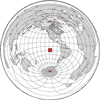 |
Rayleigh
Wave Tomography Beneath Intraplate Volcanic Ridges
in the South Pacific |
Dayanthie
S. Weeraratne1,
Don
W. Forsyth2, Yingjie Yang2 & Spahr
C. Webb3
1California State University, Northridge,
CA 91330-8266, dsw@csun.edu
2Department of Geological
Sciences, Brown University, Providence, Rhode Island,
USA, donald_forsyth@brown.edu; yingjie.yang@colorado.edu
3Lamont-Doherty Earth Observatory,
Columbia University, Palisades, New York, USA, scw@ldeo.columbia.edu
For more details, see: Weeraratne, D. S., D. W. Forsyth,
Y. Yang, and S. C. Webb (2007), Rayleigh wave tomography
beneath intraplate volcanic ridges in the South Pacific, J.
Geophys. Res., 112, B06303, doi:10.1029/2006JB004403.
Introduction
Intraplate seamount chains and volcanic
ridges are the surface expression of the dynamic interaction
between the oceanic asthenosphere and a mobile tectonic
plate. One class of such volcanic features is associated
with gravity lineations west of the East Pacific Rise
that are aligned in the direction of Pacific plate
motion in the hotspot coordinate frame [e.g.,
Sandwell et al., 1995]. A group of such seamount
chains and ridges lies in the south Pacific on 3 -
8 Ma seafloor. They include the well-developed Sojourn
ridge and the actively forming Hotu Matua volcanic
complex (Figure 1). These two linear volcanic trends,
and the Pukapuka ridge to the south [Ed: see
also Pukapuka
page],
are parallel to plate motion and spaced about 200 km
apart, roughly consistent with the dominant wavelength
observed in free-air gravity lineations [e.g.,
Haxby & Weissel, 1986; Cazenave et al.,
1992]. Seamounts and seamount
chains are direct indicators of melt
in the asthenosphere and its transport through the
lithosphere, but the origin of the gravity lineations,
the mechanism of melt generation, and the relationship
of the volcanic activity to the gravity lineations
are debated.
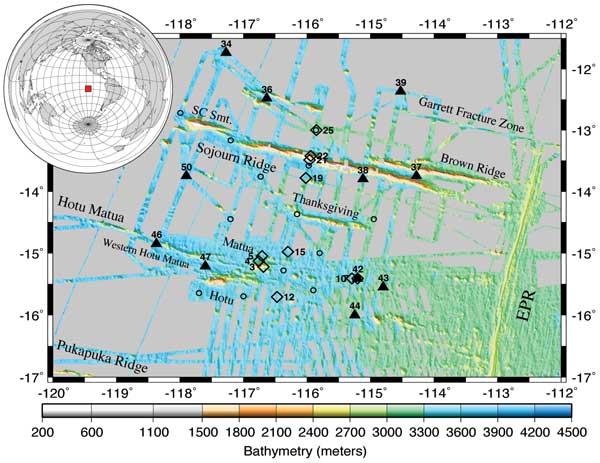
Figure 1: Bathymetric
map of the study area, primarily from the GLIMPSE and MELT experiment
surveys. Inset indicates the experiment location
in the south Pacific. The Southern Cross seamount
(SC Smt) was the tallest volcanic seamount we surveyed.
The Garrett Fracture zone intersects the EPR at about
13.25° and extends to the west
with only subtle relief. OBS deployment sites are
shown which recovered data for the refraction and
microearthquake studies (open diamonds), long term
deployment (filled triangles) and those which did
not return useful data (small open circles).
The GLIMPSE experiment
The Gravity
Lineations and Intraplate Melting Petrology and Seismic
Expedition (GLIMPSE) was
designed to test competing models for the origin
of these volcanic ridges and gravity lineations.
Methods used included petrological and geochemical
analyses of dredged basalts, refraction/wide-angle
reflection measurements for crustal thickness variations,
shipboard measurements of gravity, bathymetry and
side-scan reflectivity, and probing of upper mantle
structure using seismic tomography. The 12-month
duration of the deployment from November 2001 to
December 2002 and the low instrument noise enabled
us to record 155 earthquakes which had Rayleigh waves
with good signal-to-noise ratio and azimuthal distribution
for measurement of dispersion, anisotropy, attenuation,
and derivation of the 3D velocity structure of the
oceanic mantle.
Candidate models
We considered five models to explain
these ocean floor features (Figure 2):
- We dismissed the suggestion that mini-hotspots
are responsible for the chains of ridges and seamounts
because the age progression found by dating basalts
dredged from the Pukapuka ridge indicates propagation
that is several times faster than Pacific plate
motion [Sandwell et al.,
1995; Janney
et al.,
2000].
- Small-scale convective rolls, proposed to be
responsible for the gravity lineations [Haxby & Weissel,
1986] form as negatively buoyant instabilities
drip from a cooling and thickening lower thermal
boundary layer [Jha & Parmentier,
1997; Ed: see also Small-scale
convection page]
or as instabilities within a melt-rich asthenosphere
[Schmeling,
2000], that are organized into linear rolls by
shear in the asthenosphere between the plate and
the deeper mantle [Richter & Parsons,
1975]. Recent numerical work on convective cooling,
however, suggests that convective instabilities
may form at young ages but require mantle viscosities
as low as 1017 Pa s [Zaranek & Parmentier,
2004].
- Tectonic mechanisms such as lithospheric extension
or boudinage (Figure 2b; Ed: see also Pukapuka
page) are expected to allow
pre-existing melt to percolate up through lithospheric
cracks on the seafloor [e.g., Winterer & Sandwell,
1987]. Lithospheric extension should thin the plate
creating somewhat lower velocities. The amount
of extension required to produce the gravity lineations
is of the order of 10%. However, recent studies
indicated that the possible extension is much
less than this [Goodwillie & Parsons,
1992; Gans et al., 2003].
- Thermoelastic bending stresses can produce
lithospheric failure in the troughs of plate undulations
[Gans et al., 2003; Sandwell & Fialko, 2004].
In this case, a downward deflection of
lithosphere and underlying asthenosphere of a few
100 m is predicted beneath the ridges but no upwelling
of the mantle matrix (Figure 2c).
- Viscous fingering instabilities may form
in the asthenosphere when hot, volatile-rich
mantle material, such as may be produced from an
off-axis mantle plume, travels to the spreading
ridge through the asthenosphere [Weeraratne
et al., 2003].
Higher water or volatile content and higher temperatures
should cause lower viscosities, lower seismic velocities
and higher melt production as the return flow from
a source such as the south Pacific Super Swell
[e.g.,
McNutt et al., 1998; Montelli et al.,
2004; Ed: see also Superswell page]
rises under a thinning lithosphere [Weeraratne
et al.,
2003]. This model is consistent with geochemical
anomalies observed along spreading
ridge axes at hotspot locations
[e.g., Mahoney
et al., 1994; Schilling et al.,
2003].
These models not necessarily mutually
exclusive.
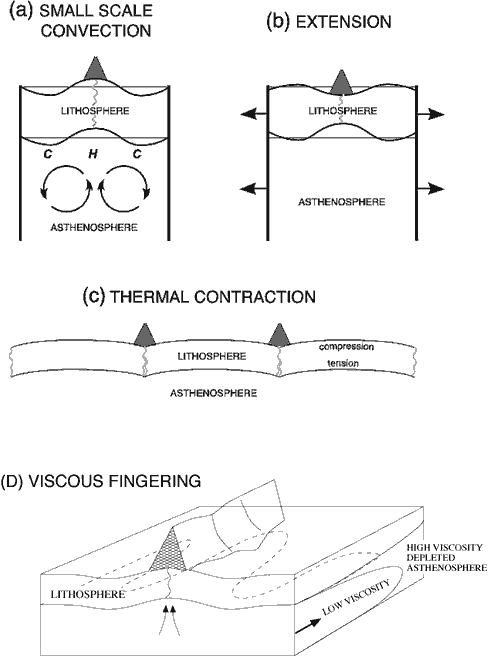
Figure 2: Models to
explain the formation of seamount chains and gravity
lineations in the south Pacific. a) Small scale convection
[Haxby
& Weissel,
1986], b) Lithospheric extension and cracking [Winterer
& Sandwell, 1987], c) Thermoelastic bending and
cracking [Gans et al., 2003], d) Viscous fingering
instabilities involving anomalously low viscosity mantle
intruded into a higher viscosity asthenospheric channel
[Weeraratne et al., 2003]. Each model makes unique
predictions for lithospheric deformation, asthenospheric
flow, and melt generation.
Results
There is a strong negative shear-wave
velocity gradient with its top at about 25 km depth,
beneath which is a minimum shear velocity
of 3.95 km/s at 70 km (Figure 3). We associate the
negative velocity gradient with the base of the lithosphere
averaged over the study area at 40 ± 15 km depth.
The minimum velocity at 70 km is underlain by a sharp
positive velocity gradient extending to ~125 km. We
suggest that the onset of decompression melting causes
the change in shear velocity gradient at ~125 km. Dehydration
associated with increased melting and melt removal
may be responsible for this reversal in gradient.
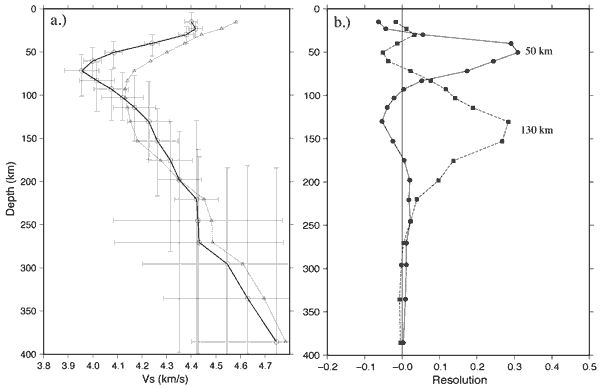
Figure 3: a.) Average phase velocity
as a function of depth (period). These results are
from an inversion that also solves simultaneously
for average anisotropy. Error bars indicate 2 standard
deviations. b.) Dispersion curves from previous studies
for 0 - 4 Ma (solid) and 4 - 20 Ma (dashed).
Anomalously low seismic phase velocities
were observed below the Sojourn Ridge and Hotu Matua
seamount complex at periods up to at least 67 s (corresponding
very roughtly to 67 km depth). High velocities observed
between the chains (Figures 4 &
5) are consistent with active geodynamic models for
seamount formation such as convective rolls or viscous
fingering instabilities.
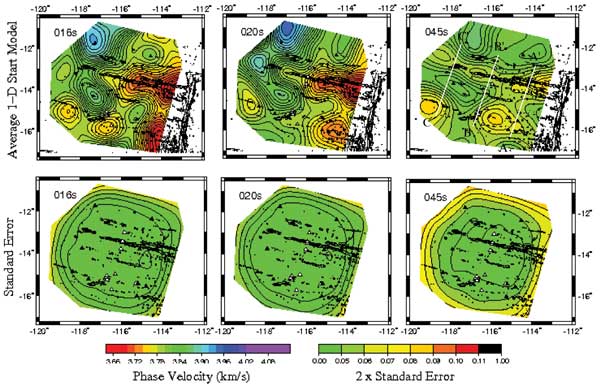
Figure 4: Examples of two dimensional
phase velocity maps for periods of 16 - 45 s.
The starting model is the 1D average phase
velocity result. Standard error contours are shown
in the bottom row and are roughly the same for all
models. Phase velocity variations include trade offs
with anisotropy. Gaussian interpolation (smoothing)
was used with an 80-km length scale. Low velocities
are in red and high velocities in blue. The data
are masked to show information only in the region
considered reliable, based on the distribution of
standard errors shown in the bottom
row. White triangles indicate the temporary refraction
and seismic stations which provide data from
the first month for periods up to ~ 50 s. Black
triangles are station locations for the long-term
deployment. Bathymetry in the top row is contoured
in black. White lines in panel at top right indicate
lines of section shown in Figure 5.
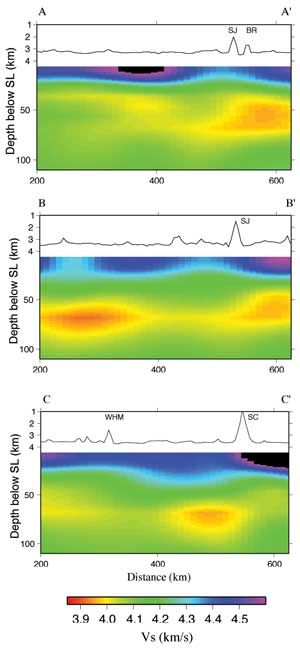
Figure 5: Shear wave velocity cross
sections parallel to the East Pacific Rise. Locations
are shown in Figure 4, top right panel. The depth
scale from 1 - 4 km is exaggerated to show the bathymetry.
SJ: Sojourn ridge, SC: Southern Cross seamount,
WHM: Western Hotu Matua, BR: Brown ridge.
Conclusions
Our
results do not fit predictions of passive tectonic
models such as lithospheric cracking or plate bending
that invoke the tapping of pre-existing, widespread
melt in the asthenosphere. If the convective roll hypothesis
is to fit our data it must be modified to include some
mechanism such as mantle return flow currents that
sweep instabilities that form earlier, back
to the EPR, where low seismic velocities, low Bouguer
gravity anomalies, and small seamounts are observed.
Viscous fingering in the asthenosphere could
introduce compositional anomalies that would vary in
enrichment from west to east and continue all the way
to the EPR spreading center.
References
-
Cazenave, A., S. Houry, B. Lago,
K. Dominh, Geosat-derived geoid anomalies at medium
wavelength, J. Geophys.
Res., 97, 7081-7096, 1992.
-
Gans, K.D., D.S. Wilson, K.C.
Macdonald, Pacific plate gravity lineaments: diffuse
extension or thermal contraction, Geochem.
Geophys. Geosys.,
4, 1074, doi:10.1029/2002GC000465,
2003
-
Goodwillie, A.M., Parsons,
B, Placing bounds on lithospheric
deformation in the central Pacific Ocean, Earth
Planet. Sci. Lett., 111,
123-139, 1992.
-
Haxby, W. F., J.K. Weissel,
Evidence for small-scale mantle convection from
Seasat altimeter data, J.
Geophys. Res., 91, 3507-3520,
1986.
-
Janney, P.E., J.D. Macdougall,
J.H. Natland, M.A. Lynch, Geochemical evidence
from the Pukapuka volcanic ridge system for a shallow
enriched mantle domain beneath the south Pacific
superswell, Earth Planet. Sci.
Lett., 181, 47-60, 2000.
-
Jha, J., E.M. Parmentier, and D.W. Sparks, Buoyant
mantle upwelling and crustal production at oceanic
spreading centers: on-axis segmentation and off-axis
melting, J. Geophys. Res., 102,
11979-11989, 1997.
-
Mahoney, J.J., J.M. Sinton,
M.D. Kurz, J.D. Macdougall, K.J. Spencer, G.W.
Lugmair, Isotope and trace element characteristics
of a super-fast spreading ridge: East Pacific rise,
13-23°, Earth Planet. Sci.
Lett., 121, 173-193, 1994.
-
McNutt, M. K., Superswells, Rev.
Geophys.,
36,
211-244, 1998.
-
Montelli, R., G. Nolet, F.A.
Dahlen, G. Masters, E.R. Engdahl, S.H. Hung, Finite-frequency
tomography reveals a variety of plumes in the mantle, Science,
303, 338-343, 2004.
-
Richter, F. M., and B. Parsons, On the interaction
of two scales of convection in the mantle, J. Geophys.
Res., 80, 2529-2541, 1975.
-
Sandwell, D.T., E.L. Winterer,
J. Mammerickx, R.A. Duncan, M.A. Lynch, D.A.
Levitt, C.L. Johnson, Evidence for diffuse extension
of the Pacific plate from Pukapuka ridges and cross-grain
gravity lineations, J.
Geophys. Res., 100, 15087-15099,
1995.
-
Sandwell, D.T., and Y. Fialko,
Warping and cracking of the Pacific plate by thermal
contraction, J.
Geophys. Res., 109, B10401,
doi:10.1029/2004JB003091, 2004.
-
Schilling, J.G., D.
Fontignie, J. Blichert-Toft, R. Kingsley, and
U. Tomza, Pb-Jf-Nd-Sr isotope variations along
the Galapagos spreading center (10-83°) constraints
on the dispersal of the Galapagos mantle plume, Geochem.
Geophys. Geosys., 4,
10, 8512, doi:10.1029/2002GC000495, 2003.
-
Schmeling, H., Partial melting
and melt segregation in a convecting mantle, in Physics
and Chemistry of Partially Molten Rocks In: N.
Bagdassarov, D. Laporte, A.B. Thompson (eds.),
Kluwer Academic Publisher, Dordrecht, 2000.
-
Weeraratne, D.S., E.M. Parmentier,
D.W. Forsyth, Viscous fingering instabilities
in miscible fluids and the oceanic asthenosphere, EOS
Trans. AGU,
San Francisco, California, 2003.
-
Winterer, E.L., and D.T. Sandwell,
Evidence from en-echelon cross-grain ridges for
tensional cracks in the Pacific plate, Nature, 329,
1987.
-
Zaranek, S.E., and E.M. Parmentier,
Convective cooling of an initially stably stratified
fluid with temperature-dependent viscosity: Implications
for the role of solid-state convection in planetary
evolution, J. Geophys.
Res., 109, B03409, doi:10.1029/2003JB002462,
2004.
last updated 27th
September, 2008
|