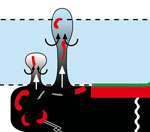 |
Melting of the oceanic crust in stagnant slabs in the transition zone: Constraints from alkaline basalts in eastern China |
Tetsuya Sakuyama1, Wei Tian2, Jun-Ichi Kimura1, Yoshio Fukao1, Yuka Hirahara1, Toshiro Takahashi1, Ryoko Senda1, Qing Chang1, Takashi Miyazaki1, Masayuki Obayashi1, Hiroshi Kawabata3, & Yoshiyuki Tatsumi4
1JAMSTEC, Japan; sakuyama@jamstec.go.jp ; jkimura@jamstec.go.jp ; fukao43@yahoo.co.jp ; hiraharay@jamstec.go.jp ; toshirot@jamstec.go.jp ; rsenda@jamstec.go.jp ; qchang@jamstec.go.jp ; tmiyazaki@jamstec.go.jp ; obayashi@jamstec.go.jp
2Peking University, China; davidtian@pku.edu.cn
3Kochi University, Japan
4Kobe University, Japan; tatsumi@diamond.kobe-u.ac.jp
Introduction
Stagnation of the subducted Pacific slab in the mantle transition zone beneath eastern China has been imaged by seismic tomography. Late Cenozoic (0–15 Ma) basalt volcanoes lying above the stagnant slab, are distributed throughout eastern China (Figure 1). These basalts are rich in alkaline and incompatible trace elements that are typically associated with oceanic island basalts (OIBs). Such geochemical similarities between the basalts in eastern Asia and typical OIBs have led some researchers to conclude that the Chinese basalts originated from the upwelling of mantle, independent of a stagnant slab. Others believe that they were produced by or related to chemical components derived from the stagnant slab. According to the results of high-pressure experiments, melting of the stagnant slab (which affects slab chemistry and density more than dehydration) could occur if the slab contains sufficient H2O or CO2. However, geochemical examination of the melting of the stagnant slab has not yet been conducted, owing to the difficulty in identifying the signature of deep (> 400 km) processes in surface rocks. In the present study we provide evidence that melts from the igneous layers in the stagnant Pacific plate slab contributed to the source composition of basalts erupted in eastern China.
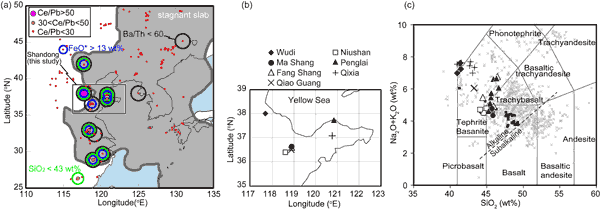
Figure 1: (a) Distribution of Cenozoic basalts with less differentiated compositions (SiO2 < 60 wt.% and FeO*/MgO < 1.3). Localities where samples with low SiO2 and high FeO* contents and a low Ba/Th (<60) were reported are encircled by thick light-green, blue, and black circles respectively. Localities where samples with extremely high Ce/Pb (N50) and intermediately high Ce/Pb are plotted by large pink, and orange circles respectively. The western edge of the 0.3–0.6% P-wave high-velocity anomaly at a depth of 550–630 km is shown as a grey region. Thin broken lines represent schematic tectonic boundaries (Zeng et al., 2011). NCC: North China craton; QDSL: Qinlin–Dabie– Su–Lu fold belt; SCC: South China craton. (b) Detailed localities of the samples from Shandong. Whole-rock: (c) Na2O + K2O content against MgO content, of Shandong basalts and Cenozoic basalts in eastern China (small grey crosses). A chemical classification indicated by grey lines and an alkaline/subalkaline boundary indicated by a broken line are from Le Bas et al. (1986) and Miyashiro (1978), respectively. Whole-rock major-element chemical compositions of Cenozoic basalts in eastern China were obtained from previous studies. Click here or on Figure for enlargement.
FeO-rich, SiO2-poor basalt in eastern China
Cenozoic basalts in the Shandong area, particularly Wudi and Qixia, are heavily enriched in Na2O + K2O (>6 wt%), FeO* (>13 wt.%), and TiO2 (>2 wt.%) and depleted in SiO2 (<43 wt.%) and Al2O3 (<13 wt.%) (Figure 2). Those basalts have distinctive trace-element patterns. Multi-element plots (normalized to primitive mantle) show that they commonly have convex-up patterns with depletions in Rb, Ba, Pb relative to other large-ion-lithophile elements and light rare-earth elements, and to a lesser extent, depletions in Zr and Hf relative to middle rare-earth elements. This is similar to those of the OIBs with HIMU isotopic signatures (Figure 3). However, in contrast to HIMU basalts, the radiogenic isotope compositions (Nd-Sr-Pb) of the Shandong basalts suggest that they were derived from a depleted mantle source rather than a HIMU mantle source (Figure 4).
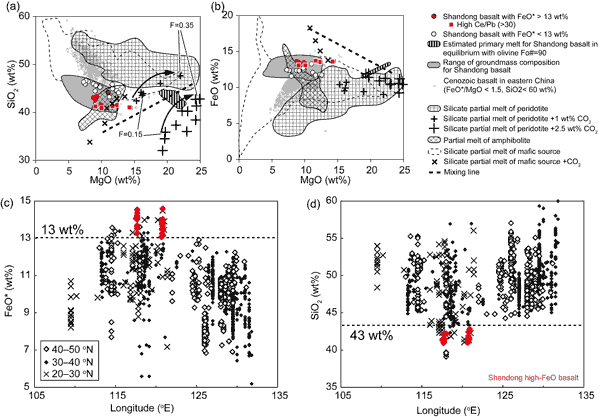
Figure 2: (a and b) Compositions of less differentiated (FeO*/MgO < 1.5, SiO2 < 60 wt.%) Cenozoic basaltic lavas in eastern China from this study, with compiled data shown as experimental partial melts of peridotite and mafic sources against the MgO content. A range of primary melts of all the Shandong basalts plotted was calculated by assuming the maximum olivine fractionation from the magma and compositionally dependent KD(Fe/Mg) ol/liq (Tamura et al., 2000). Fe3+/(Fe2++Fe3+) in the melt and Fo# of olivine in the source mantle peridotite were assumed to be 0.1 and 90, respectively. A mixture of the basalt and the equilibrium olivine was assumed to be in a weight ratio of 99.5:0.5. Chemical compositions of partial melts produced in melting experiments of peridotites were obtained from previous studies. (c and d) Major element compositions of less differentiated (FeO*/MgO < 1.5, SiO2 < 60 wt.%) Cenozoic basaltic lavas in eastern Eurasia obtained in this work, combined with compiled data, plotted against longitude. Click here or on Figure for enlargement.
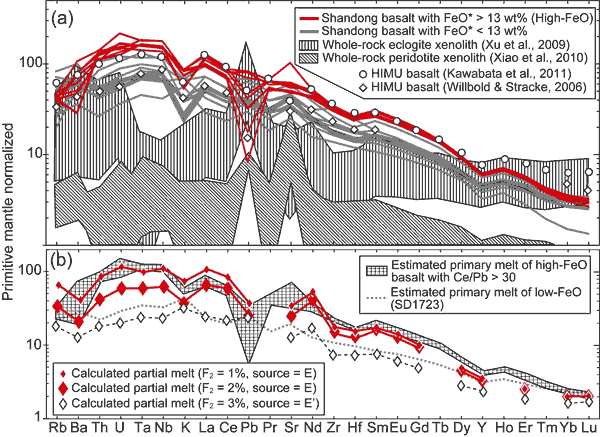
Figure 3: (a) Trace element compositions of volcanic rocks from Shandong, eclogite xenoliths (Xu et al., 2009), and peridotite xenoliths (Xiao et al., 2010) in eastern China, normalized to the primitive mantle (Sun & McDonough, 1989). The Shandong basaltic rocks exhibit characteristic depletions in Rb, Ba, Pb, Zr, and Hf relative to neighbouring elements. (b) Primitive mantle-normalized trace element compositions of an estimated primary melt compositions and modelled partial melts of the hybridized mantle.
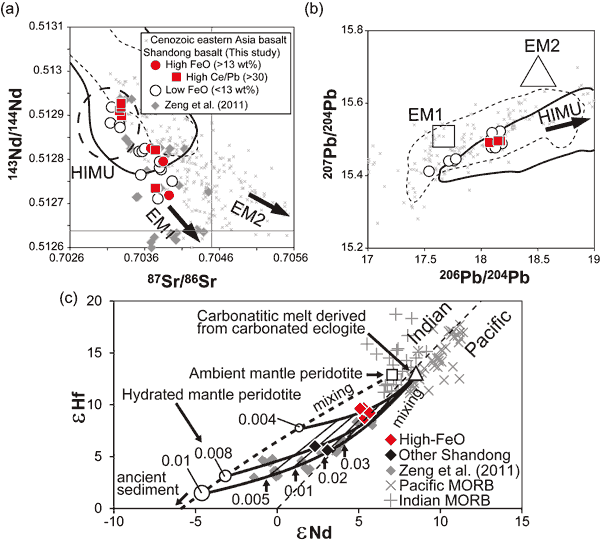
Figure 4: (a and b) Sr–Nd–Pb isotopic compositions for Shandong basalts and Cenozoic basalts in eastern China. (c) Variation in εNd vs. εHf for basalts in the Shandong area and Pacific MORB, showing the mixing line between the partial melt of the Pacific MORB and the assumed solid mantle, which has the least radiogenic isotopic composition.
The FeO-rich samples exhibit the highest 143Nd/144Nd and 176Hf/177Hf ratios, plotting at the end of the mixing curve closest to Pacific MORB, lying somewhere between Pacific MORB and Indian Ocean asthenospheric mantle affected by an ancient sediment-derived slab fluid (Figure 4). Melting of the stagnant Pacific slab igneous layer, which was modified by subduction zone processes but subjected to only short time-integrated ingrowth of Sr–Nd–Hf–Pb isotope systems, results in production of melt depleted in Rb, K, and Pb contents with isotopically depleted source mantle characteristics.
The extremely silica-undersaturated and iron-rich characteristics of the high-FeO basalts cannot be formed easily by the melting of any single source lithology, such as peridotite or eclogite. However, it can be reproduced by melting a hybridized source, presumably in the presence of CO2 (Figure 2). Our geochemical forward modelling calculations on trace elements also suggest that high-FeO* and high-Ce/Pb samples can be reproduced by melting of peridotite hybridized by fluid derived from subducted sediment and the partial melt of carbonated and dehydrated oceanic crust (Figure 3). Such FeO-rich (>13 wt.%), SiO2-poor (<43 wt.%) basalts occur only above the leading edge of the stagnant Pacific slab in eastern China, ~2000 km west of the Pacific Plate trench (Figure 2). The geochemical characteristics of the SiO2-poor basalts, particularly those of Shandong, lead us to conclude that the source of these basalts includes a strong contribution from melts generated at the leading edge of the stagnant slab of the Pacific plate. These melts are derived from the melting of dehydrated carbonate-bearing igneous layers at the leading edge of the stagnant slab (Figure 5).
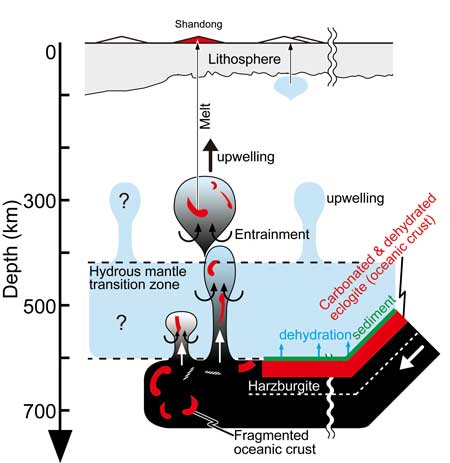
Figure 5: A schematic illustration of the upper mantle beneath Shandong.
This is the first time evidence has been presented that suggests that the carbonated igneous crustal layer has uplifted into the upper mantle by the buoyant harzburgite from the stagnant Pacific slab. As a result of conductive heating from the surrounding ambient upper mantle this carbonated igneous crustal layer melts to supply magma to generate Cenozoic basaltic volcanism in eastern China.
References
-
Le Bas, M. J., Le Maitre, R. W., Streckeisen, A. & Zanettin, B. (1986). A chemical classification of volcanic rocks based on the total alkali-silica diagram. Journal of Petrology 27, 745-750.
-
Miyashiro, A. (1978). Nature of alkalic volcanic rock series. Contributions to Mineralogy and Petrology 66, 91-104.
-
Sun, S.-S. & McDonough, W. F. (1989). Chemical and isotopic systematics of oceanic basalts: implications for mantle composition and processes. Magmatism in the Ocean Basins, Geological Society Special Publication 42, 313-345.
-
Tamura, Y., Yuhara, M. & Ishii, T. (2000). Primary arc basalts from Daisen volcano, Japan: Equilibrium crystal fractionation versus disequilibrium fractionation during supercooling. Journal of Petrology 41, 431-448.
-
Xiao, Y., Zhang, H. F., Fan, W. M., Ying, J. F., Zhang, J., Zhao, X. M. & Su, B. X. (2010). Evolution of lithospheric mantle beneath the Tan-Lu fault zone, eastern North China Craton: Evidence from petrology and geochemistry of peridotite xenoliths. Lithos 117, 229-246.
-
Xu, W. L., Yang, D. B., Pei, F. P. & Yu, Y. (2009). Petrogenesis of Fushan high-Mg(#) diorites from the southern Taihang Mts. in the central North China Craton: Resulting from interaction of peridotite-melt derived from partial melting of delaminated lower continental crust. Acta Petrologica Sinica 25, 1947-1961.
-
Zeng, G., Chen, L.-H., Hofmann, A. W., Jiang, S.-Y. & Xu, X.-S. (2011). Crust recycling in the sources of two parallel volcanic chains in Shandong, north China. Earth and Planetary Science Letters 302, 359-368.
last updated 27th March, 2014 |