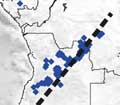 |
South Atlantic opening: A plume-induced breakup? |
Tanja Fromm1, Lars Planert2,†, Wilfried Jokat1, Trond Ryberg3, Jan H. Behrmann2, Christian Haberland3 & Michael Weber3,4
1Alfred-Wegener-Institut für Polar- und Meeresforschung, Am Alten Hafen 26, D-27568 Bremerhaven, Germany, Tanja.Fromm@awi.de ; Wilfried.Jokat@awi.de
2GEOMAR, Helmholtz Centre for Ocean Research Kiel, Wischhofstrasse 1-3, D-24148 Kiel, Germany, lplanert@geomar.de ; jbehrmann@geomar.de
3Helmholtz-Centre Potsdam - German Research Centre for Geosciences (GFZ), Telegrafenberg, D-14473 Potsdam, Germany, trond@gfz-potsdam.de ; christian.haberland@gfz-potsdam.de ; michael.weber@gfz-potsdam.de
4University of Potsdam, Institute of Earth and Environmental Science, Golm, D-14471 Potsdam, Germany.
†Current address: Bundeswehr Technical Center WTD71, Research Department for Underwater Acoustics and Marine Geophysics, Berliner Strasse 115, D-24340 Eckernförde, Germany
This webpage is a summary of: Fromm, T., L. Planert, W. Jokat, T. Ryberg, J. H. Behrmann, M.H. Weber, and Christian Haberland, South Atlantic opening: A plume-induced breakup?, Geology, 43, 931-934, 2015.
Introduction
Deep mantle plumes are thought to play an important role in plate tectonics. In the original plume model Morgan (1971) postulated that regional uplift and stress induced by thermal doming above an arriving plume head cracked the continents and pushed them apart. More recent simulations show that plumes also have the potential to thermally and chemically erode the base of the lithosphere (Sobolev et al., 2011) and promote the accumulation of melt that weakens the lithosphere. This melt intrudes the crust and erupts at the surface as large flood basalt provinces.
The formation of flood basalt provinces is often in close spatial and temporal proximity to continental breakup, which has led to the controversial concept that the impact of plume heads arriving at the base of the lithosphere initiates continental breakup (e.g., Cande & Stegman, 2011). However, this model is only one possible end member and observations from continental margins with and without flood basalt provinces suggest a very different explanation: preexisting weak zones and a prior history of rifting in combination with plate movements might be more important factors for breakup (e.g., Armitage et al., 2010; Buiter & Torsvik, 2014).
When hot plume material intrudes the crust it alters the normal crustal structure and leads to increased seismic velocities. Seismic imaging therefore offers valuable clues about the distribution of anomalous mantle material. In this webpage we explain how we interpret new deep seismic data from the junction of the Walvis Ridge with the Namibian continental margin. The results provide new insights into plume-crust interaction in the presence of weak zones during continental breakup and the initiation of South Atlantic opening.
New seismic data
We use seismic refraction data to image the crustal structure associated with the Tristan hotspot track and the proposed site of the plume head impact (Duncan, 1984): the easternmost Walvis Ridge in the South Atlantic Ocean and its junction with the Namibian coast (Figure 1). The area is well covered by four, mostly amphibious, deep seismic sounding profiles. The data image the crust along four profiles varying in length from 470 to 730 km. The travel times of refracted and reflected P-phases were used to derive two-dimensional velocity models using standard modeling procedures: forward modeling with program rayinvr (Zelt, 1992) and tomography with tomo2D (Korenaga et al. 2000). Further details of the analysis and data examples are available in the supplement of the original paper.
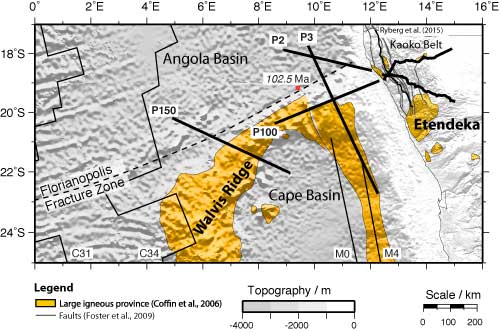
Figure 1: Location of the deep crustal seismic profiles at Walvis Ridge in the southeast Atlantic Ocean. Magnetic anomalies are shown with their ages (Gee & Kent, 2007): C34 - 83.5 Ma, M0 -120.6 Ma, M4 - 125.7 Ma. Red square marks the dated Ocean Drilling Program (ODP) Site 530 Leg 75 (Hay & Sibuet, 1984).
Results & Discussion
Our P-wave velocity models (Figure 2) show that the edifice of the Walvis Ridge consists of closely spaced seamounts and up to 35-km-thick crust. Crustal velocities of 5.5-7.2 km/s are typical of normal gabbroic oceanic crust. The crustal fabric of the Walvis Ridge is therefore of gabbroic composition similar to normal oceanic crust but approximately 5 times thicker. The transition from the Walvis Ridge to the adjacent basins reveals drastic differences between the northern and the southern flanks as well as along the axis of the ridge. While the southern flank gradually converts into the continent ocean transition of the volcanic margin (Figure 2b), the northern flank is characterized by a surprisingly sharp transition from 35-km-thick crust below the ridge to 5–6-km-thick normal oceanic crust in the Angola Basin (Figures 2b and 2c).
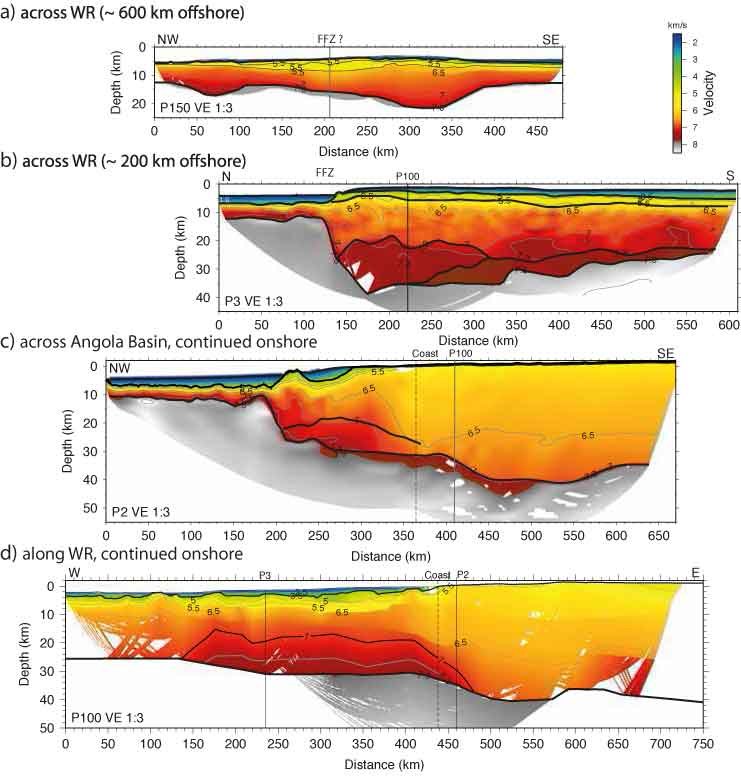
Figure 2: P-wave velocity models. A: P150 across Walvis Ridge (WR) 600 km offshore. B: P3 across WR 200 km offshore. C: P2 across Angola Basin and WR with an angle of 45°. D: P100 along axis. All models are plotted with the same scaling and a vertical exaggeration of 3. Reflectors are marked with thick black lines. White model areas have no ray coverage and are not resolved. The 7.3 km/s contour line is emphasized in P100.
This surprising jump in crustal thickness on the Angola Basin side can be explained by the kinematic evolution of the South Atlantic (Figure 3). The Angola Basin is considerably younger than the Cape Basin (up to 20 Ma; Gee & Kent, 2007; Hay & Sibuet, 1984) and the northern flank of Walvis Ridge is defined by the Florianopolis Fracture Zone (FFZ). The crust that formed initially to the north of Walvis Ridge has likely been sheared along the FFZ and transferred to the South American margin as the Sao Paolo Plateau (Figure 3b). The younger/thinner crust found today in the Angola Basin was formed after the magmatic activity associated with the hotspot was located further westward (Figure 3c). This implies that the plume tail did not supply sufficient additional melt to thicken the oceanic crust 200–600 km away in the Angola Basin.
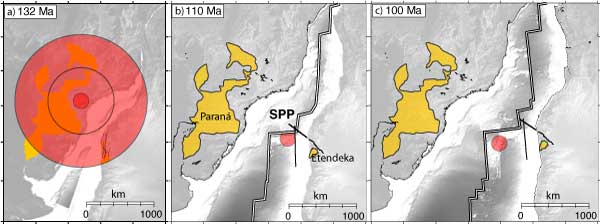
Figure 3: Reconstruction of South Atlantic opening (Pérez-Díaz & Eagles, 2014). Large red circles in a) mark the postulated location 1000 km and 2000 km contours of the plume head (O’Connor & Duncan, 1990). Small red circles denote the location of the plume stem with a diameter of 200 km. Black lines indicate the reconstructed positions of profiles 2 and 3. Thin black lines in a) show faults (Foster et al., 2009). Double line marks the spreading center. Yellow areas indicate continental flood basalts (Coffin et al., 2006).
In the west-east direction along the axis of Walvis Ridge crustal thickness increases from 18 to 30 km towards the coast (Figure 2d). The continental crust reaches 40 km thickness beneath the Kaoko Fold Belt. Close to the coast, the models show high seismic velocities (up to 7.5 km/s) in the lower crust of the Walvis Ridge indicating fractions of ultramafic mantle intrusions. This high-velocity lower crustal body (HVLCB) is partly constrained by reflections from the top and otherwise defined by the 7.0 km/s contour line. Such a HVLCB has been observed along the whole continental margin south of the Walvis Ridge and is considered to be part of the volcanic margin.
The offshore extent of the HVLCB beneath Walvis Ridge is similar to the remaining portions of the volcanic margin (Figure 2; Bauer et al., 2000; Hirsch et al., 2009; Schinkel, 2006). Quite different is the landward-facing boundary. There, the HVLCB continues 100 km further inland (Figure 1). Independent onshore seismic profiles confirm our findings and furthermore indicate that this eastern promontory of the Walvis HVLCB is only 100 km wide (Ryberg et. al., 2015). Thus, compared to the southern volcanic margin, the additional area of intrusive lower crust at the landfall of Walvis Ridge is at most 100 × 100 = 10,000 km2 (Figure 4, inset). According to our data, the continental crust including the root of the Kaoko Fold Belt has not been significantly modified by the proposed plume head.
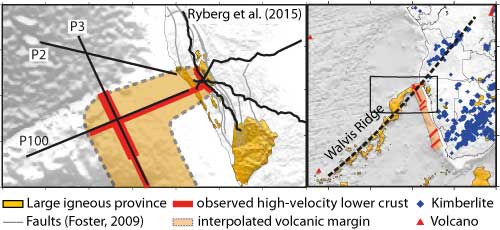
Figure 4: Track of the Tristan-Gough hotspot extended on the African continent. The dashed line follows the axis of Walvis Ridge and coincides with kimberlite intrusions onshore. Together with the narrow track-like promontory of the high-velocity lower crustal body (HVLCB) in prolongation of the Walvis Ridge it indicates that both volcanic features might be related. The inset magnifies the distribution of HVLC observed in the models presented and demonstrates their relation to onshore faults and flood basalts.
The intruded area around Walvis Ridge is surprisingly small in comparison to the often-cited diameters of plume heads, which is between 800 and 2000 km based on the regional extent of flood basalt volcanism (White & McKenzie, 1989) and theoretical calculations (Tan et al., 2011). The limited encroachment into African continental crust may be explained by greater distance from the center, but then it is reasonable to expect a much wider shape than the observed 100 km resembling a large diameter circle (Figure 3a). Instead, the confinement of intruded continental crust to a narrow strip in the landward prolongation of the Walvis Ridge seamount chain suggests a hotspot track origin and is not the signature of a plume head.
There are additional indications for the existence of a hotspot long before breakup. An indication for such a pre-existing hotspot is the geometry of the continental HVLCB and its relation to continental fault systems. In Namibia, the northern Etendeka basalts are associated with deep-reaching coast-parallel faults (Foster et al., 2009), which extend well beyond the area of basalt outcrops and intruded lower crust. In the plume-head scenario it is difficult to explain why only this localized crustal portion was affected even though the faults are much longer and would have been completely underlain by the plume head (Figure 3a).
The presence of a well-established hotspot producing mantle melts prior to continental breakup implies that the Tristan hotspot track might extend onto the African continent. Further volcanic features onshore include a lineament of kimberlites, scattered along an eastward-extrapolation of the ridge axis (Figure 4). Such rocks have long been associated with hotspots underneath thick continental lithosphere and indicate the presence of a thermal anomaly beneath the craton (e.g. Crough et al., 1980). If these features are associated with the Tristan hotspot, the onset of the Walvis Ridge cannot mark the beginning of the Tristan hotspot chain (Figure 5).
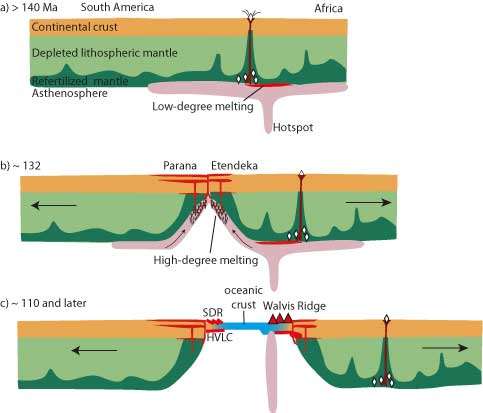
Figure 5: Sketch of the proposed breakup model. a) The hotspot existed prior to the rifting and formed low-degree melts at the hotspot location. Lithospheric structure focused intrusions venting to the surface and marking the hotspot trail by kimberlites. b) Changing plate boundary forces (Jokat et al., 2003) stretched the lithosphere and initiated rifting. Decompression melting at the thinned areas generated large volumes of melt which formed the large flood-basalt provinces. The following onset of seafloor spreading was characterized by excessive melt extraction building the volcanic margins. c) Further plate movement over the hotspot formed the Walvis Ridge.
This model implies that the source for the large volumes of melt required for the flood basalt volcanism was ponded hotspot material at the base of the lithosphere as previously suggested (Sleep, 2006). With the onset of rifting in response to changing plate boundary forces driven by spreading systems in the young ocean basins around Antarctica (Jokat et al., 2003) new melt pathways became available for the ponded melt to migrate to the surface and form the flood-basalt provinces and volcanic margins.
Conclusion
We do not find evidence for large-scale intrusions within the continental crust at the junction with the Walvis Ridge which would indicate plume head-lithosphere interaction during South Atlantic breakup. Thus, it seems unlikely that the arrival of a plume head initiated the opening of the South Atlantic Ocean.
A second, companion paper discusses our onshore geophysical investigations of the landfall region of Walvis Ridge: Ryberg, T., Haberland, C., Haberlau, T., Weber, M. H., Bauer, K., Behrmann, J. H., and Jokat, W., 2015, Crustal structure of northwest Namibia: Evidence for plume-rift-continent interaction, Geology, 43(8), 739-742, 10.1130/G36768.1
Acknowledgments
We thank the German Research Foundation for funding this project (BE 1041/29-1, JO-191/15-1), the South Atlantic Margin Processes and Links with onshore Evolution (Sample SPP), the crew of the R/V Maria S. Merian and the onshore field party. Seismic instruments onshore were provided by the Geophysical Instrument Pool (GIPP, GFZ Potsdam) and offshore by Geomar, Kiel.
References
-
Armitage, J.J., Collier, J.S., and Minshull, T.A., 2010, The importance of rift history for volcanic margin formation: Nature, 465, p. 913–917, doi:10.1038/nature09063.
-
Bauer, K., Neben, S., Schreckenberger, B., Emmermann, R., Hinz, K., Fechner, N., Gohl, K., Schulze, A., Trumbull, R., and Weber, K., 2000, Deep structure of the Namibia continental margin as derived from integrated geophysical studies: Journal of Geophysical Research, 105, B11, doi:10.1029/2000JB900227.
-
Buiter, S.J., and Torsvik, T.H., 2014, A review of Wilson Cycle plate margins: A role for mantle plumes in continental break-up along sutures?: Gondwana Research, 26, doi:10.1016/j.gr.2014.02.007.
-
Cande, S.C., and Stegman, D.R., 2011, Indian and African plate motions driven by the push force of the Reunion plume head: Nature, 475, p. 47–52, doi:10.1038/nature10174.
-
Coffin, M.F., Duncan, R.A., Eldholm, O., Fitton, J.G., Frey, F.A., Larsen, H.C., Mahoney, J.J., Saunders, A.D., Schlich, R., and Wallace, P.J., 2006, Large igneous provinces and scientific ocean drilling: Status quo and a look ahead: Oceanography (Washington, D.C.), 19, p. 150–160, doi:10.5670/oceanog.2006.13.
-
Crough, S.T., Morgan, W.J., and Hargraves, R.B., 1980, Kimberlites: their relation to mantle hotspots: Earth and Planetary Science Letters, 50, p. 260–274, doi:10.1016/0012-821X(80)90137-5.
-
Duncan, R. A., 1984. Age progressive volcanism in the New England seamounts and the opening of the central Atlantic Ocean: Journal of Geophysical Research: Solid Earth, 89, B12, 9980–9990, doi:10.1029/JB089iB12p09980.
-
Foster, D.A., Goscombe, B.D., and Gray, D.R., 2009. Rapid exhumation of deep crust in an obliquely convergent orogen: The Kaoko Belt of the Damara Orogen: Tectonics, 28, TC4002, doi:10.1029/2008TC002317.
-
Fromm, T., L. Planert, W. Jokat, T. Ryberg, J. H. Behrmann, M.H. Weber, and Christian Haberland, South Atlantic opening: A plume-induced breakup?, Geology, 43, 931-934, 2015.
-
Gee, J.S., and Kent, D.V., 2007. Source of oceanic magnetic anomalies and the geomagnetic polarity time scale, in Kono, M., Treatise on Geophysics, Volume 5: Geomagnetism: Amsterdam, Elsevier, 455–507.
-
Hay, W., and Sibuet, J., 1984. Initial Reports of the Deep Sea Drilling Project: vol. 75: Washington D.C., U.S. Government Printing Office, 295–445.
-
Hirsch, K., Bauer, K., and Scheck-Wenderoth, M., 2009, Deep structure of the western South African passive margin—Results of a combined approach of seismic, gravity and isostatic investigations: Tectonophysics, 470, p. 57–70, doi:10.1016/j.tecto.2008.04.028.
-
Jokat, W., Boebel, T., König, M., and Meyer, U., 2003. Timing and geometry of early Gondwana breakup: Journal of Geophysical Research, 108, doi:10.1029/2002JB001802,2003.
-
Korenaga, J., Holbrook, W., Kent, G., Kelemen, P., Detrick, R., Larsen, H. C., Hopper, J., and Dahl-Jensen, T., 2000, Crustal structure of the southeast Greenland margin from joint refraction and reflection seismic tomography, Journal of Geophysical Research, 105(B9), 21591–21614, 2000.
-
Morgan, W.J., 1971, Convection plumes in the lower mantle: Nature, 230, 42–43, doi:10.1038/230042a0, doi:10.1038/230042a0.
-
O’Connor, J., and Duncan, R., 1990, Evolution of the Walvis Ridge-Rio Grande rise hot spot systen: Implications for African and South American plate motions over plumes: Journal of Geophysical Research, 95, B11, p. 17475–17502, doi:10.1029/JB095iB11p17475.
-
Pérez-Díaz, L., and Eagles, G., 2014, Constraining South Atlantic growth with seafloor spreading data: Tectonics, 33, p. 1848–1873, doi:10.1002/2014TC003644.
-
Ryberg, T., Haberland, C., Haberlau, T., Weber, M. H., Bauer, K., Behrmann, J. H., and Jokat, W., 2015, Crustal structure of northwest Namibia: Evidence for plume-rift-continent interaction, Geology, 43(8), 739-742, 10.1130/G36768.1
-
Schinkel, J., 2006. Tiefenstruktur der Kontinent-Ozean-Grenze vor dem Orange Fluss, Namibia. [MA Thesis]: Jena, Germany, Friedrich-Schiller-Universitaet.
-
Sleep, N.H., 2006, Mantle plumes from top to bottom: Earth-Science Reviews, 77, p. 231–271, doi:10.1016/j.earscirev.2006.03.007.
-
Sobolev, S.V., Sobolev, A.V., Kuzmin, D.V., Krivolutskaya, N.A., Petrunin, A.G., Arndt, N.T., Radko, V.A., and Vasiliev, Y.R., 2011, Linking mantle plumes, large igneous provinces and environmental catastrophes: Nature, 477, p. 312–316, doi:10.1038/nature10385.
-
Tan, K.-K., Thorpe, R.B., and Zhao, Z., 2011, On predicting mantle mushroom plumes: Geoscience Frontiers, 2, 223–235, doi:10.1016/j.gsf.2011.03.001.
-
White, R. and McKenzie, D., 1989. Magmatism at rift zones: The generation of volcanic continental margins and flood basalts: Journal of Geophysical Research: Solid Earth, 94, B6, 7685–7729.
-
Zelt, C. A. and Smith, R. B., 1992, Seismic traveltime inversion for 2-D crustal velocity structure, Geophysical Journal International, 108(1), 16--34, 10.1111/j.1365-246X.1992.tb00836.x
last updated 13th
January, 2016 |