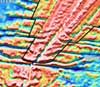 |
Marine Geophysical Survey of the Active Reykjanes Ridge Reorganization Tip – Implications for the Radially Expanding Iceland Plume Model |
Richard Heya, Fernando Martineza, Ármann Höskuldssonb, Deborah E. Easonc, Jonathan Sleeperc, Sigvaldi Thordarsond, Ásdís Benediktsdóttirb,d, Sergey Merkuryeve
aHawaii Institute of Geophysics & Planetology, School of Ocean & Earth Science & Technology, University of Hawaii at Manoa, Honolulu, Hawaii, USA. hey@soest.hawaii.edu ; fernando@hawaii.edu
bInstitute of Earth Sciences, University of Iceland, Reykjavik, Iceland. armh@hi.is ; asb1@hi.is
cDepartment of Geology & Geophysics, School of Ocean & Earth Science and Technology, University of Hawaii at Manoa, Honolulu, Hawaii, USA. deborahe@hawaii.edu ; jsleeper@hawaii.edu
dIceland GeoSurvey, Reykjavik, Iceland. sthor@isor.is
ePushkov Institute of Terrestrial Magnetism, Ionosphere & Radio Wave Propagation of the Russian Academy of Sciences St.-Petersburg Filial, St. Petersburg, Russia, & Saint Petersburg State University, Institute of Earth Sciences, St. Petersburg, Russia. sam@ns1480.spb.edu
This webpage is a summary of: Hey, R., F. Martinez, Á. Höskuldsson, D.E. Eason, J. Sleeper, S. Thordarson, Á. Benediktsdóttir, and S. Merkuryev, Multibeam investigation of the active North Atlantic plate boundary reorganization tip, Earth Planet. Sci. Lett., 435, 115-123, http://dx.doi.org/10.1016/j.epsl.2015.12.019, 2016.
Introduction
There have been three distinct phases of seafloor spreading along the Mid-Atlantic Ridge (MAR) south of Iceland, as shown by changes in plate boundary configuration in both gravity maps (Figure 1) and magnetic anomaly maps (Figure 2) (e.g., Vogt, 1971; White, 1997). Immediately after Greenland - Eurasia breakup at ~55 Ma, an initial ridge system without transform faults was established, forming continuous magnetic and gravity lineaments nearly parallel to the continental margins. This pattern changed approximately synchronously at ~37 Ma (Jones, 2003) into a more typical slow-spreading orthogonal ridge/transform pattern. This probably occurred because of a nearly instantaneous change in spreading direction from ~125° to ~100° caused by the coupling of Greenland to North America (e.g., Vogt, 1971), although nearly instantaneous plume-related mechanisms have also been suggested (e.g., Howell et al., 2014). Almost immediately after that initial reorganization the most recent major reorganization of this plate boundary initiated south of Iceland and continues at present. The orthogonal North America-Eurasia ridge/transform staircase geometry is progressively changing to the present non-segmented oblique spreading geometry on the Reykjanes Ridge as transform faults are successively eliminated. The boundary wakes of this diachronous seafloor spreading reorganization are difficult to reconcile with radially expanding Iceland plume models.
Figure 1: Satellite-derived gravity (Sandwell et al., 2014) and tectonic interpretation. White dashes approximate Reykjanes Ridge axis. Heavy black step-like V-pattern is interpreted as diachronous, transform-eliminating, reorganization boundary. Light dashed lines: E scarps of Vogt (1971); approximately E-W black lines: old (named) FZs. The V-shaped ridges, troughs and scarps (VSRs) are the structures slightly oblique to the Reykjanes Ridge axis enclosed by the reorganization boundary. Bight is the first transform fault remaining south of Iceland, Modred is now a migrating non-transform offset (NTO). Thin black V-shaped lines extending from reorganization boundaries toward the ridge axis are wakes of migrating NTOs. Black dots show propagator wake independent of Reykjanes reorganization. Click here or on Figure for enlargement.
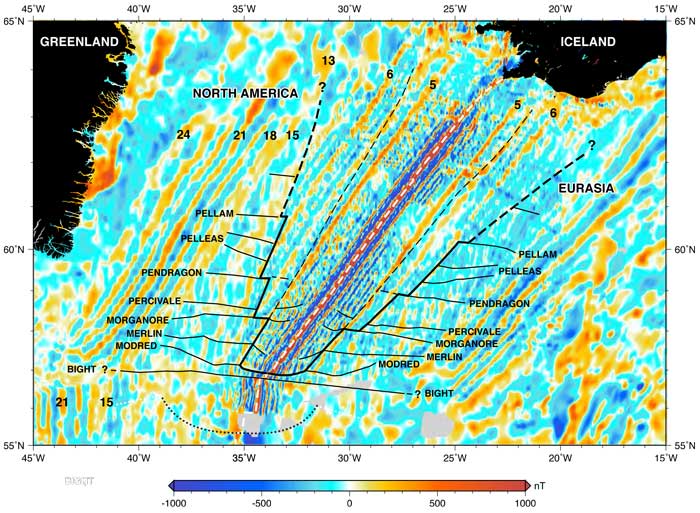
Figure 2: Compiled magnetic data from the Geological Survey of Canada (Macnab et al., 1995) merged with RRS Charles Darwin (CD87) data (Searle et al., 1998), 2007 R/V Knorr cruise data (Hey et al., 2010) and our 2013 R/V Langseth data. Major chrons numbered; tectonic boundaries from Figure 1. Click here or on Figure for enlargement.
Alternative models for the ongoing transform-eliminating reorganization
The generally accepted model (White, 1997; Jones, 2003) proposes that this latest spreading-center reorganization results from a thermally induced change in lithospheric behavior from the previous brittle rheology, producing the orthogonal ridge/transform fault pattern, to a ductile rheology producing the oblique unsegmented ridge pattern. The change from brittle to ductile behavior occurs as a critical Iceland plume head isotherm progressively interacts with the ridge segments, eliminating transform faults and reorganizing the geometry from orthogonal to oblique. White (1997) and Jones (2003) propose that the limit of the transform fault eliminations corresponds to the transition from axial ridge to axial valley, supporting a thermal model, as both these phenomena were thought to coincide with the onset of ductile behavior at the reorganization tip, near 58°N. However, modern data show that there is a ~200 km separation between the transition from axial high to axial valley (at 58.8°N, Keeton et al., 1997) and the most recent transform fault elimination near 57°N (Figure 1; Sandwell et al., 2014). This weakens this argument. In addition, this is the only place on the global spreading plate boundary where such a thermal reorganization mechanism is generally accepted.
In all other areas of progressive plate boundary reorganization, including several other hotspot-affected areas, the accepted mechanism is tectonic rift propagation (e.g., Hey, 2001). This includes the Juan de Fuca, Northeast Pacific, Galapagos, and East Pacific Rise microplates, the Southeast Indian Ridge, Pacific-Antarctic Ridge, Chile Rise, and elsewhere along the MAR. On Iceland itself it is also the accepted mechanism of rift relocation. South of Iceland, the small Reykjanes Ridge jumps modeled magnetically (Benediktsdóttir et al., 2012; Hey et al., 2010) occur in patterns consistent with rift propagation, and explain the observed pattern of progressively developed asymmetric accretion (Figure 2). However, there are significant differences between those other reorganizations and the Reykjanes Ridge reorganization. In typical rift propagation a more orthogonally-spreading ridge propagates through preexisting lithosphere to replace a more obliquely-spreading axis. On the Reykjanes Ridge it is the obliquely-spreading ridge that is replacing the orthogonal one. Additionally, along the Reykjanes Ridge the propagators and ridge jumps proposed have generally been small-offset, usually 7 km or less, and within the ~15 km wide plate boundary zone which is generally assumed to be characterized by non-rigid plate behavior (e.g., Macdonald, 1982). This contrasts with classic propagators that break through rigid plates well outside the plate boundary zone.
Thus, whether the mechanism is thermal or tectonic, the Reykjanes Ridge reorganization appears to be unique. To determine exactly how this reorganization is occurring we conducted a marine geophysical survey surrounding our hypothesized active reorganization tip, including the northernmost transform fault remaining south of Iceland, the Bight transform (Figure 1; Sandwell et al., 2014) and the three most recent transform eliminations north of Bight. The reorganization boundaries in Figures 1 and 2 are strongly constrained by our new data between the Morganore fracture zone (FZ) and the reorganization tip (Figures 3-5).
Unfortunately the only high-resolution data along any part of the reorganization boundaries are our new data near the tip. North of this the boundaries have been drawn in several ways. We agree with interpretations that include reorganization boundaries with offsets at various FZs (e.g., Vogt & Johnson, 1975; Merkuryev et al., 2009; Poore et al., 2009; Parnell-Turner et al., 2014) consistent with the interpretation that this reorganization had trouble getting past some transform faults (Vogt & Johnson, 1975). Our reorganization boundaries were based on the transform eliminations and thus were constrained to go through our interpretations of the terminations of the approximately E-W FZ structures (Figure 1). Narrow straight lines drawn between these points, with step-like offsets at the Pellam, Pendragon, and Morganore FZs (all names from Vogt & Avery, 1974) enclose the diachronous V-shaped ridges, troughs and scarps (VSRs) flanking the Reykjanes Ridge (Vogt, 1971).
Figure 2 shows that these simple straight-line reorganization boundaries, interpreted from the gravity data, provide a good overall fit to the regional magnetic anomaly pattern. They separate younger oblique from older orthogonal patterns and the reorganization boundaries are the same ages at conjugate positions, as required. If these boundaries were known exactly the reorganization history would be precisely defined, but to the extent they remain interpretive different reorganization models remain possible. For example, if the boundaries were “U-shaped” curves they would indicate the slowing of progressive reorganization and be consistent with radial plume flow models which must slow as 1/r away from the melting anomaly in order to conserve mass (Vogt, 1971; White & Lovell, 1997).
New results
Our 33-day marine geophysical survey was conducted in August-September 2013 on the R/V Marcus G. Langseth. The new EM122 multibeam data (Figure 3), together with shipboard and satellite-derived free-air gravity data (Figure 4), show a monotonic sequence of FZ terminations from north to south of the Pendragon, Morganore, Merlin and Modred FZs. This survey, combined with previous data (Keeton et al., 1997; Searle et al., 1998; E. Kjartansson, pers. comm., 2007; Höskuldsson et al., 2007; Hey et al., 2010), completes the multibeam mapping of the oblique Reykjanes Ridge axis and shows definitively that transform faults no longer exist between the Bight transform and Iceland. Just north of the Bight transform, the Modred transform has evolved to a NTO (Figure 5). From there to Iceland there is a continuous oblique ridge axis without transform offsets (Figure 1). South of the Bight transform there are numerous MAR transform faults and orthogonal seafloor spreading (Sandwell et al., 2014). This is the pattern expected if the transform-eliminating reorganization tip is presently at the Modred NTO near 57°N, just north of the Bight transform.
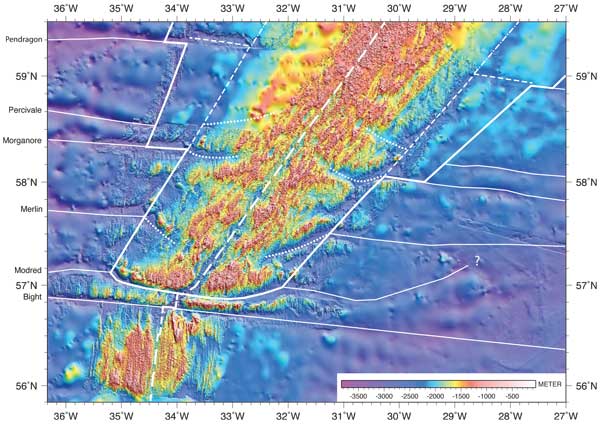
Figure 3: EM122 multibeam bathymetry collected on R/V Langseth in 2013, combined with British EM12 multibeam data (Keeton et al., 1997) north of our survey, surrounded by predicted bathymetry from Smith and Sandwell (1997). Heavy white step-like transform-eliminating reorganization boundaries based on FZ terminations as in Figure 1. Dash-dot lines north of Pendragon are E scarps from Figure 1. FZs (thin white lines) and NTO wakes (dotted white lines) as in Figure 1. Thick white dashed lines are simplified spreading axes.
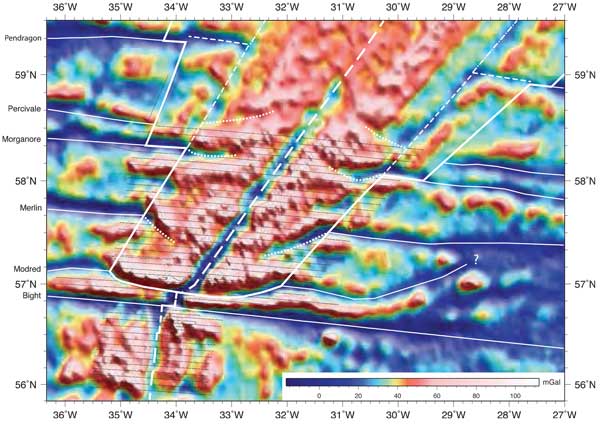
Figure 4: R/V Langseth free-air gravity data (ship tracks shown by fine dotted black lines, dots indicate positions of gravity measurements) merged with satellite-derived gravity (Sandwell et al., 2014) outside mosaic survey area. Tectonic boundaries as in Figure 3.
The EM122 backscatter data (Figure 5) show that the oblique Reykjanes Ridge axis (high backscatter) north of Modred curves to overlap with the tip of the still orthogonally-spreading axis between the Modred NTO and the Bight transform. There is no transform fault at the Modred offset at present, but rather an ~10-km-long NTO between curving overlapping ridge segments as seen at overlapping spreading centers elsewhere (e.g., Macdonald et al., 1988). The pattern of curved faults extending away from the overlap zone shows a long-term history of the curvature of the axes toward each other.
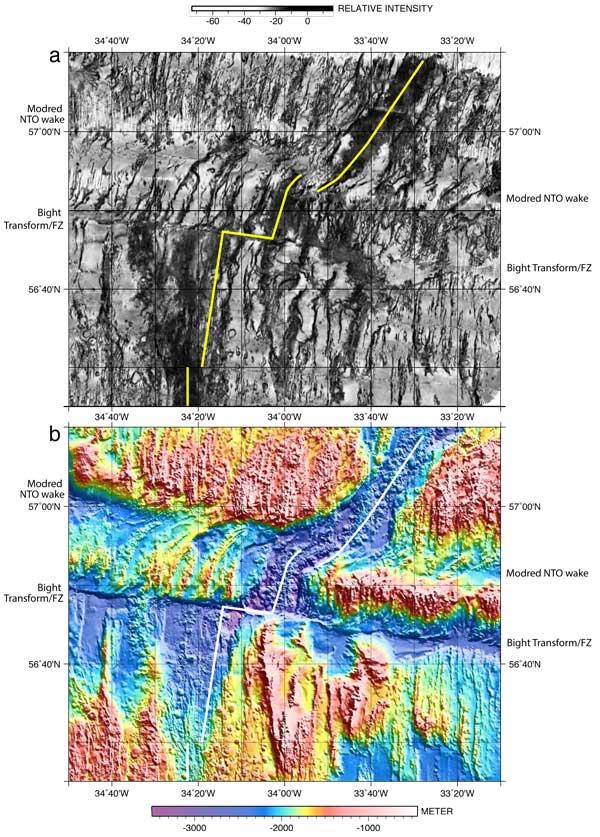
Figure 5: (a) R/V Langseth EM122 acoustic backscatter mosaic over reorganization tip. High relative intensities are dark. Yellow lines approximate the center of most recent axial volcanism, the pattern south of Bight is from Benediktsdóttir et al. (2016). The Modred NTO bounds the oblique Reykjanes Ridge axis. It has had an overlapping spreading geometry for the past several Ma, during which the offset migrated slowly south. The linear Bight transform fault offsets orthogonally-spreading ridge segments. (b) R/V Langseth EM122 multibeam bathymetry over same area, showing axial graben pattern, Bight transform fault stability, and Modred NTO migration wake.
Although the exact locations of the terminations of the ~E-W FZs are somewhat interpretive, narrow straight-line reorganization boundaries can be drawn through reasonable places (Figures 3-4). Our new multibeam mosaic between ~58.5°N and ~56°N covers the elimination of the Morganore, Merlin and Modred transforms on both plates, and the Percivale transform on the North American plate. The young reorganization boundaries extending to the reorganization tip are thus strongly constrained. In addition, a brief survey of the Pendragon FZ termination on the North American plate during our transit helps constrain the older reorganization history. On the Eurasian plate, the Pendragon elimination is partly constrained by RRS Charles Darwin multibeam data (Figure 3; Keeton et al., 1997; Searle et al., 1998) and gravity data (Figure 4; Sandwell et al., 2014).
The terminations of the Pendragon, Morganore, Merlin and Modred FZs are essentially co-linear (Figures 3-4). They indicate that the transform-elimination reorganization propagated through our mosaic survey area at a constant velocity of ~110 km/Myr until it intersected the Modred transform. There, propagation abruptly slowed and the transform offset was converted into the very slowly southward-migrating NTO that exists today at the reorganization tip (Figure 5).
Notably, these youngest transform-elimination boundaries occur along essentially linear extensions of the VSR E scarps (dashed lines between Pendragon and the Iceland shelf in Figure 1; dashed-dot lines north of Pendragon in Figures 3-4) discovered by Vogt (1971). The E scarps and other VSRs were originally postulated to be symmetric plume pulse wakes (Vogt, 1971; White, 1997). Later they were shown to be asymmetric (Jones et al., 2002; Hey et al., 2010) and interpreted as ridge-jump-boundary pseudofaults (Hey et al., 2010; Benediktsdóttir et al., 2012) based on detailed magnetic modeling between 62°N and Iceland. The direct linkage of the FZ terminations near the reorganization tip with the E scarps suggests that the Pendragon, Morganore, Merlin and Modred transform eliminations and the E-scarp VSR formation involved the same process, whatever that may be.
The transform-elimination process led to distinctive, non-flowline, broad, V-shaped gravity and bathymetry troughs extending from the Morganore, Merlin and Modred FZ terminations (dotted lines in Figures 3-4). These have considerably different azimuths from the ~E-W flowline FZ troughs, and from the VSR troughs closer to Iceland that subtend much more acute angles (Figure 1). We interpret these as wakes of slowly migrating NTOs that evolved from the transform/FZ troughs. They are analogous to V-shaped pseudofault troughs characteristic of propagator wakes documented where the ridge jumps are bigger and the magnetic anomalies are easier to understand. Such structures are not explained by current thermal models, and incompletely explained by the simple propagating rift model, which predicts that a distinct failed rift or pseudofault graben should exist at each FZ termination.
Discussion
The Pendragon, Morganore, Merlin and Modred transform-elimination ages are consistent with the times when the Loki propagator, identified near Iceland, would have intersected these transforms if it propagated away from Iceland at a constant velocity of ~110-120 km/Myr following initial more rapid propagation as it left Iceland at ~15 Ma (Benediktsdóttir et al., 2012). In this interpretation, the E scarps that are linear extensions of these transform eliminations would be pseudofault wakes. (In the thermal interpretation the E-scarp VSRs would have formed from plume pulses and their asymmetry would require an additional mechanism such as rift propagation or asymmetric spreading.) The asymmetry of the reorganization boundaries, V-shaped structural troughs extending from the FZ eliminations, and numerous NTO migrations, suggest that rift propagation was involved in this reorganization. However, it appears that this propagation must be significantly different than in other areas, and appears to be occurring on several different scales, including a regional scale (with pseudofaults expressed as VSR boundaries) and a local scale (with pseudofaults expressed as migrating NTO wakes).
White & Lovell (1997) and Jones et al. (2014) proposed that the VSR pattern extends well south of the Bight transform. However, our multibeam mosaic data show transform-orthogonal abyssal hill structures south of the Bight transform with no indication of oblique VSRs extending into this area (Figure 3). We think that the structures south of the Bight transform interpreted by White & Lovell (1997) to indicate dramatic VSR curvature and 1/r radial flow slowing at a reorganization tip near 55°N (black dots in Figure 1) are instead pseudofaults from an independent propagator, not part of the oblique Reykjanes reorganization, and similar to several other independent propagators identified farther south along the MAR. That propagator, with another possibly following behind, appears to have been slowly propagating south for ~20 Ma after eliminating a ridge offset near 56°N (white dots in Figure 1).
The transform eliminations and VSR formations appear to result from the same process. None of the published models explains all of the observations. The broad V-shaped troughs extending away from the recent FZ terminations (Figures 3-4) are more compatible with propagating rift models than thermal models, and suggest that NTO formation and migration are an important part of the reorganization process. The observation that the reorganization stopped or greatly slowed at transform offsets appears to be incompatible with models in which deep plume flow under a thick viscous dehydration layer (invoked to prevent otherwise unrealistic crustal thicknesses predicted by the inferred fast plume upwelling; Ito, 2001) causes the reorganization. This is because such deep plume flow presumably would not be affected by lithospheric transform offsets.
Neither the propagating rift model nor the thermal model for the transform fault eliminations provides an obvious explanation for why the Reykjanes Ridge should be spreading obliquely. However, Martinez et al. (2015) show that the present Reykjanes Ridge axis, reconstructed by the Greenland – Eurasia finite rotations of Smallwood & White (2002), is nearly exactly congruent with the unsegmented magnetic anomalies formed just after Greenland – Eurasia breakup, strongly suggesting that the reorganization is reestablishing the original continental margin-parallel seafloor spreading geometry. They propose a new conceptual model involving propagating buoyant mantle upwelling that does provide an explanation for the Reykjanes Ridge obliquity and may ultimately prove successful.
Conclusions
A regional diachronous reorganization of the North Atlantic seafloor spreading geometry is currently occurring. The previous orthogonal North America-Eurasia ridge/transform staircase geometry is being progressively changed to the non-segmented oblique spreading geometry on the Reykjanes Ridge as transform faults are successively eliminated. New marine geophysical data over the reorganization tip boundaries reveal the recent reorganization history and indicate that neither the simple thermal model nor the simple propagating rift model explains all of the observations.
Our new data show rapid sequential eliminations of the Pendragon, Morganore and Merlin transform faults followed by abrupt reorganization slowing at Modred as that transform was shortened by some form of asymmetric spreading and converted to a slowly-migrating NTO. The youngest FZ terminations occur along linear extensions of the VSR E scarps discovered by Vogt (1971) and interpreted by Benediktsdóttir et al. (2012) as pseudofault wakes of the Loki propagator that left Iceland ~15 Ma.
The data suggest the following reorganization history. Beginning at ~35 Ma an obliquely-spreading Reykjanes Ridge axis began extending southwest from Iceland. At ~22 Ma the reorganization temporarily stopped at the Pellam transform fault for ~2 Ma. Continuous reorganization resumed at ~20 Ma, extending past Pellam to Pendragon and eliminating the Pelleas north and south transforms (or NTOs). There was then a long reorganization pause at the Pendragon transform beginning at ~18 Ma. In our preferred model continued reorganization at ~60 km/Myr eliminated Pendragon at ~15 Ma and then Percivale at ~13.5 Ma, but it then paused at the Morganore transform at ~13 Ma with the youngest sections of Pendragon formed as a very slowly migrating NTO or zero-offset FZ. Subsequent reorganization caused by the Loki propagator then eliminated Pendragon at ~11 Ma, Morganore at ~10 Ma, and Merlin at ~9 Ma at an approximately constant along-ridge velocity of ~110 km/Myr, before slowing at Modred, the present location of the reorganization tip, at ~8.5 Ma. Between pauses at FZs the diachronous reorganization boundaries appear to be straight instead of showing the 1/r curvature that radially expanding plume-ridge interaction would predict. We see no structural evidence that the VSRs extend south past the Bight transform.
This work was supported by NSF grant OCE-1154071.
References
-
Benediktsdóttir, Á., R. Hey, F. Martinez, and Á. Höskuldsson (2012), Detailed Tectonic Evolution of the Reykjanes Ridge During the Past 15 Ma, Geochem. Geophys. Geosyst., 13, Q02008, doi:02010.01029/02011GC003948.
-
Benediktsdóttir, Á., R. Hey, F. Martinez, and Á. Höskuldsson (2016), A new kinematic model of the Mid-Atlantic Ridge between 55°55'N and the Bight transform fault for the past 6 Ma, J. Geophys. Res., 121, doi:10.1002/2015JB012504.
-
Hey, R.N. (2001), Propagating Rifts and Microplates, in Encyclopedia of Ocean Sciences, eds. J.H. Steele, S.A.Thorpe, and K.K. Turekian, Academic Press, London, Volume 4, 2300-2308.
-
Hey, R., F. Martinez, Á. Höskuldsson, and Á. Benediktsdóttir (2010), Propagating rift model for the V-shaped ridges south of Iceland, Geochem. Geophys. Geosyst., 11, Q03011, doi: 10.1029/2009GC002865.
-
Höskuldsson, Á., R. Hey, E. Kjartansson, and G. B. Gudmundsson (2007), The Reykjanes Ridge between 63°10'N and Iceland, J. Geodynamics, 43, 73-86.
-
Howell, S.M., G. Ito, A.J. Breivik, A. Rai, R. Mjelde, B.Hanan, K. Sayit, P. Vogt (2014), The origin of the asymmetry in the Iceland hotspot along the Mid-Atlantic Ridge from continental breakup to present-day, Earth Planet. Sci. Lett., 392, 143–153.
-
Ito, G. (2001), Origin of V-Shaped Reykjanes Ridges from a Pulsing and Dehydrating Mantle Plume, Nature, 411, 681-684.
-
Jones, S.M. (2003), Test of a ridge-plume interaction model using oceanic crustal structure around Iceland, Earth Planet. Sci. Lett., 208, 205-218.
-
Jones, S.M., N. White, and J. Maclennan (2002), V-shaped ridges around Iceland: Implications for spatial and temporal patterns of mantle convection,Geochem. Geophys. Geosyst., doi:10.1029/2002GC000361.
-
Jones, S.M., B.J. Murton, J.G. Fitton, N.J. White, J. Maclennan and R.L. Walters (2014), A joint geochemical-geophysical record of time-dependent mantle convection south of Iceland, Earth Planet. Sci. Lett., 386, 86-97.
-
Keeton, J.A., R.C. Searle, B. Parsons, R.S. White, B.J. Murton, L.M. Parson, C. Peirce, M.C. Sinha (1997), Bathymetry of the Reykjanes Ridge, Mar. Geophys. Res., 19, 55-64.
-
Macdonald, K. C. (1982), Mid-ocean ridges: Fine scale tectonic, volcanic and hydrothermal processes within the plate boundary zone, Ann. Rev. Earth Planet. Sci., 10, 155-190.
-
Macdonald, K.C., R.M. Haymon, S. P. Miller, J.C. Sempere, and P.J. Fox (1988), Deep-Tow and Sea Beam studies of dueling propagating ridges on the East Pacific Rise near 20°40’S, J. Geophys. Res., 93, 2875-2898.
-
Macnab, R., J. Verhoef, W. Roest, and J. Arkani-Hamed (1995), New database documents the magnetic character of the Arctic and North Atlantic, Eos Trans. AGU, 76(45), 449 and 458.
-
Martinez, F., R.N. Hey and D.E. Eason (2015), Plate boundary processes as alternatives to mantle plume effects on the Reykjanes Ridge, Eos Trans. AGU, 96, Abstract V12A-03.
-
Merkuryev, S. A., C. DeMets, N. I. Gurevich (2009), Geodynamic evolution of crust accretion at the axis of the Reykjanes Ridge, Atlantic Ocean, Geotectonics, 43, 194–207.
-
Parnell-Turner, R., N. White, T. Henstock, B. Murton, J. Maclennan, and S.M. Jones (2014), A continuous 55-million-year record of transient mantle plume activity beneath Iceland, Nature Geoscience, 7, 914–919, doi: 10.1038/ngeo2281.
-
Poore, H.R., N. White, and S. Jones (2009), A Neogene chronology of Iceland plume activity from V-shaped ridges, Earth Planet. Sci. Lett., doi:10.1016/j.epsl.2009.02.028.
-
Sandwell, D. T., R. D. Müller, W. H. F. Smith, E. Garcia, and R. Francis (2014), New global marine gravity model from CryoSat-2 and Jason-1 reveals buried tectonic structure, Science, 346, 65-67.
-
Searle, R.C., J.A. Keeton, R.B. Owens, R.S. White, R. Mecklenburgh, B. Parsons, and S.M. Lee (1998), The Reykjanes Ridge; structure and tectonics of a hot-spot-influenced, slow-spreading ridge, from multibeam bathymetry, gravity and magnetic investigations, Earth Planet. Sci. Lett., 160, 463-478.
-
Smallwood, J. R., and R. S. White (2002), Ridge-plume interaction in the North Atlantic and its influence on continental breakup and seafloor spreading, in The North Atlantic Igneous Province: Stratigraphy, Tectonic, Volcanic and Magmatic Processes, edited by D. W. Jolley, B. R. Bell, Geological Society Special Publications, London, 197, 15-37.
-
Smith, W. H. F., and D. T. Sandwell (1997), Global seafloor topography from satellite altimetry and ship depth soundings, Science, 277, 1957-1962.
-
Vogt, P. R. (1971), Asthenosphere motion recorded by the ocean floor south of Iceland, Earth Planet. Sci. Lett., 13, 153-160.
-
Vogt, P.R. and O.E. Avery (1974), Detailed magnetic surveys in the northeast Atlantic and Labrador Sea, J. Geophys. Res., 79, 363-389.
-
Vogt, P.R. and G.L. Johnson (1975), Transform faults and longitudinal flow below the midoceanic ridge, J. Geophys. Res., 80, 1399-1428.
-
White, R. S. (1997), Rift-plume interaction in the North Atlantic. Phil. Trans. Roy. Soc. Lond, Series A, 355, 319-339.
-
White, N., and B. Lovell (1997), Measuring the pulse of a plume with the sedimentary record, Nature, 387, 888-891.
last updated 2nd August, 2016 |