of its massive keel. The
resulting stress field in SE North America is NW extensional,
which would result in NE-trending normal faults. These
are indeed seen in the geologic record (Figure 3A).
At ~ 200
Ma, when volcanism onset in SE North America, South
America began to move to the SW. This changed the stress
field orientation along the SE margin of North America
from NW extensional to NE extensional. The new stress
field results in NW-trending extensional features,
consistent with the NW-trending dike swarm along the
SE margin of North America. This also created an E-W
trending extensional regime north of the present-day
Gulf of Mexico, which resulted in the N-trending dikes
seen along that margin. The stress field varies close
to the meandering suture zone. This implies that pre-existing
variability in lithospheric strength affected the stress
field and can account for regional features on a scale
smaller than continental (Figure 3B).
At ~ 199
Ma South America separated from North America. This
is best modeled as the formation of oceanic crust and
a mid-ocean ridge. Fast- to medium-spreading ridges
are weak features and do not effectively transmit stress
across themselves. This results in the stress field
along the eastern margin of North America rapidly reverting
to NW extensional, successfully explaining the observed
NE-trending diabase dikes. The presence of N-trending
dikes between the two end-members is likely as the
stress field rotated between NE extensional and NW
extensional. Of note is the sudden decrease in extensional
stress along the southern margin of North America and
the increase in stress along the mid-southeast portion
of the margin. This change in the stress field agrees
with the lack of multiple dike orientations along the
southern margin and perhaps the sudden northward propagation
of the dikes and rifts into what is now the New England
margin of eastern North America (Figure 3C).
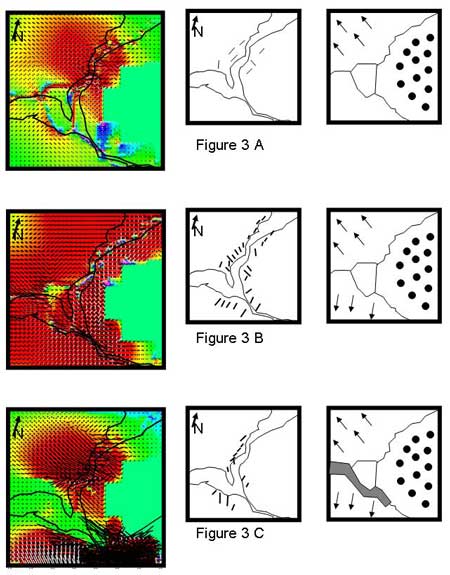
Figure 3: Left column images
are the results of the finite-element model. Background
colors indicate maximum stress magnitude and type (blue
- compressional, red - extensional), bars are the
maximum and minimum stress directions (black - extensional,
white - compressional). Middle column images show
the projected field-observable extensional features
created by this stress field. Right column images
show the applied stress fields. Black dots are elements
and nodes held fixed in space (modified from Beutel,
2009).
Conclusions
The
non-radial CAMP dike swarm associated with the break-up
of Pangaea requires a rapidly evolving orientation
of stress to account for the change from NE trending
normal faults at 230 Ma to NW trending diabase dikes
at ~200 Ma, followed by N-trending and then NE-trending
diabase dikes within 2 Ma, all of which overlap along
the southeast margin of North America. A plume scenario
cannot account for such an evolving stress pattern,
but tectonic events that are known to have occurred
can.
Finite-element models demonstrate
that the observed extensional features can be explained
by ongoing motion of North America to the NW in the
presence of a stationary Africa, followed by SW-motion of South
America, and then detachment and final separation
from North America at a mid-ocean ridge. The driving
force behind the magmatism is tectonic rather
than plume in origin, and the dikes cannot be used
to prove a plume source for the break-up of Pangaea.
References
-
Beutel, E.K., Nomade, S., Fronabarger,
A.K., Renne, P.R., 2005, Pangea’s complex
breakup: A new rapidly changing stress field model: Earth
Planet. Sci. Lett., 236,
471-485.
-
-
de Boer, J.Z., Snider, F.G, 1997, Magnetic and
chemical variations of Mesozoic diabase dikes from
eastern North America: Evidence for a hotspot in
the Carolinas?: Geol. Soc. Am. Bull., 90,
185-198.
-
Dalziel, I.W.D., Lawver, L.A., Murphy, J.B., 2000,
Plumes, orogenesis, and supercontinental fragmentation: Earth
Planet. Sci. Lett., 178, 1-11.
-
Ernst,
R.E., and Buchan, K.L., 1997, Giant radiating dyke
swarms; their use in identifying pre-Mesozoic large
igneous provinces and mantle plume. In Mahoney,
J.J.; Coffin, M.F. (Eds.), Large igneous provinces;
continental, oceanic, and planetary flood volcanism.
Geophysical Monograph 100, 297-333.
-
Gobalt J.L. and
Atkinson D.C., 1996, FElt users guide and reference
manual;
San Diego, San Diego Press, University of San Diego,
234 pp.
-
Golonka, J., 2007, Late Triassic and Early
Jurassic palaeogeography of the world: Palaeogeography,
Palaeoclimatology, Palaeoecology, 244,
297-307.
-
Hames, W.E., Renne, P.R., and Ruppel, C.,
2000, New evidence for geologically instantaneous
emplacement of earliest Jurassic Central Atlantic
magmatic province basalts on the North American
margin: Geology, 28,
859-862.
-
Janney, P.E., P.R. Castillo,
2001, Geochemistry of the oldest Atlantic oceanic
crust suggests mantle plume involvement in the
early history of the central Atlantic Ocean: Earth
Planet. Sci. Lett., 192, 291-302.
-
Johnson, R.B., 1961, Patterns and origin of radial
dike swarms associated with West Spanish peak and
Dike Mountain, South Central Colorado: Geological
Society of America Bulletin, 72, 579-590.
-
King,
S.D., D.L. Anderson, 1998, Edge-driven convection: Earth
Planet. Sci. Lett., 160,
289-296.
-
Marzoli, A., Renne, P.R., Piccirillo, E.M.,
Ernesto, M., Bellieni, G., and De Min, A., 1999,
Extensive 200 Million-Year-Old Continental Flood
Basalts of the Central Atlantic Magmatic Province: Science, 284,
616-618.
-
May, P.R.. 1971, Pattern of Triassic-Jurassic
diabase dikes around the North Atlantic in the context
of the predrift positions of the continents: Geological
Society of America Bulletin, 82,
1285-1292.
-
McHone, J. G., 2000, Non-plume magmatism
and tectonics during the opening of the central
Atlantic Ocean: Tectonophysics, 316,
287-296.
-
McHone, J.G., 1988, Tectonic
and paleostress patterns of Mesozoic intrusions
in eastern North America. In Manspeizer, W.
(Ed.),Triassic-Jurassic
Rifting: Continental Breakup and the Origins of
the Atlantic Ocean and Passive Margins:
Elsevier, New York, pp 607-
620.
-
McHone, J. G., Ross, M. E.,
Greenough, J. D., 1987, Mesozoic dyke swarms of
Eastern North America. In Halls, H.C.; Farhig,
W.F. (Eds.), Mafic dyke
swarms.
Geol. Ass. Can. Spec. Pap. 34, 279-288.
-
Nomade, S.;
Knight, K B; Beutel, E; Renne, P R; Verati, C.;
Feraud, G.; Marzoli, A; Youbi, N; Bertrand, H.,
2007, Chronology of the Central Atlantic Magmatic
Province; implications for the central Atlantic
rifting processes and the Triassic-Jurassic biotic
crisis: Palaeogeography,
Palaeoclimatology, Palaeoecology, 244,
326-344.
-
Ragland, P.C, 1991, Mesozoic
Igneous Rocks, in The Geology
of the Carolinas.
In Horton, J.W.; Zullo, V.A. (Eds.), Carolina Geological
Society Fiftieth Anniversary Volume 171-190.
-
Ragland, P.C., Hatcher,
R D., Jr., Whittington, D., 1983. Juxtaposed Mesozoic
diabase dike sets from the Carolinas: Geology, 11,
394-399.
-
Salters, V. J. M, Ragland, P.C,
Hames, W.E, Milla, K., and Ruppel, C., 2003, Temporal
chemical variations within lowermost Jurassic tholeiitic
magmas of the Central Atlantic Magmatic Province.
In Hames, W.E; McHone, J.G.; Renne, P.R; Ruppel,
C. (Eds.), The
Central Atlantic Magmatic Province: Insights
from fragments of Pangea: Geophysical Monograph
136, 163-177.
-
Schlische, R.W., M.O. Withjack,
P.E. Olson, 2003, Relative timing of CAMP, rifting,
continental breakup, and basin inversion; tectonic
significance. In Hames, W.E; McHone, J.G.; Renne,
P.R; Ruppel, C. (Eds.), The Central Atlantic
Magmatic Province: Insights from fragments of Pangea:
Geophysical Monograph 136, 33-59.
-
Schlische, R.W.,
2002, Progress in understanding the structural
geology, basin evolution, and tectonic history
of the eastern North American rift system. In LeTourneau,
P.M.; Olsen, P.E. (Eds.), The Great Rift
Valleys of Pangaea in Eastern North America,
Vol. 1: New York, Columbia University Press.
-
Storey, B.C.,
Leat, P.T., and Ferris, J.K., 2001, The location
of mantle-plume centers during the initial stages
of Gondwana breakup. In Ernst, R.E.; Buchan,
K.L. (Eds.), Mantle Plumes: Their Identification
through Time:
Geological Society of America, Special Paper 352,
71-80.
-
Van Wijk, J.W., Huismans, R.S., ter Voorde,
M., Cloetingh, S.A.P.L., 2001, Melt Generation
at Volcanic Rifted Margins: no Need for a Mantle
Plume: Geophysical
Research Letters, 28, 3995-3998.
-
Wilson, M., 1997, Thermal evolution of the Central
Atlantic passive margins: Continental break-up above
a Mesozoic super-plume: Journal of the Geological
Society (London), 154, 491-495.
-
Ziv, A., Rubin, A.M., Agnon, A., 2000, Stability
of dike intrusion along preexisting fractures: Journal
of Geophysical Research, 105, 5947-5961.
last updated 20th
June, 2009 |