 |
Implications
for melt in the upper mantle from the electrical
conductivity of melt-bearing peridotites at
high pressures |
Takashi Yoshinoa, Mickael Laumonierb,
Elizabeth McIsaacc & Tomoo Katsuraa
aInstitute for Study of
the Earth's Interior, Okayama University, Misasa, Tottori
682-0193, Japan, tyoshino@misasa.okayama-u.ac.jp ; Tomo.Katsura@uni-bayreuth.de
bInstitut des Sciences de
la Terre d'Orléans,
UMR 6113, Campus Géosciences, 1A, Rue de la
Férollerie, 41071 Orléans cedex 2, France, mickael.laumonier@univ-orleans.fr
cDepartment of Earth Sciences,
Dalhousie University, Edzell Castle Circle, Halifax
NS, Canada B3H 4J1, EL997655@dal.ca
This webpage is a summary of: Takashi,
Y., M. Laumonier, E. McIsaac & T. Katsura, Electrical
conductivity of basaltic and carbonatite melt-bearing
peridotites at high pressures: Implications for melt
distribution and melt fraction in the upper mantle, Earth
Planet. Sci. Lett., 295, 593-602,
2010.
Laboratory measurements on anhydrous
peridotite and single crystals of dry olivine show
that the electrical conductivity of the upper mantle
should be ~10-4 to 10-2 S/m at
mantle temperatures. Electromagnetic observations,
however, have demonstrated that the electrical conductivity
of some upper mantle regions greatly exceeds these
values. In the mantle under the Pacific Ocean, just
beneath or close to the mid-ocean ridge, conductivities
of >10-1 S/m
have been measured at depths of > 60 km (Evans
et al., 2005). On the other hand, the deep electrical
conductivity profile, obtained from analysis of data
from a submarine cable extending from Hawaii to North
America, showed a conductivity peak of 10-1 S/m in
the depth range 200~250 km (Lizaralde et al.,
1995).
Highly conductive zones in the upper
mantle require the presence of conductive phases, and
silicate melts or hydrated olivine crystals are commonly
considered. High mantle conductivities have usually
been interpreted as indicating trace amounts of hydrogen
in olivine. The magnitude of the effect of water on
olivine conductivity remains under debate (Wang
et al., 2006; Yoshino et al., 2006).
However, two recent pieces of work indicate that although
the presence of water greatly enhances the electrical
conductivity of olivine this effect is not large enough
to explain the high conductivity at the top of the
asthenosphere as observed at the East Pacific Rise
(Yoshino et al., 2009; Poe et al.,
2010).
Silicate melt is another candidate
to explain conductivity anomalies in the upper mantle.
However, conductivity measurements on dry olivine and
basaltic melt by Tyburczy & Waff (1983)
suggested that a 5-10% melt fraction is required to
explain 0.1 S/m based on the Hashin and Shtrikmann
upper bound¶, which assumes an ideal
geometry. Such a high melt fraction is not consistent
with estimates from seismological studies (e.g., The
MELT seismic team, 1998). This discrepancy may
be caused by a difference between the ideal geometry
and a realistic partial-melt geometry.
Recently Gaillard et al. (2008)
measured the conductivity of carbonatite melts at atmospheric
pressure, and demonstrated that carbonatite melt has
a distinctly higher conductivity than silicate melt. Gaillard
et al. (2008) suggested that a very small amount
of carbonate melt is enough to explain the conductivity
anomaly at the top of the asthenosphere. To assess
this suggestion, we thus need to know the effect of
carbonatitic melt on the bulk conductivity of partially
molten rocks under high pressure.
In our study, we performed electrical
impedance measurements on two types of partially molten
samples with basaltic and carbonatitic melts in a Kawai-type
multi-anvil apparatus in order to investigate melt-fraction
vs. conductivity relationships and melt distribution
in partially molten peridotite under high pressure.
The silicate samples comprised San Carlos olivine with
various amounts of mid-ocean ridge basalt (MORB). The
carbonate samples were a mixture of San Carlos olivine
with various amounts of carbonatite. High pressure
experiments on the silicate and carbonate systems were
performed up to 1600 K at 1.5 GPa and up to at least
1650 K at 3 GPa, respectively.
Sample conductivity increased with
increasing melt fraction. Carbonatite-bearing samples
show approximately one order of magnitude higher conductivity
than basalt-bearing ones at a similar melt fraction.
Comparison of the electrical conductivity data with
theoretical predictions for melt distribution indicates
that the model assuming that the grain boundary is
completely wetted by melt is the most preferable melt
geometry. The gradual change of conductivity with melt
fraction suggests no permeability jump due to melt
percolation at a certain melt fraction. The melt fraction
of the partial molten region in the upper mantle can
be estimated to be 1 to ~3% and ~0.3% for basaltic
melt and carbonatite melt, respectively.
Just beneath the mid-ocean ridge, the
melt fractions required to explain the conductivity
of 0.1 S/m are 1 and 3 vol.% at 1600 and 1500 K, respectively.
This range of melt fraction is consistent with the
minimum melt concentration of 1 to 2 vol.% in the melt
production region predicted from seismic shear-wave
delays and Rayleigh-wave velocity variations (The
MELT seismic team, 1998). Therefore, the conductivity
anomaly in this region can be explained by a presence
of silicate melt.
Hirschmann (2010) suggested
that carbonatite melt can be stable below depths of
130-180 km for mantle peridotite containing typical
amounts of volatile components. Melting beneath the
mid-ocean ridge occurs at depths up to 330 km, producing
0.03-0.3 wt.% carbonatite melt. Our estimated melt
fraction for carbonatite-bearing peridotite falls in
this range. The deep electrical conductivity profile,
obtained from analysis of data from a submarine cable
extending from Hawaii to North America, showed a conductivity
peak of 10-1 S/m in the depth range 200~250
km (Lizaralde
et al., 1995). Such a conductive anomaly can be
explained by a presence of very small amount of carbonatite
melt (Figure 1).
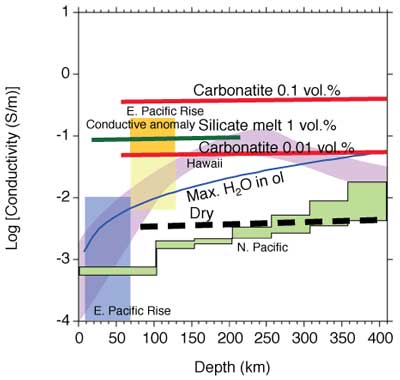
Figure 1: Comparison of laboratory
data on electrical conductivity of carbonatite melt,
silicate melt and hydrous olivine with geophysically
observed electrical conductivity in the upper mantle
beneath the Pacific ocean. Red and green lines: electrical
conductivity of carbonatite-bearing and silicate-melt-bearing
peridotites respectively, blue line: upper bound
of the electrical conductivity of hydrous olivine
based on the maximum hydroxyl solubility in olivine
(Yoshino et al., 2009), thick black dashed line:
electrical conductivity of dry olivine (Xu et al.,
1998), green and pink areas: geophysically observed
conductivity profile in the Pacific ocean (Utada
et al., 2003 and Lizzaralde et al., 1995), orange
and light-orange regions: conductivity around a depth
of 100 km near the East Pacific Rise, light blue
area: the range of conductivity
above 60 km near the East Pacific Rise (Evans et
al., 2005). The conductivity anomaly beneath Hawaii
can be explained by a very small amount
of carbonatite melt.
¶Rocks are usually
multi-phase. To predict the effective properties as
a function of volume fraction of the various phases,
the best approach is to predict the upper and lower
bounds theoretically. The best bounds, defined as giving
the narrowest possible range without specifying the
geometries of the constituents, are the Hashin-Shtrikman
bounds. The upper bound indicates the case where the
conductive phase is the best interconnected.
References
-
Evans, R.L., Hirth,
G., Baba, K., Forsyth, D., Chave, A., Mackie, R., 2005,
Geophysical evidence from the MELT area for compositional
controls on oceanic plates, Nature, 437,
249–252.
-
Gaillard, F., Marki, M., Iacono-Marziano,
G., Pichavant, M., Scaillet, B., 2008, Carbonatite
melts and electrical conductivity in the asthenosphere, Science, 322,
1363-1365.
-
Hirscmann, M.M., 2010, Partial
melt in the oceanic low velocity zone, Phys.
Earth Planet. Int., 179,
60-71.
-
Lizarralde, D., Chave, A.D.,
Hirth, G., Schultz, A., 1995, A Northern Pacific
mantle conductivity profile from long-period magnetotelluric
sounding using Hawaii to California submarine cable
data, J. Geophys.
Res., 100, 17837-17854.
-
Poe, B.T., Romano, C., Nestola,
F., Smyth, J.R., 2010, Electrical conductivity
anisotropy of dry and hydrous olivine at 8 GPa, Phys.
Earth Planet. Int., doi:10.1016/j.pepi.2010.05.003
-
The MELT
seismic team, 1998, Imaging the deep seismic structure
beneath a mid-ocean ridge: The MELT experiment, Science, 280,1215-1218.
-
Tyburczy,
J.A., Waff, H.S., 1983, Electrical conductivity
of molten basalt and andesite to 25 kilobars pressure:
geophysical significance and implications for charge
transport and melt structure, J. Geophys. Res., 88,
2413-2430.
-
Utada, H., Koyama, T., Shimizu,
H., et al., 2003, A semi-global reference model
for electrical conductivity in the mid-mantle beneath
the north Pacific region, Geophys.
Res. Lett.,
31, 1194, doi:10.1029/2002GL016902
-
Wang, D., Mookherjee, M., Xu,
Y., Karato, S., 2006, The effect of water on the
electrical conductivity of olivine, Nature, 443,
977–980.
-
Yoshino, T., Matsuzaki, T.,
Yamashita, S., Katsura, T., 2006a, Hydrous olivine
unable to account for conductivity anomaly at the
top of the asthenosphere, Nature, 443,
973-976.
-
Xu, Y., Poe, B.T., Shankland,
T.J., et al., 1998, Electrical conductivity of
olivine, wadsleyite and ringwoodite under upper-mantle
condition, Science, 280,
1415-1418
-
Yoshino, T., Yamazaki, D., Mibe,
K., 2009, Well-wetted olivine grain boundaries
in partial molten peridotites in the asthenosphere, Earth
Planet. Sci. Lett., 283,
167-173.
last updated 8th
July, 2010 |