|
The Headless Tristan da Cunha Mantle Plume |
Eduardo R.V. Rocha Júniora,b,c, Leila S. Marquesa, Fábio B. Machadod, &
Antônio J.R. Nardye
aInstituto de Astronomia, Geofísica e Ciências Atmosféricas, Universidade de São Paulo, São Paulo, SP 05508-090, Brazil, eduardofisica@yahoo.com.br ; leila.marques@usp.br
bInstituto de Geociências, Universidade Federal do Rio Grande do Sul, Porto Alegre, RS 91501-970, Brazil
cInstituto de Geociências, Universidade de São Paulo, São Paulo, SP 05422-970, Brazil (from June 1, 2013)
dDepartamento de Ciências Exatas e da Terra, Universidade Federal de São Paulo, Diadema, SP, Brazil, fabio.machado@unifesp.br
eInstituto de Geociências e Ciências Exatas, Universidade Estadual Paulista, Rio Claro, SP, Brazil, nardy@rc.unesp.br
This webpage is a summary of:
- Rocha-Júnior, E.R.V., Puchtel, I.S., Marques, L.S., Walker, R.J., Machado, F.B., Nardy, A.J.R., Babinski, M., Figueiredo, A.M.G., 2012. Re-Os isotope and highly siderophile element systematics of the Paraná Continental Flood Basalts (Brazil). Earth Planet. Sci. Lett., 337-338, 164-173,
- Rocha-Júnior, E.R.V., Marques, L.S., Babinski, M., Nardy, A.J.R., Figueiredo, A.M.G., Machado, F.B., 2013 (in press). Sr-Nd-Pb isotopic constraints on the nature of the mantle sources involved in the genesis of the high-Ti tholeiites from Northern Paraná Continental Flood Basalts (Brazil). J. South American Earth Sciences, 46, 9-25.
1. Introduction
Continental Flood Basalts (CFB) represent major, short-lived igneous events on Earth, and are responsible for the addition of new juvenile material to the continental crust (White & McKenzie, 1989). CFB are thought by some to form as a result of impingement of a rising mantle plume head on sub-continental lithospheric mantle (SCLM, e.g., Coffin & Eldholm, 1994), and by others to be more directly linked to heterogeneous sources present either in the lithospheric mantle (e.g., Bellieni et al., 1984) or in the shallow mantle (e.g., Meibom & Anderson, 2003). Their origin remains a subject of controversy.
Isotopic data for CFB range widely (e.g., Carlson, 1995) reflecting a complex petrogenesis that may include:
- an original magma source in plumes rising from the deep mantle;
- contamination of asthenospheric magmas by the continental lithosphere;
- overprinting by wall rock contamination in crustal magma chambers;
- partial melting of enriched, metasomatized portions of the SCLM.
For example, detailed geochemical and isotopic studies of Mesozoic Gondwana CFB, such as the Central Atlantic Magmatic Province (CAMP), Karoo, Ferrar and Paraná, indicate derivation from heterogeneous source regions, but with overall characteristics typified by low-143Nd/144Nd, high-87Sr/86Sr, and high-207Pb/206Pb, at a given 206Pb/204Pb, relative to ambient convecting upper mantle (e.g., Merle et al., 2011; Molzahn et al., 1996). The origins of the enriched isotopic compositions commonly observed in CFB are uncertain, as the isotopic contrasts between some possible sources, such as ancient SCLM and postulated plume sources, are not sufficient to discriminate and assign respective proportions.
In addition, the geographic locations of CFB are not random but invariably associated with ancient mobile belts (Lustrino, 2005). This may be considered to be evidence for strong structural control of both the crustal and mantle portions of the lithosphere in channeling the CFB feeding systems.
2. The Paraná Continental Flood Basalt (PCFB) province
The opening of the South Atlantic was preceded by voluminous magmatism in Brazil and Namibia, giving rise to the Paraná-Etendeka magmatic province. This is one of the largest continental volcanic provinces of the world. The PCFB province was emplaced in the Early Cretaceous on top of a large intracratonic Paleozoic sedimentary basin (Paraná Basin) that had been subsiding since the Early Paleozoic. The crystalline basement probably formed by accretion of Archean and Paleoproterozoic cratonic terrains, as well as Neoproterozoic mobile belts related to the Pan-African and Brasiliano orogenies that were responsible for the assembly of West Gondwana (Holz et al., 2006).
The PCFB covers an area of about 1,200,000 km2 and comprises 780,000 km3 of extrusives (Piccirillo & Melfi, 1988; Figure 1). In addition there was intrusive magmatism, represented by sills, which outcrop mainly at Eastern and Northeastern Paraná Basin, and dyke swarms such as Ponta Grossa, Serra do Mar, and Florianópolis.
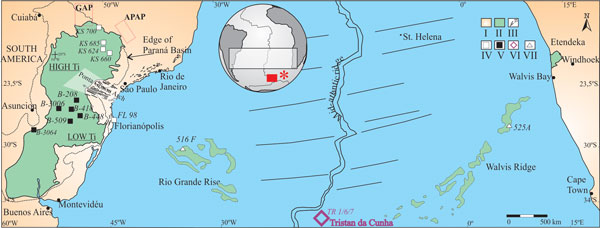
Figure 1: Generalized geological map of the PCFB. (I) crystalline basement, (II) PCFB, (III) dykes occurrence, (IV) high-Ti basalts, (V) low-Ti basalts, (VI) Tristan da Cunha samples, (VII) Rio Grande Rise and Walvis Ridge sample locations. Red asterisk in the inset represents the 40–50°S segment of the South Atlantic ridge. From Rocha-Júnior et al. (2012). Click here or on image for enlargement.
Geochronological data (based on the 40Ar/39Ar method) show that the main volcanic activity occurred 133-132 Ma (Renne et al., 1992; Turner et al., 1994). Most recently, Thiede & Vasconcelos (2010) placed PCFB extrusion at 134.6 ± 0.6 Ma, using new K decay constants, concluding that the duration of PCFB volcanism was < 1.2 Ma. Janasi et al. (2011) reported the first U-Pb baddeleyite/zircon dates on felsic volcanic rocks from the PCFB, with an age of 134.3 ± 0.8 Ma.
Geological and geochemical data have encouraged the division of the PCFB province into two main regions:
- the southern PCFB province, which is characterized by tholeiitic basalts with low-TiO2 (LTi; TiO2 ≤ 2%) and low concentrations of incompatible elements and light rare earth elements; and
- the northern PCFB province, which is characterized by high concentration of TiO2 (HTi; TiO2 > 2%) and incompatible elements.
3. Our perspective on the origin of the PCFB province
There are many more difficulties than certainties in models invoking a Tristan da Cunha plume as the source material for PCFB (Rocha-Júnior et al., 2012). In that study, the possible influence of an ancient sub-continental lithospheric mantle (SCLM), a Tristan da Cunha plume (OIB – ocean island basalt) or an asthenospheric mantle source (depleted mantle) on the formation of the PCFB was investigated based on new Re-Os isotopic data. These showed that exclusive melting of either ancient SCLM or a mantle-plume cannot satisfactorily explain the overall chemical and isotopic features of the PCFB. On a plot of 187Re/188Os vs. 187Os/188Os, the Re-Os data for 11 samples of both high- and low-Ti basalts form a linear trend with a slope that corresponds to a Model 3 ISOPLOT age of 131.6 ± 2.3 Ma and an initial 187Os/188Os = 0.1295 ± 0.0018 (γ187Os = +2.7±1.4). For the regression age of 131.6 Ma, the initial γOs values calculated for individual samples range only from +1.5 to +4.3. These data indicate remarkably little variation in initial 187Os/188Os within the suite.
To characterize the mantle sources involved in PCFB genesis and account for the chemical and isotopic observations, the involvement of asthenospheric mantle and two enriched mantle sources (EM-I and EM-II) are required. The asthenospheric component was enriched by fluids and/or magmas related to Neoproterozoic subduction. The new, combined HSE and lithophile element geochemical data for the PCFB indicate that the high- and low-Ti basalts were derived from magmas that originated from an asthenosphere-like source (similar to arc-mantle peridotite) variably enriched in EM-I and EM-II respectively (Figure 2). EM-I has low 87Sr/86Sr (< 0,706) and 206Pb/204Pb (< 18) (Carlson, 1995) similar to either an ancient, recycled lower continental crustal component, or a metasomatized SCLM component. EM-II has more radiogenic Sr (87Sr/86Sr > 0.706), and Pb (206Pb/204Pb ≈ 18.8) (Carlson, 1995). EM-II ultimately derives from the upper continental crust probably through the subduction and recycling of pelagic sediments or metasomatized (i.e., fluid/melt infiltration) oceanic lithosphere.
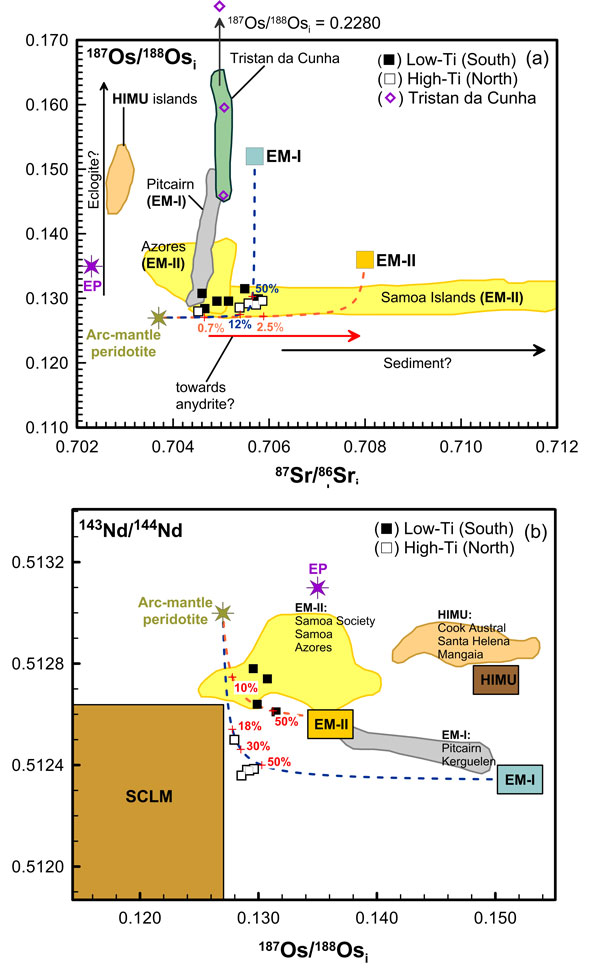
Figure 2: (a) Initial 187Os/188Os (at 131.6 Ma) plotted against initial 87Sr/86Sr. Modeling assumes two-component mixing between sublithospheric mantle (represented by arc-mantle peridotite) and two enriched components. Model (1) is EM-I (blue curve). Model (2) is EM-II (orange curve). (b) Initial 143Nd/144Nd plotted against initial 187Os/188Os. Modeling assumes two-component mixing between depleted mantle-derived melts (represented by arc-mantle peridotite) and two enriched components. Model (1) is EM-I (blue curve). Model (2) is EM-II (orange curve). The parameters used for modeling are found in Rocha-Júnior et al. (2012). From Rocha-Júnior et al. (2012).
Rocha-Júnior et al. (2013) summarise a new view on the genesis of the high-Ti basalts from the northern PCFB. The geochemical composition may be explained by fluids and/or small-volume melts related to metasomatic processes. In this context, the source of these magmas was a mixture of sublithospheric peridotite veined and/or interlayered with mafic components (e.g., pyroxenites or eclogites; Figure 3). The sublithospheric mantle (dominating the osmium isotopic compositions) was probably enriched by fluids and/or magmas related to Neoproterozoic subduction. This sublithospheric mantle region may have been frozen and coupled to the base of the Parana basin lithospheric plate. Note that the sources of the high-Ti basalts of the PCFB, as well as alkaline rocks that surround the Paraná Basin and oceanic basalts with DUPAL signatures appear to be related to EM-I component. Our favored explanation is that the EM-I ‘flavour’ associated to these magmatic events derived from mixtures of eclogites or pyroxenite with peridotite, since pyroxenite melts freeze and react entirely with the ambient peridotite.
What was the triggering mechanism for mantle melting? Ernesto et al. (2002) proposed a non-plume model using geochemical, isotope, paleomagnetic, and geoid anomaly data. In this model, magmatism was triggered by a large thermal anomaly, located at the coast of Western Africa, over which Paraná Basin remained almost stationary for about 50 Ma. The Tristan da Cunha plume hypothesis has also been disputed by Rocha-Júnior et al. (2012) based on osmium isotopic data, in which evidence for the participation of a Tristan da Cunha plume is lacking. The first osmium isotope and HSE abundance data for alkaline rocks from Tristan da Cunha was also presented by those authors. The initial 187Os/188Os ratios of the three basalts, calculated for 1 Ma, vary from 0.146 to 0.228 (initial γ187Os values range from +15 to +80). All three samples are considerably more radiogenic than PCFB source. Thus, PCFB magmatism may be attributed to local hotter mantle conditions due, for example, to the combined effects of edge-driven convection (King & Anderson, 1998) and large-scale mantle warming (Coltice et al., 2007) beneath Pangea. The proximity of the PCFB magmatism to the craton margins (e.g., São Francisco, Congo, Rio de la Plata, Amazon) are consistent with the “edge-effect” mantle flow model, where the small-scale flow is driven by a discontinuity in the thickness of the lithosphere.
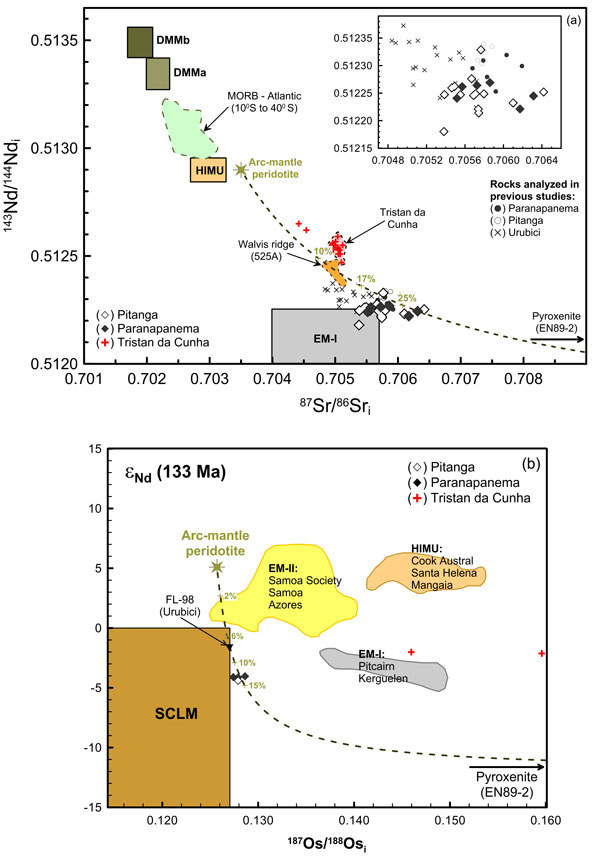
Figure 3: (a) Initial 87Sr/86Sr (at 133 Ma) plotted against initial 143Nd/144Nd. Modeling assumes two-component mixing between sublithospheric mantle (represented by arc-mantle peridotite) and a hypothetical “mafic vein” material (pyroxenite EN89-2). (b) 187Os/188Os plotted against initial εNd. Modeling assumes two-component mixing between sublithospheric mantle (represented by arc-mantle peridotite) and a hypothetical “mafic vein” material (pyroxenite EN89-2). The parameters used for modeling are found in Rocha-Júnior et al. (2013). From Rocha-Júnior et al. (2013).
The Geology Service of Brazil (CPRM) and the Japan Agency for Marine-Earth Science and Technology (JAMSTEC) recently announced the discovery of granite at the bottom of the Atlantic Ocean, about 900 miles (1,500 km) off the coast of Rio de Janeiro (Brazil), in the Rio Grande Rise. The Rio Grande Rise and Walvis Ridge in the South Atlantic Ocean have been interpreted as the trace of a plume currently beneath the islands of the Tristan da Cunha group and Gough (Figure 1), and this postulated plume has been linked the Paraná and Etendeka flood basalts (e.g., Gallagher & Hawkesworth, 1994). How, in this model, can granites be explained?
More likely, the Rio Grande Rise, an aseismic ridge, formed as a result of local decompression and melting due to stress release along several transform fault segments (e.g., Fairhead & Wilson, 2005; Ussami et al., 2012). In addition, the geochemical and isotopic signatures of Walvis Ridge and Rio Grande basalts may be explained by the remelting of detached continental lithospheric mantle left behind during the continental break-up processes (e.g., Hawkesworth et al., 1986). According to this model, the aseismic ridges are mixtures of delaminated enriched subcontinental lithosphere and more typical “normal” oceanic compositions lying within the oceanic mantle array. Ussami et al. (2012) present a review of the processes that led to the formation of the Rio Grande Rise and Walvis Ridge. In this context, could the granite found in the Rio Grande Rise be a piece of continental crust–a microcontinent broken off during South Atlantic opening, or blocks carried to the surface by eruptives?
References
-
Bellieni, G., Comin-Chiaramonti, P., Marques, L.S., Melfi, A.J., Nardy, A.J.R., Piccirilo, E.M., Roisenberg, A., 1984. High- and low-TiO2 flood basalts from the Paraná plateau (Brazil): petrology and geochemical aspects bearing on their mantle origin. Neues Jahrbuch für Mineralogie, 150, 273-306.
-
Carlson, R.W., 1995. Isotopic inferences on the chemical structure of the mantle. J. Geodynamics, 20, 365-386.
-
Coffin, M.F., Eldholm, O., 1994. Large igneous provinces: crustal structure, dimension, and external consequences. Rev. Geophys., 32, 1-36.
-
Coltice, N., Phillips, B.R., Bertrand, H., Ricard, Y., Rey P., 2007. Global warming of the mantle at the origin of flood basalts over supercontinents. Geology, 35, 391-394.
-
Ernesto, M, Marques, L.S., Piccirillo, E.M., Molina, E.C., Ussami, N., Comin-Chiaramonti, P, Bellieni, G., 2002. Paraná Magmatic Province-Tristan da Cunha plume system: fixed versus mobile plume, petrogenetic considerations and alternative heat sources. J. Volc. Geotherm. Res., 118, 15-36.
-
Fairhead, J. D., Wilson, M. 2005. Plate tectonic processes in the South Atlantic Ocean: Do we need deep mantle plumes? In: Foulger, G. R., Natland, J. H., Presnall, D. C., Anderson, D. L. (eds) Plates, Plumes and Paradigms. Geological Society of America, Special Paper, 388, 537-553.
http://dx.doi.org/10.1130/2005.2388(32)
-
Gallagher, K., Hawkesworth, C.J., 1994. Mantle plumes, continental magmatism and asymmetry in the South Atlantic. Earth Planet. Sci. Lett., 123, 105-117.
-
Hawkesworth, C.J., Mantovani, M.S.M., Taylor, P.N., Palacz, Z., 1986. Evidence from the Parana of South Brazil for a continental contribution to Dupal basalts. Nature, 322, 356-359.
-
Holz, M., Küchle, J., Philipp, R.P., Bischoff, A.P., Arima, N. 2006. Hierarchy of tectonic control on stratigraphic signatures: Base-level changes during the Early Permian in the Paraná Basin, southernmost Brazil. J. South American Earth Sciences, 22, 185-204.
-
Janasi, V.A., Freitas, V.A., Heaman, L.H., 2011. The onset of flood basalt volcanism, Northern Paraná Basin, Brazil: A precise U–Pb baddeleyite/zircon age for a Chapecó-type dacite. Earth Planet. Sci. Lett., 302, 147-153.
-
King, S.D., Anderson, D.L., 1998. Edge-driven convection. Earth Planet. Sci. Lett., 160, 289-296.
-
Lustrino, M., 2005. How the delamination and detachment of lower crust can influence basaltic magmatismo. Earth-Science Reviews, 72, 21-38.
-
Meibom, A., Anderson, D.L., 2003. The statistical upper mantle assemblage. Earth Planet. Sci. Lett., 217, 123-139.
-
Merle, R., Marzoli, A., Bertrand, H., Reisberg, L., Verati, C., Zimmermann, C., Chiaradia, M., Bellieni, G., Ernesto, M., 2011. 40Ar/39Ar ages and Sr-Nd-Pb-Os geochemistry of CAMP tholeiites from Western Maranhão basin (NE Brazil). Lithos, 122, 137-151.
-
Molzahn, M., Reisberg, L., Wörner, G., 1996. Os, Sr, Nd, Pb, O isotope and trace element data from the Ferrar flood basalts, Antarctica: evidence for an enriched subcontinental lithosphere source. Earth Planet. Sci. Lett., 144, 529-546.
-
Piccirillo, E.M., Melfi, A.J., 1988. The Mesozoic Flood Volcanism of the Paraná Basin: Petrogenetic and geophysical aspects. Universidade de São Paulo, São Paulo. 600 pp.
-
Renne, P.R., Ernesto, M., Pacca, I.G., Coe, R.S., Glen, J., Prev, M., Perrin, M., 1992. The age of Parana flood volcanism, rifting of Gondwanaland, and the Jurassic – Cretaceous boundary. Science, 258, 975-979.
-
Rocha-Júnior, E.R.V., Puchtel, I.S., Marques, L.S., Walker, R.J., Machado, F.B., Nardy, A.J.R., Babinski, M., Figueiredo, A.M.G., 2012. Re-Os isotope and highly siderophile element systematics of the Paraná Continental Flood Basalts (Brazil). Earth Planet. Sci. Lett., 337-338, 164-173.
-
Rocha-Júnior, E.R.V., Marques, L.S., Babinski, M., Nardy, A.J.R., Figueiredo, A.M.G., Machado, F.B., 2013. Sr-Nd-Pb isotopic constraints on the nature of the mantle sources involved in the genesis of the high-Ti tholeiites from Northern Paraná Continental Flood Basalts (Brazil). J. South American Earth Sciences, 46, 9-25.
-
Thiede, D.S., Vasconcelos, P.M., 2010. Paraná flood basalts: Rapid extrusion hypothesis confirmed by new 40Ar/39Ar results. Geology, 38, 747-750.
-
Turner, S., Regelous, M., Kelley, S., Hawkesworth, C.J., Mantovani, M., 1994. Magmatism and continental break-up in the South Atlantic: high precision 40Ar-39Ar geochronology. Earth Planet. Sci. Lett., 121, 333-348.
-
Ussami, N., Chaves, C.A.M., Marques, L.S., Ernesto, M., 2012. Origin of the Rio Grande Rise-Walvis Ridge reviewed integrating palaeogeographic reconstruction, isotope geochemistry and flexural modelling. Geological Society, London, Special Publications, 369.
-
White, R., McKenzie, D.J., 1989. Magmatism at rift zones: the generation of volcanic continental margins and flood basalts. J. Geophys. Res., 94, 7685-7729.
last updated 30th
May, 2013 |