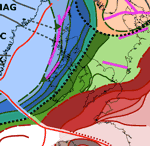 |
Large-scale Structural Inheritance in the Circum-North Atlantic Region |
Christian Schiffer1, Anthony G. Doré2, Gillian R. Foulger3, Dieter Franke4, Laurent Geoffroy5, Laurent Gernigon6, Robert E. Holdsworth3, Nick Kusznir7, Erik Lundin8, Ken J.W. McCaffrey3, Alexander L. Peace9, Kenni Petersen10, Thomas Phillips3, Randell Stephenson11, Martyn Stoker12, J. Kim Welford13
1Department of Earth Sciences, Uppsala University, Villavägen 16, 75236 Uppsala, Sweden; christian.schiffer@geo.uu.se
2Energy & Geoscience Institute (EGI, University of Utah), office London; agdore@gmail.com
3Department of Earth Sciences, Durham University, Science Laboratories, South Rd. DH1 3LE, UK; g.r.foulger@durham.ac.uk ; R.E.Holdsworth@durham.ac.uk ; k.j.w.mccaffrey@durham.ac.uk ; thomas.b.phillips@durham.ac.uk
4Bundesanstalt für Geowissenschaften und Rohstoffe (Federal Institute for Geosciences and Natural Resources), Germany; Dieter.Franke@bgr.de
5Université de Bretagne Occidentale, Brest, 29238 Brest, CNRS, UMR 6538, Laboratoire Domaines Océaniques, 29280 Plouzané, France; Laurent.Geoffroy@univ-brest.fr
6Norges Geologiske Undersøkelse (NGU), Geological Survey of Norway, Leiv Erikssons vei 39, N-7491 Trondheim, Norway; Laurent.Gernigon@ngu.no
7University of Liverpool, School of Environmental Sciences, Liverpool L69 3GP, United Kingdom; n.kusznir@liverpool.ac.uk
8Equinor, Research Centre, Arkitekt Ebbels vei 10, 7053 Trondheim, Norway; erlun@equinor.com
9School of Geography and Earth Sciences, McMaster University, Hamilton, Ontario, Canada, L8S 4L8; peacea2@mcmaster.ca
10Department of Geoscience, Aarhus University, Høegh-Guldbergs Gade 2, DK-8000 Aarhus C, Denmark; kenni.dinesen.petersen@gmail.com
11School of Geosciences, University of Aberdeen, King’s College, Aberdeen AB24 3UE, UK; r.stephenson@abdn.ac.uk
12Australian School of Petroleum, University of Adelaide, Adelaide, SA 5005, Australia; martyn.stoker@gmail.com
13Department of Earth Sciences, Memorial University of Newfoundland, St. Johns, Newfoundland, Canada, A1B 3X5; kwelford@mun.ca
This webpage is a summary of Schiffer, C., A. G. Doré, G. R. Foulger, D. Franke, L. Geoffroy, L. Gernigon, B. Holdsworth, N. Kusznir, E. Lundin, K. McCaffrey, A. L. Peace, K. D. Petersen, T. B. Phillips, R. Stephenson, M. S. Stoker, and J. K. Welford (2019), Structural inheritance in the North Atlantic, Earth-Science Reviews, 102975.
Introduction
The Circum-North Atlantic region (CNAR) comprising the North Atlantic Ocean and its surrounding continents is the type example of the Wilson-cycle concept and regarded as a classic case of structural inheritance (Wilson 1966; see review by Wilson et al., 2019). The lithosphere of the CNAR is a patchwork of stable Precambrian continental cores, orogenic belts, continental rifts and new ocean basins (Figures 1,2). Breakup of the NE Atlantic follows Caledonian and Variscan orogenic trends in general but in detail was a protracted, diachronous and complex process that did not follow known sutures zones exactly (Figures 1-3) (Ady & Whittaker, 2018; Gernigon et al., 2019; Peace et al., 2019).
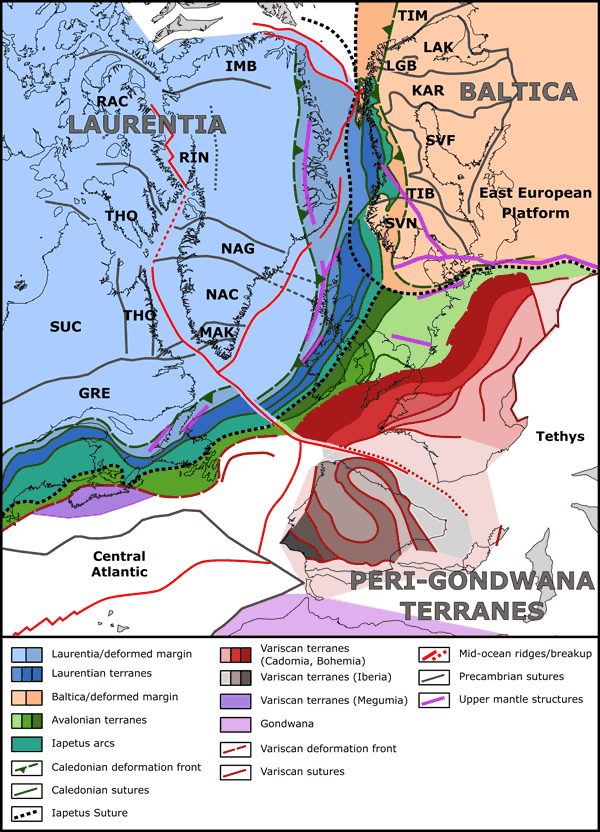
Figure 1: Terrane and structure map of the Circum-North Atlantic region at 145 Ma showing continents, terranes, suture zones and upper mantle structures. Caledonian and Variscan terranes and structures are generally closely aligned with the breakup axes In the NE Atlantic, breakup occurred west of the Iapetus Suture; In contrast, breakup occurred to the east of the Iapetus Suture in the northern Central Atlantic. Precambrian terranes are generally perpendicularly aligned, but have therefore probably affected margin segmentation and the formation of transform zones and faults during rifting. The NW Atlantic is a region where an ocean opened (Labrador Sea and Baffin Bay) not following any known Phanerozoic structures or terranes and cross-cutting Precambrian lineaments. However, the Rinkian Orogen may have played a role during the formation of Baffin Bay. GRE – Grenvillian Orogen, IMB – Inglefield Mobile Belt, KAR – Karelian, LGB – Lapland Granulite Belt, MAK – Makkovik-Ketilidian Orogen, NAC – North Atlantic Craton, NAG – Nagssugtoqidian Orogen, RAC – Rae Craton, RIN – Rinkian Orogen, SUC – Superior Craton, SVF – Svecofennian, SVN – Sveconorwegian Orogen, THO – Trans-Hudson Orogen, TIB – Transscandinavian Igenous Belt, TIM – Timanian Orogen. Click here or on Figure for enlargement.
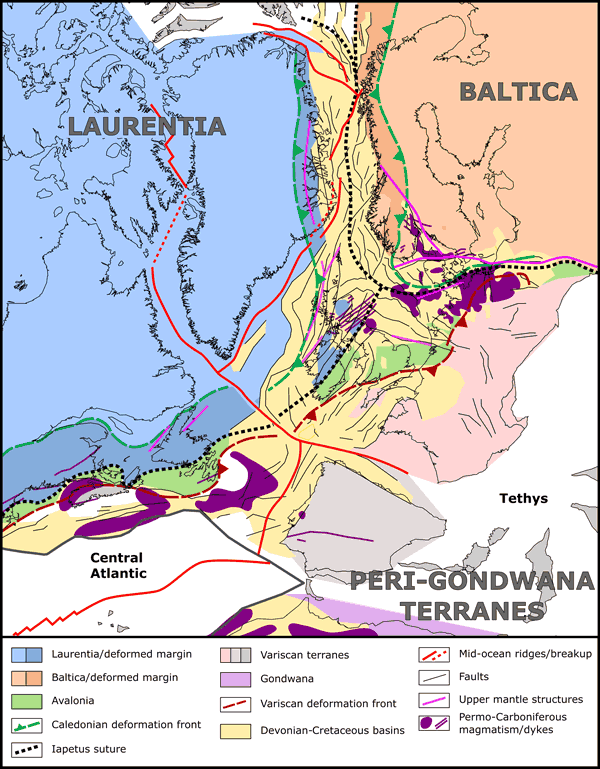
Figure 2: Basin and structure map of the Circum-North Atlantic region at 145 Ma showing continents, basins, faults, upper mantle structures and Permo-Carboniferous magmatism. Almost all of the early, Devonian-Jurassic basins illustrated in this figure have formed within the Caledonian or Variscan orogens (within their deformation fronts) and oblique to the axis of breakup. Click here or on Figure for enlargement.
Schiffer et al. (2019) examine, summarize and evaluate different aspects of lithospheric-scale structural inheritance and rejuvenation, as well as the diachronous and segmented rifting and breakup history in the CNAR. We describe and discuss the CNAR in five segments of different structural style: The Norwegian-Greenland Sea (Segment 1), the SE Greenland-Rockall-Hatton margins (Segment 2), the enigmatic Greenland-Iceland-Faroe Ridge (GIFR) that separates Segment 1 from Segment 2 (Segment 3), the North Sea and related seas (Segment 4) and the oceanic Labrador Sea and Baffin Bay (Segment 5).
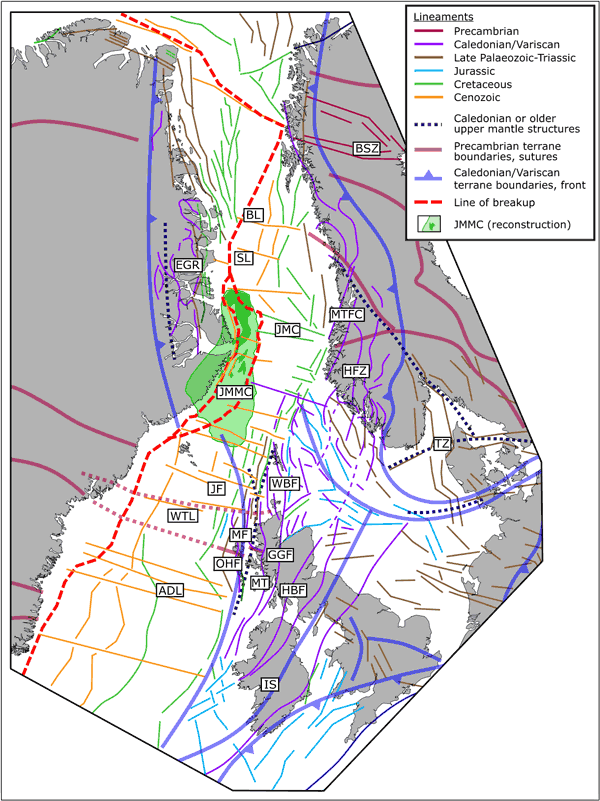
Figure 3: Basement terranes and lineaments of the NE Atlantic margins in a plate reconstruction at 60 Ma. Lineaments are coloured according to their main observed age of expression. Abbreviations: ADL, Anton Dohrn Lineament; BL, Bivrost Lineament; BSZ, Bothnian-Senja Shear Zone; EGR, East Greenland Rift System; GGF, Great Glen Fault; HBF, Highland Boundary Fault; HFZ, Hardangerfjord Fault Zone; IS, Iapetus Suture; JF, Judd Fault; JMMC, Jan Mayen Microplate Complex; JMC, Jan Mayen Corridor; MF, Mich Fault Zone; MTFZ, Møre-Trøndelag Fault Zone; MT, Moine Thrust; OHF, Outer Hebrides Fault Zone; SL, Surt Lineament; TZ, Tornquist Zone; WTL, Wyville-Thomson Lineament. Click here or on Figure for enlargement.
Rifting, segmentation and breakup
Schiffer et al. (2019) suggest that in the NE Atlantic (Segments 1-4), many of the late Palaeozoic to Cretaceous rift systems followed the trend of roughly NE-SW-oriented Caledonian structures (Figure 3). These were predominantly sub-vertical, orogen-parallel strike-slip faults formed during the Silurian-Devonian such as the Great Glen Fault and Møre-Trøndelag Fault Complex. Along-margin segmentation is primarily related to pre-Caledonian lineaments and transfer zones.
A key observation in the northernmost NE Atlantic (Segment 1) is the obliquity of breakup axis compared to the trends of earlier rift structures (Figure 4). A reason for this obliquity could lie in the existence of lithospheric layers with differently oriented pre-existing fabrics. The shallow fabric was formed during Caledonian thrusting, whilst the deeper upper mantle fabric may have been formed during late-Caledonian shear- and strike-slip deformation. This may also explain the similar orientation of breakup axis and late-Caledonian shear zones exposed at the surface.
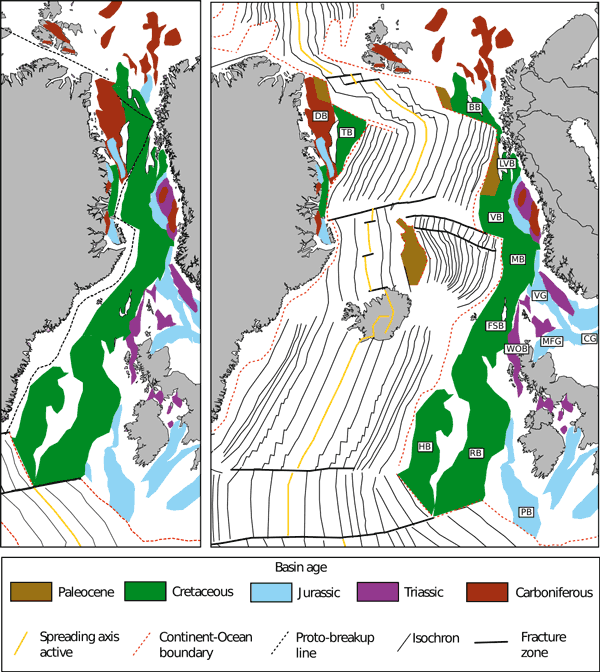
Figure 4: Basin age map of the North Atlantic, shown before breakup at 53 Ma (left), and at the present day (right). Basins are coloured according to the age of the crustal extension that mainly created the basin. The general asymmetry of the breakup (much of pre-existing basin system was left on the European margin) and the obliquity of the breakup in the NE are clearly shown. Abbreviations: BB, Bjørnøya Basin; CG, Central Graben; DB, Danmarkshavn Basin; FSB, Faroe-Shetland Basin; HB, Hatton Basin; LVB, Lofoten-Vestrålen Basin; MB, Møre Basin; MFG, Moray Firth Graben; PB, Porcupine Basin; RB, Rockall Basin; TB, Thetis Basin; VB, Vøring Basin; VG, Viking Graben; WB, Wandel Sea Basin, WOB, West Orkney Basin. Click here or on Figure for enlargement.
These depth-dependent fabrics rejuvenated at different times in response to changes in the regional stress field, such as occurred in the Eocene. Research shows that deeper lithospheric fabrics are more efficiently rejuvenated compared to shallower fabrics in the upper crust (e.g., Heron et al., 2016). Thus we argue that breakup was achieved first after the direction of extension was favourably aligned with the deep late-Caledonian shear fabric rather than the shallow, oblique Caledonian thrust faults.
The occurrence of pre- and syn-rift magmatism is also generally distributed parallel to the line of breakup, which may suggest that magmatic intrusions additionally “perforated” and weakened the lithosphere (Gernigon et al., 2019). Pre-rift strike-slip deformation has been also suggested as a mechanism to perforate the lithosphere and facilitate breakup (Lundin & Doré, 2018).
In the southern NE Atlantic (Segment 2) breakup occurred generally parallel to Caledonian structures, but 500 km east of the Caledonian suture through undisturbed cratonic lithosphere, leaving large parts of Laurentian lithosphere on the British and Irish margins. The line of breakup obviously relocated from previously developed and subsequently abandoned major rifts on the Rockall-Hatton shelf. One model for such rift relocation suggests cooling and strengthening of the lithosphere after cessation of the initial rift (Van Wijk & Cloetingh, 2002).
The GIFR is an enigmatic physiographic high underlain by thick crust and forms a buffer between Segments 1 and 2, crossing the North Atlantic. It may contain a significant component of continental lithosphere (Foulger et al., 2019) and may owe its existence to diffuse, oblique extension across a complex zone of interacting Precambrian and Caledonian inherited structures. The GIFR may be a key element to explain many observations and peculiarities in the NE Atlantic:
- Its possibly rheologically different lithosphere could be the reason the GIFR developed as a large-scale transfer zone between Segments 1 and 2;
- Flow of warm and weak crustal material may have facilitated retention of the thick continental crust now observed beneath the GIFR;
- After major rotation of plate motion/stress field in the Eocene, the GIFR was under local transpression and locked, thereby causing the Jan Mayen Microplate to release from East Greenland (Schiffer et al., 2018).
The North Sea (Segment 4) is a major intracontinental rift system formed with complex interaction of rejuvenation of Variscan, Caledonian and Precambrian structures. It never developed into a new ocean.
In the Labrador Sea and Baffin Bay (Segment 5), rifting and breakup seem to have broken perpendicular to known cratonic and orogenic trends, with the exception perhaps of the Rinkian fold belt in NW Greenland which is oriented parallel to the Baffin Bay margin. Breakup of the Labrador Sea may have been guided along the western edge of the North Atlantic craton, while the northern craton boundary may have controlled the location of the Davis Strait transform (Heron et al., 2019). Several sets of Precambrian dyke swarms are sub-parallel to rift and breakup trends (Buchan & Ernst, 2006).
The Wilson Cycle in the North Atlantic
The Wilson Cycle predicts closure and reopening of oceans along orogenic belts that represent weak zones. It is one of the important concepts describing the role of first-order inheritance in plate tectonic theory. However, the original Wilson Cycle theory fails to explain the many structural complexities of developing oceans and continental margins, and how and why the North Atlantic opened in the first place.
During the past 50 years, more data have been acquired, and new theories proposed, but the mechanisms driving the Wilson Cycle and plate tectonics are still a matter of debate. Aspects such as long-lasting intraplate inheritance (Heron et al., 2016), mantle dynamics (Heron, 2018) and “short-cuts” through the Wilson Cycles (such as incomplete closure of oceans or incomplete rift phases) (Chenin et al., 2018) have been formally incorporated into the Wilson Cycle model. It is also becoming increasingly clear that the age of structural inheritance extends much further back in time than simply the most recent phase of oceanic closure and orogenesis (Peace et al., 2018; Schiffer et al., 2018; Heron et al., 2019).
Concluding remarks
The CNAR is the type example of the Wilson Cycle concept and, in general, reopened elements of the Caledonian and Appalachian fold belt. In the CNAR, however, Pangaea did not simply re-open along the surface traces of suture zones. The rift evolution and opening of the North Atlantic ocean is to varying degrees linked to rejuvenation of differently oriented anisotropies at different depths (crust vs. mantle) originating from different stages of the orogenic evolution (collision vs. late Caledonian transpression-transtension). Our model assumes highly three-dimensional geometries of differently aged orogenic and extensional fabrics and that the weakest structures are likely at depth and not at the surface. Additional factors such as regional stress-field changes, plate-boundary effects, magmatism and strike-slip deformation may explain the observed complexities. Inheritance at all scales and preserved over billions of years is important in explaining rejuvenation at regional and global scales.
References
-
Ady, B.E., and Whittaker, R.C., 2018, Examining the influence of tectonic inheritance on the evolution of the North Atlantic using a palinspastic deformable plate reconstruction: Geological Society, London, Special Publications, 470, SP470–9.
-
Buchan, K.L., and Ernst, R., 2006, Giant dyke swarms and the reconstruction of the Canadian Arctic islands, Greenland, Svalbard and Franz Josef Land, in Dyke Swarms - Time Markers of Crustal Evolution, Taylor & Francis, 27–48, http://www.crcnetbase.com/doi/abs/10.1201/NOE0415398992.ch2 (accessed June 2014).
-
Chenin, P., Picazo, S., Jammes, S., Manatschal, G., Müntener, O., and Karner, G., 2018, Potential role of lithospheric mantle composition in the Wilson cycle: a North Atlantic perspective: Geological Society, London, Special Publications, 470, SP470–10.
-
Foulger, G.R. et al., 2019, The Iceland Microcontinent and a continental Greenland-Iceland-Faroe Ridge: Earth-Science Reviews, 102926, doi:10.1016/j.earscirev.2019.102926.
-
Gernigon, L., Franke, D., Geoffroy, L., Schiffer, C., Foulger, G.R., and Stoker, M., 2019, Crustal fragmentation, magmatism, and the diachronous opening of the Norwegian-Greenland Sea: Earth-Science Reviews, doi:10.1016/j.earscirev.2019.04.011.
-
Heron, P.J., 2018, Mantle plumes and mantle dynamics in the Wilson cycle: Geological Society, London, Special Publications, 470, SP470.18, doi:10.1144/SP470.18.
-
Heron, P. J., A. L. Peace, K. J. W. McCaffrey, J. K. Welford, R. Wilson, J. van Hunen, and R. N. Pysklywec (2019), Segmentation of Rifts Through Structural Inheritance: Creation of the Davis Strait, Tectonics, 38, 2411-2430.
-
Heron, P.J., Pysklywec, R.N., and Stephenson, R., 2016, Lasting mantle scars lead to perennial plate tectonics: Nature Communications, 7, 11834, doi:10.1038/ncomms11834.
-
Peace, A.L., Dempsey, E., Schiffer, C., Welford, J., McCaffrey, K., Imber, J., and Phethean, J., 2018, Evidence for Basement Reactivation during the Opening of the Labrador Sea from the Makkovik Province, Labrador, Canada: Insights from Field Data and Numerical Models: Geosciences, 8, 308.
-
Peace, A.L., Phethean, J.J.J., Franke, D., Foulger, G.R., Schiffer, C., Welford, J.K., McHone, G., Rocchi, S., Schnabel, M., and Doré, A.G., 2019, A review of Pangaea dispersal and Large Igneous Provinces – In search of a causative mechanism: Earth-Science Reviews, 102902, doi:10.1016/j.earscirev.2019.102902.
-
Schiffer, C., A. G. Doré, G. R. Foulger, D. Franke, L. Geoffroy, L. Gernigon, B. Holdsworth, N. Kusznir, E. Lundin, K. McCaffrey, A. L. Peace, K. D. Petersen, T. B. Phillips, R. Stephenson, M. S. Stoker, and J. K. Welford (2019), Structural inheritance in the North Atlantic, Earth-Science Reviews, 102975.
-
Schiffer, C., Peace, A., Phethean, J., Gernigon, L., McCaffrey, K., Petersen, K.D., and Foulger, G.R., 2018, The Jan Mayen Microplate Complex and the Wilson Cycle: Geological Society Special Publication, 470.
-
Van Wijk, J.W., and Cloetingh, S.A., 2002, Basin migration caused by slow lithospheric extension.: Earth and Planetary Science Letters, 198, 275–288.
-
Wilson, J.T., 1966, Did the Atlantic Close and then Re-Open? Nature, 211, 676–681, doi:10.1038/211676a0.
-
Wilson, R.W., Houseman, G.A., Buiter, S.J.H., McCaffrey, K.J.W., and Doré, A.G., 2019, Fifty years of the Wilson Cycle Concept in Plate Tectonics: An Overview: Geological Society, London, Special Publications, 470, SP470-2019–58, doi:10.1144/SP470-2019-58.
last updated 10th
January, 2020 |