 |
|
Domal
uplift and volcanism in a collision zone without
a mantle plume:
Evidence
from Eastern Anatolia |
|
|
Mehmet Keskin
Istanbul University, Faculty of Engineering, Dept.
of Geological Engineering, 34850 Avcilar,
Istanbul, Turkey.
April 2005
keskin@istanbul.edu.tr
Click here to
download a PDF version of this webpage
|
Abstract
The
Eastern Anatolia region is one of the best examples
of a continental collision zone in the world. It also
comprises one of the high plateaus of the Alpine-Himalaya
mountain belt with an average elevation of ~2 km above
sea level (Fig. 1). It displays
shallow and diffuse seismicity (Fig.
2), indicating that the lithosphere is still being
actively deformed as a result of diffuse north-south
shortening. This implies that the collision is still
in progress. Previous studies have shown that the Arabian
plate made its initial contact with the Eurasian plate
during the Late Eocene. The region underwent compressional
tectonic evolution subsequently, but most of it lay
beneath sea level during a period between the Late Eocene
(~50 Ma) and Serravalian (~13 Ma). At about 13 Ma, the
region was subjected to abrupt block uplift and consequently
elevated above sea level. Uplift was followed
by subaerial volcanic activity. Volcanism intensified
and had became widespread all over the region by about
7-8 Ma, while the region gradually acquired a regional
domal shape comparable to that of the
Ethiopian
High Plateau. However, the dome structure in Eastern
Anatolia has a north-south shortened asymmetrical shape,
due to the compressional tectonic regime created by
collision, in contrast to that of the Ethiopian High
Plateau. At present,
it is difficult to recognise the dome in topographic
maps since the topography of the region has been strongly
modified by volcanoes and river drainage systems.
Volcanism
migrated to the south/southeast over time. Great volumes
of volcanic material (i.e. lavas and pyroclastic units)
reaching over 1 km in thickness in places were erupted
onto the surface between 8 and 1.5 Ma, forming volcano-sedimentary
successions, and covering almost two-thirds of the region.
Thus, the Eastern Anatolia region can be regarded as
the site of a "melting anomaly" or "hotspot"
resembling closely the setting proposed for mantle plumes.
However, geologic and geochemical data provide evidence
against a plume origin. In addition, the results of
new geophysical studies, coupled with geologic and geochemical
findings, support the view that both domal uplift and
extensive magma generation can be linked to the mechanical
removal of a portion or the whole thickness of the mantle
lithosphere, accompanied by passive upwelling of normal-temperature
asthenospheric mantle to a depth as shallow as 50 km.
This process is argued to have occurred either by delamination,
slab-steepening and breakoff, or a combination of both.
Therefore, magma generation beneath Eastern Anatolia
may have been controlled by adiabatic decompression
of the asthenosphere. The presence of a subduction component
and thus water in the asthenospheric mantle wedge whould
have played an important role in this melting process.
In addition,
material derived from previously subducted slabs might
have contributed to the fertility of the mantle source
region.
The
Eastern Anatolian example is important in showing that
not
only plumes but also shallow plate tectonic processes
have the potential to generate regional domal structures
in the Earth's lithosphere as well as large volumes
of magma, as proposed by a number of recent studies. |
Introduction
Orogenic belts formed by collisions between continents
contain invaluable records of the geological history
of the Earth and therefore have always attracted the
attention of Earth scientists. The Eastern Anatolia
Region, exhibiting plateau morphology with an elevation
1500 – 2000 m above sea level, is one of two regions
where active continent-continent collision is currently
taking place, the other being the Tibetan Plateau (Fig.
1). Therefore, the Eastern Anatolia region is a
spectacular natural laboratory where the early stages
of a continent-continent collision and their effects
can be thoroughly studied.
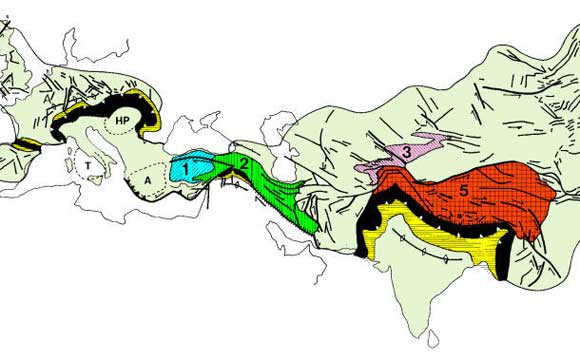
Figure
1. Plateaus in the Alpine/Himalayan mountain belt.
Black: thrust belts; yellow:
foreland and hinterland basins. Numbers refer to the
average height of the plateaus.
1: Western Anatolian plateau (1 km);
2: Eastern Anatolian Plateau (2 km);
3: Tien Shan (3 km);
5: Tibet (5 km)
[Fig. 1 from Dewey et al., 1986].
Figure
2. Distribution of earthquake epicentres, magnitudes
and depths across the Eastern Anatolia region. The map
includes recorded earthquakes from 1st December
1999 to 23rd March 2005. The figure is a
screenshot from the Earthquake Monitor program of Gezdirici
[2001]. Red circles are hypocentres which are shallower
than 20 km (see figure legend).
Previous
studies to date [e.g., Sengor & Kidd, 1979; Dewey et al., 1986] have shown that collision
occurred between the Eurasian and Arabian continents,
resulting in the formation of an extensive (~ 150,000
km2) high plateau with an average elevation
of 2 km above sea level (Fig. 3).
These studies also revealed that the region has reached
this elevation as a block since the Serravalian (~ 13-11
Ma: Gelati, 1975), when the terminal collision
of Arabia with Eurasia started [Sengor & Kidd,
1979].
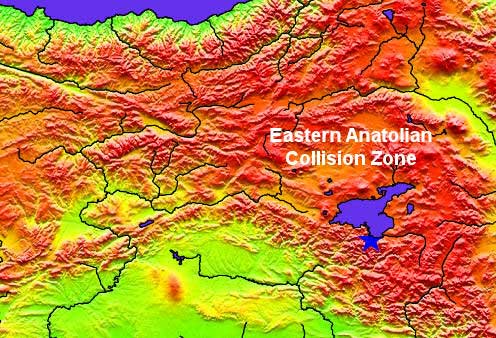
Figure
3. Topographic map showing the Eastern Anatolian plateau
with an average elevation of 2 km above sea level. See
Figs. 4, 5
and 6 for the main tectonic
lines and stratigraphic units.
Volcanic
activity initiated immediately after the rapid block
uplift of Eastern Anatolia and became widespread all
over the region, producing subaerial lava flows and
pyroclastic products which are very variable in their
composition and eruptive style [Pearce et al.,
1990; Keskin et al., 1998; Yilmaz et al.,
1998]. The volcanic activity initiated in the north
around the Erzurum-Kars Plateau and migrated to the
south-southeast [Keskin, 2003] (Fig.
7). A vast volume of volcanic material was produced
by this activity, covering almost two thirds of the
region and reaching over 1 km in thickness in some localities
(Figs. 4, 5
and 6).
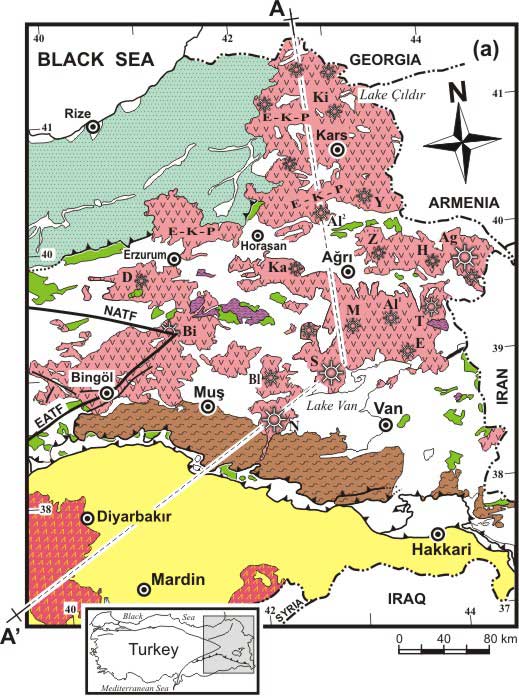
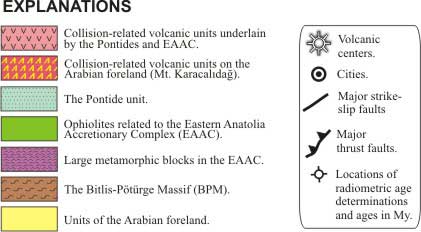
Figure
4. Simplified geological map of the Eastern Anatolia
region showing tectonic units, collision-related volcanic
products and volcanic centres (compiled by Keskin, 2003).
A-A’: direction of the cross section given in Fig.
8. E-K-P: the Erzurum-Kars Plateau;
NATF and EATF: North and East Anatolian Transform Faults.
Volcanic centers: Ag: Mt. Agri (Ararat), Al1:
Mt. Aladag (SE of Agri), Al2: Mt. Aladag
(NW of Horasan), Bi: Mt. Bingol, Bl: Mt. Bilicandagi,
D: Mt. Dumanlidag, E: Mt. Etrusk, H: Mt. Hamadag, K:
Mt. Karatepe, Ki: Mt. Kisirdag, M: Mt. Meydandag, N:
Mt. Nemrut, S: Mt. Suphan, T: Mt. Tendürek, Y: Mt. Yaglicadag,
Z: Mt. Ziyaretdag.
Although
fissure eruptions dominated the volcanic activity, there
are over 20 volcanic centres (e.g., Mt. Nemrut,
Mt. Ararat, Mt. Tendurek) in the region, corresponding
basically to central eruption sites (Figs.
4 and 9). The erupted volumes
may represent only a small fraction of the melt generated
beneath the region, because a greater proportion presumably
was emplaced deeper in the crust as plutonic intrusions.
Thus, there must have been enormous magma generation
beneath the whole region related to the collision of
Arabia with Eurasia. As a result, Eastern Anatolia can
be regarded as one of the Earth's major “hotspots”
or a "melting anomalies".
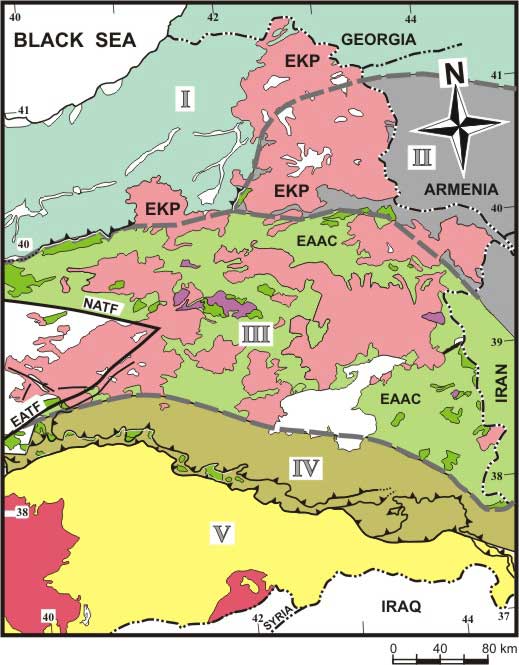
Figure 5. Major tectonic
blocks of the Eastern Anatolia region. The borders are
modified from Sengor et al. [2003]. I: Rhodope-Pontide
fragment, II: Northwest Iranian fragment, III: Eastern
Anatolian Accretionary Complex (EAAC), IV: Bitlis-Poturge
Massif, V: Arabian foreland. Dark green areas: outcrops
of ophilitic melange, Pink and red areas: collision-related
volcanic units, white areas: undifferentiated units
or young cover formations. EKP: the Erzurum-Kars Plateau
in the north.
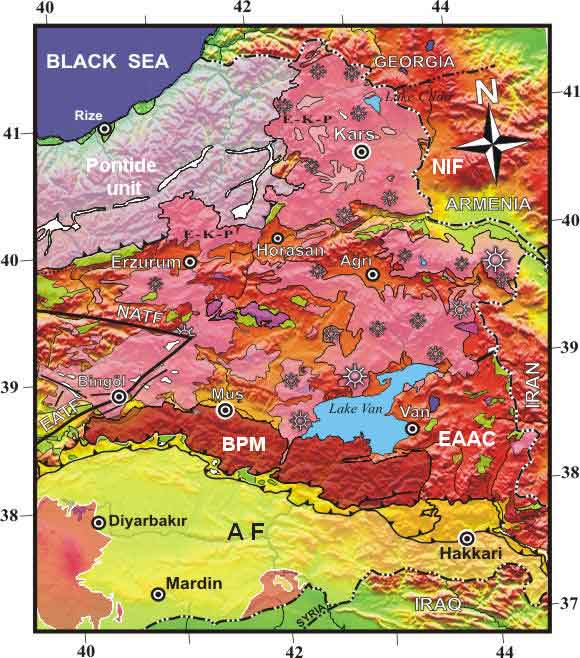
Figure 6. Topographic
map of the Eastern Anatolia Collision Zone (EACZ) over
which the main tectonic units as well as collision-related
volcanics are superimposed. NIF: Northwest Iranian Fragment,
BPM: Bitlis-Poturge massif, EAAC: Eastern Anatolian
Accretionary Complex, AF: Arabian Foreland. For more
explanation, see Figs. 4 and
5.
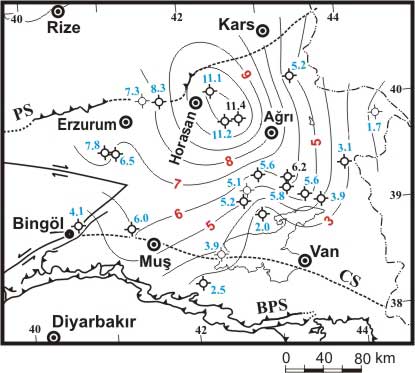
Figure
7. Distribution of the oldest radiometric ages of the
volcanic units. Ages are from Pearce et al. [1990],
Ercan et al. [1990] and Keskin et al. [1998]. Initiation
ages of the volcanism are contoured in 1-Myr intervals.
PS: Pontide suture, BPS: Bitlis-Poturge suture, CS:
inferred cryptic suture between the EAAC and BPS. Figure
from Keskin [2003].
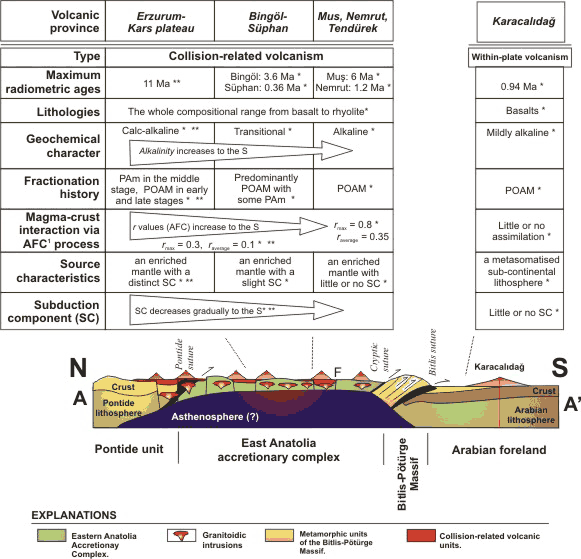
Figure
8. Cross section summarizing the crustal structure and
petrologic/geochemical properties of the collision-related
volcanic units across the Eastern Anatolia Region [Keskin,
2003]. The crustal and lithospheric thicknesses are
from Sengor et al. [2003] and Zor et al. [2003]. The
direction of the cross section (A-A’) is shown in Fig.
4 . Source of geochemical data: *Ercan et al. [1990],
**Pearce et al. [1990], ***Keskin et al. [1998]. SC:
subduction component, AFC: Assimilation combined with
fractional crystallization process, r: ratio of the
rates of mass assimilation and mass crystallization.
F: strike-slip faults.
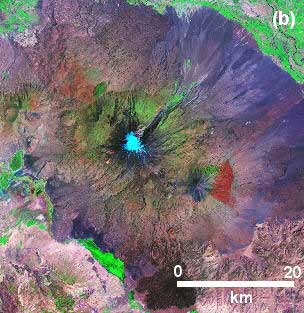
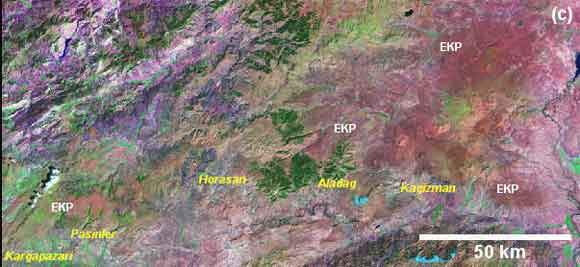
Figure 9. MrSID satellite view of (a) Mt. Nemrut
volcano in the south, (b) Mt. Ararat: a double-peaked
strato-volcano in the northeast, and (c) the Erzurum-Kars
Plateau (EKP) in the northernmost part of the Eastern
Anatolia region. On the Erzurum-Kars Plateau (i.e. c)
Reddish coloured areas marked EKP correspond to volcanic
units, while purple to pinkish areas are either basement
units (e.g., areas in the northwest) or young sedimentary
cover formations. Vegetation is represented by green
areas. For the exact regional locations of Mt. Nemrut
and Mt. Ararat, see Fig. 4.
The
East Anatolian topographic uplift resembles the Tibetan
Plateau and has been viewed as a younger version of
it in many studies [e.g., Sengor & Kidd,
1979; Dewey et al., 1986; Barazangi,
1989]. In these studies, the Eastern Anatolian lithosphere
is thought to have doubled in thickness (to ~ 250-300
km) as a result of collision (Fig.
10). However, recent geophysical studies have revealed
that the mantle lithosphere is almost completely absent
beneath a greater portion of the region [Gök et
al., 2000, 2003; Al-Lazki et al., 2003]
(Fig. 8).
Moreover, studies of receiver functions indicate that
the crust beneath the region ranges in thickness between
38 and 50 km, averaging ~ 40–45 km [Zor et
al., 2003]. This indicates that an almost normal-thickness
crust is underlain by an extremely thin mantle lithosphere
or perhaps almost directly by the asthenosphere. Such
a lithospheric thickness can be considered to be normal
in extensional areas, such as Iceland, but unusual in
a continental collision setting with a compressional
tectonic regime.
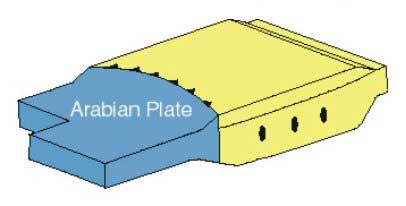 |
Figure
10: Continental collision and subsequent thickening
of the Anatolian crust/lithosphere [Dewey et al.,
1986]. |
On
the basis of these results and the geology of the region,
Sengor et al. [2003] proposed that the East
Anatolian high plateau is a mantle-supported, north-south
shortened domal structure, whose E-W topographic
profile along the 40°N parallel is very similar
to that of the Ethiopian High Plateau (Fig.
11).
Figure
11. Figure 4 from Sengor et al. [2003].
Comparison of the topography of Ethiopia with
an E-W profile along the 40° parallel in
Eastern Anatolia. The smooth lines represent
least squares simplifications of the topography.
|
When
these findings and interpretations are taken into account,
it can be argued that Eastern Anatolia represents a
tectonically-deformed, N-S shortened lithospheric dome
structure, supported by an asthenospheric upwelling
(see cross section in Fig. 8).
Thus, Eastern Anatolia closely resembles a mantle plume
setting. However, geologic and geochemical data indicate
that a mantle plume setting cannot be a viable model
for the region as I discuss in the following sections.
The
rest of this web-page deals with a number of problems
including:
-
how great volumes
of collision-related magma were generated in the region,
-
how and why the region
gained its elevation and the aforementioned domal
shape in the absence of a mantle plume, and
-
what tectonic processes
are responsible for both magma generation and the
regional uplift.
It
is organized as follows:
- Section
I focuses on the geology of the region,
- Section
II deals with the geochemical characteristics of the
collision-related volcanic units,
- Section
III describes the results of the Eastern
Turkey Seismic Experiment project,
- Section
IV discusses ten competing geodynamic models proposed
for the region with emphasis on the inherent discrepancies
in each model.
- Section
V is a discussion.
|
1.
Geology
There are two main plateaus in the Alpine-Himalayan
collision system (Fig. 1):
-
The Anatolian –
Iranian plateau (1 and 2 in Fig.
1),
- The
Tibetan plateau (5 in Fig. 1)
[Sengor & Kidd, 1979; Dewey et al.,
1986].
The Anatolian – Iranian Plateau extends from Eastern
Anatolia to Eastern Iran, and typically has an elevation
of about 1.5 – 2 km in Eastern Anatolia. The basement
of the Anatolian – Iranian Plateau is made up
of micro-continents, accreted to each other during the
Late Cretaceous to Early Tertiary [Sengor,
1990]. These micro-continents are separated from each
other by ophiolite belts and accretionary complexes.
Five different
tectonic blocks
are recognised in North-Eastern Anatolia (Fig.
5):
- The Eastern Rhodope-Pontide fragment
in the northwest of the region (I in Fig.
5). It underlies the south-western and
north-eastern parts of the Erzurum Kars Plateau (i.e.
EKP in Fig. 5).
- The Northwest
Iranian fragment (II in Fig. 5).
The eastern part of the Erzurum-Kars Plateau (i.e.
Horasan, Aladag, Kagizman, Kars areas and Mt. Ararat)
overlies this tectonic block [Keskin et
al., 1998],
-
The Eastern Anatolian Accretionary Complex in the
middle of the region located between the Aras River
and the Bitlis-Poturge Massif (III in Fig.
5),
-
The Bitlis-Pötürge unit which is exposed along the
Taurus belt (IV in Fig. 5),
and
-
Autochthonous units of the Arabian continent or foreland
(V in Fig. 5).
Except
for the EAAC, all the tectonic blocks correspond to
the aforementioned micro-continents.
The Eastern Rhodope-Pontide
unit is located in the northernmost part of the region.
Its basement is represented by a metamorphic massive
named the Pulur Complex [Topuz et al., 2004].
The Pulur complex is composed of a heterogeneous set
of granulite facies rocks, ranging from quartz-rich
mesocratic gneisses to silica- and alkali-deficient,
Fe-, Mg- and Al-rich melanocratic rocks [Topuz et
al., 2004]. A thick volcano-sedimentary arc sequence
overlies this metamorphic basement. This sequence is
regarded as an ensialic, south-facing magmatic arc,
formed by north-dipping subduction under the Eurasian
continental margin [Yilmaz et al., 1997] in
a period between the Albian and Oligocene [Sengor
et al., 2003].
The Northwest Iranian
fragment is masked by collision-related volcanic units
in Eastern Anatolia. It is exposed in Armenia around
the Tsakhkuniats basement outcrop and Hankavan-Takarly
and Agveran massifs [Karapetian et al., 2001].
The unit is composed of a heterogeneous rock sequence,
consisting of trondhjemitic, phyillitic, albite-plagiogranitic,
plagiogranite- and granite-migmatitic lithologies [Karapetian
et al., 2001].
The Eastern Anatolian
Accretionary Complex (EAAC) forms a 150-180 km wide,
NW-SE extending belt in the middle of the region. It
represents the remnant of a huge subduction-accretion
complex formed on a north-dipping subduction zone located
between the Rhodop-Pontide in the north and the Bitlis-Poturge
microcontinent in the south in a period between the
Late Cretaceous and Oligocene [Sengor et al.,
2003]. It consists of two contrasting rock units:
-
An
ophiolitic melange of Late Cretaceous age, and
-
Paleocene
to Late Oligocene flysch sequences incorporated
into the ophiolitic melange as north-dipping tectonic
slices. These flysch slices become younger from
north to south and shallower from the Cretaceous
to the Oligocene [Sengor et al., 2003].
This observation is consistent with the polarity
of the subduction zone that is thought to have created
the Eastern Anatolian accretionary prism by underthrusting.
The Bitlis-Poturge Massif is exposed in a NW-SE extending belt along the
Eastern Taurus mountain range. It is regarded as the
easternmost extremity of the Menderes-Taurus block.
It consists of medium-to-highly metamorphosed units.
Shallow marine
deposits of Oligocene to Middle Miocene age unconformably
overlie these tectonic blocks in some places (not shown
in Figs. 4 and 5).
Collision-related volcanic units, on the other hand,
unconformably overlie both these five tectonic blocks
and the aforementioned marine deposits, masking the
basement units over great distances (Figs.
4, 5 and 6).
These volcanic units become younger to the south/southeast
[Keskin, 2003] (Fig. 7).
|
2.
Lithospheric structure of the region based on the results of the Eastern Turkey Seismic Experiment project Results
from the Eastern Turkey
Seismic Experiment project [ETSE project:
Al-Lazki et al., 2003; Gök et al.,
2000; 2003; Sandvol et al., 2003] reveal that
the mantle lithosphere is either very thin or absent
beneath a considerable portion of the region between
the Aras river (broadly
corresponding to the southern border of the EKP) in the north and the
Bitlis-Poturge Massif in the south (Fig.
12). Moreover, crustal thicknesses obtained from
receiver function studies indicate a gradual change
from < 38 km in the southeast around the southern
part of the Bitlis suture zone to 50 km in the north
beneath the Erzurum-Kars Plateau [Zor et al.,
2003], averaging some 45 km. This indicates that an
almost normal-thickness crust overlies an extremely
thin mantle lithosphere or perhaps it directly overlies
the asthenosphere (see also the cross section in Fig.
8).
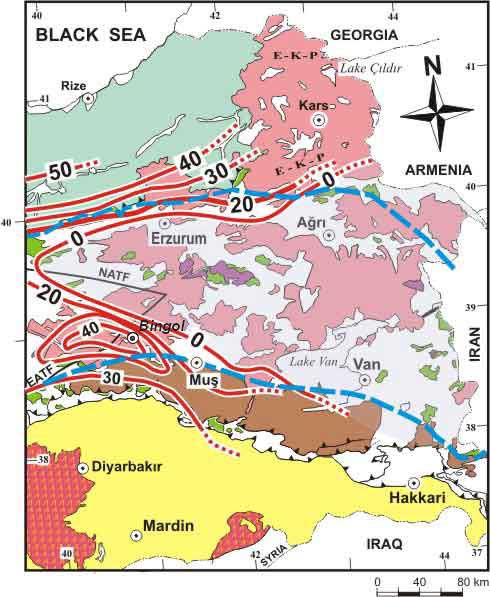
Figure 12.
Contours (red) displaying the mantle lid (i.e.
lithospheric mantle) thicknesses in km (contours
are taken from Fig. 2 of Sengor et al., 2003).
The light bluish-coloured triangular area surrounded
by the cities of Agri, Erzurum, Bingol and Van
in the centre of the figure represents the area
with no mantle lid. Thick, dotted dark-blue lines
represent the northern and southern borders of
the Eastern Anatolian Accretionary Complex (also
see Fig. 5). Note that
areas of inferred complete lithospheric detachment
almost exactly coincide with the extent of the
Eastern Anatolian Accretionary Complex (i.e. the
EAAC).
|
These results are also consistent with the study of
Hearn & Ni [1994], Maggi et al.
[2002] and Maggi & Priestley [2005], suggesting
that the temperature of the mantle significantly increased
beneath this area. What all these findings may imply
is that a huge portion of the mantle lithosphere was
lost beneath Eastern Anatolia. As the collision-related
volcanic activity is almost coeval with the rapid regional
block uplift at ~ 11–13 Ma, catastrophic delamination
might have been responsible [Keskin et al.,
1998]. |
3.
Geochemical characteristics of the collision-related
volcanic units
One of the most striking
aspects of Eastern Anatolia is the volume and the compositional
variability of collision-related volcanic products erupted
during the Neogene and Quaternary. Over half of the
region is covered with young volcanic units (Figs.
4, 5 and 6),
exceeding 1 km in thickness in places and ranging in
age from 11 Ma to present (Figs.
7 and 8).
3.1.
Classification
Collision-related
volcanic rocks across the region span the whole compositional
range from basalts to rhyolites. There is significant
variation in lava chemistry in the N-S direction between
the Erzurum-Kars Plateau (EKP) in the north and the
Mus-Nemrut-Tendurek volcanoes in the south (Figs.
13 and 14). Volcanic units
of the Erzurum-Kars Plateau are calc-alkaline (they
follow a calc-alkaline trend on the AFM diagram, which
is not shown here), while those of the Mus-Nemrut-Tendurek
volcanoes are alkaline to mildly alkaline in character.
Lavas of the Bingol and Suphan volcanoes display transitional
chemical characteristics [Pearce et al., 1990].
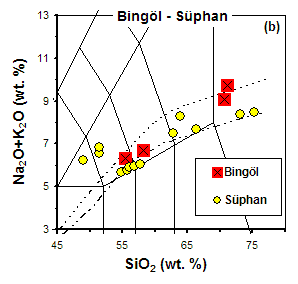
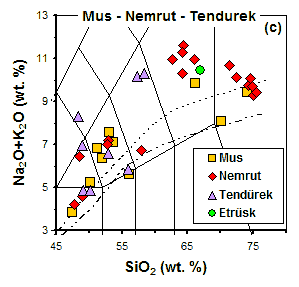 |
Figure
13. Classification of volcanic units of the Eastern
Anatolia region on the total alkali vs silica
diagram of Le Bas et al. [1986]. Data for Erzurum-Kars
Plateau are from Keskin et al. [1998], the rest
are taken from Pearce et al. [1990]. Diagrams
are arranged from north to south:
- Erzurum-Kars plateau
in the north,
- Bingol-Suphan areas
in the central-west,
- Mus-Nemrut-Tendurek
areas in the south.
Abbreviations:
B: basalt, BA: basaltic andesite, TB: trachybasalt,
BTA: basaltic trachyandesite, A: andesite, TA:
trachyandesite, D: dacite, TD: trachydacite, T:
trachite, R: rhyolite, IB: alkaline/subalkaline
divide of Irvine and Baragar [1971], Ku:
alkaline/sub-alkaline divide of Kuno [1966]. Note
that alkalinity increases from north to the south. |
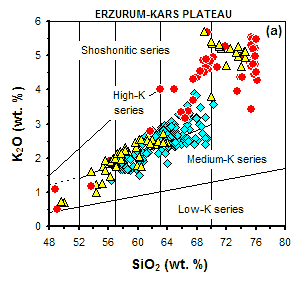 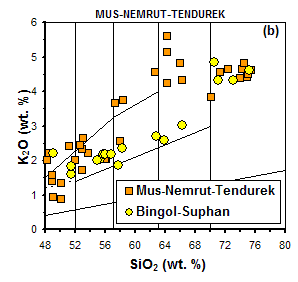
Figure
14. Classification of the volcanic units of the Eastern
Anatolia region on the K2O vs silica diagram
of Peccerillo & Taylor [1976]. Data for Erzurum-Kars
Plateau are from Keskin et al. [1998] and the rest are
from Pearce et al. [1990].
3.2.
Multi-element patterns
Calc-alkaline volcanic units on the EKP and Mt. Ararat
display MORB-normalised patterns typical of continental
arc volcanics. They are likely to have been derived
from an enriched mantle source containing a distinct
subduction signature (SC) (Figs.
15 and 16). This signature
decreases to the south and diminishes around Mus-Nemrut-Tendurek
volcanoes (Figs. 16 and 8),
where the lavas are alkaline and display an intraplate
signature [Pearce et al., 1990].
ERZURUM - KARS
PLATEAU
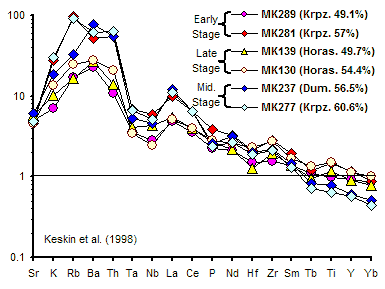
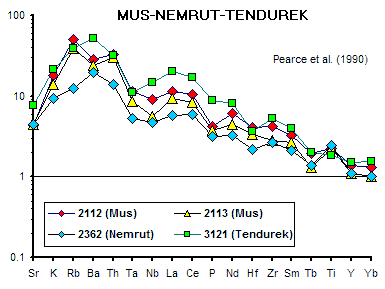 |
Figure
15. MORB-normalised patterns for volcanic samples
from the Eastern Anatolia collision zone. Normalisation
values are from Sun & McDonough (1989).
The data from the Erzurum-Kars Plateau are taken
from Keskin et al. [1998], while the data from
the Mus-Nemrut-Tendurek areas are obtained from
Pearce et al. [1990].
Numbers in brackets
are SiO2 wt. % values.
Note that
the samples from the Erzurum-Kars Plateau in the
north contain a distinct subduction signature,
while lavas of the Mus-Nermrut-Tendurek areas
display an intraplate signature with or without
a slight subduction signature.
|
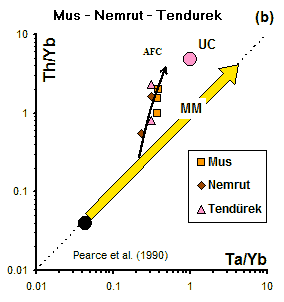
Figure
16. Th/Yb vs. Ta/Yb diagram [after Pearce, 1983] for
basic and intermediate lavas (SiO2 < 60%)
from the Eastern Anatolia Collision Zone. Data from
the Mus-Nemrut-Tendurek volcanoes are from Pearce et
al. [1990]. MM: mantle metasomatism array; SZE: subduction
zone enrichment; WPE: within-plate enrichment; UC: upper
crustal composition of Taylor & McLennan [1985];
FC: fractional crystallsation vector; AFC: assimilation
combined with fractional crsytallisation curve. The
FC curve has been modelled for 50% crystallsation of
an assemblage consisting of 50% plagioclase and 50%
amphibole from a basic magma. The AFC vector has been
drawn for an "r" value of 0.3. Note
that lavas of the Erzurum-Kars Plateau contain a distinct
subduction zone enrichment (SZE) signature.
3.3.
Petrologic modelling
3.3.1. Modelling of source-enrichment
On
a Ta/Yb vs. Th/Yb diagram, calc-alkaline
lavas of the Erzurum-Kars Plateau display a consistent
displacement from the mantle metasomatism array towards
higher Th/Yb ratios, forming a sub-parallel trend to
the main MM array (Fig. 16a).
This suggests that there was a contribution of a subduction
component to the EKP mantle source region. The alkaline
basic lavas of the Mus-Nemrut-Tendurek volcanoes show
a progressive shift from the MM array with increasing
SiO2 (Fig. 16b).
This implies that these lavas might have been derived
from an enriched source with or without a slight subduction
signature and then evolved through combined assimilation-fractional
crystallisation (AFC).
3.3.2.
Modelling fractional crystallisation
Crystallization assemblages in the collision-related
lavas of the Eastern Anatolia region also display variations
across the region. Lavas in the north contain hydrous
assemblages (e.g., amphibole) as well as anhydrous
minerals, whereas those in the south are dominated by
anhydrous minerals. This indicates that lavas are richer
in water in the north than in the south, consistent
with their subduction signature. Geochemical data are
also consistent with these petrographic observations:
the lavas containing hydrous minerals (e.g.,
amphiboles) display distinct depletion with increasing
Rb (Fig. 17a) in contrast to
the lavas of the southern areas (i.e. Mus-Nemrut-Tendurek;
Fig. 17b) which contain anhydrous
minerals that exhibit positive to flat gradients [Pearce
et al., 1990].
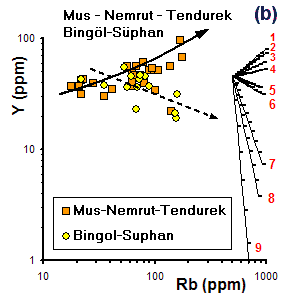
Figure
17. Rb vs. Y diagram displaying theoretical Rayleigh
fractionation vectors for 50% crystallisation of the
phase combinations (given below) from a common magma
composition. Tick marks on each vector correspond to
5% crystallisation intervals. The data for the Erzurum-Kars
Plateau are from Keskin et al. [1998], while those from
the Mus-Nemrut-Tendurek and Bingol-Suphan volcanoes
in the south are from Pearce et al. [1990]. Bulk partition
coefficient values used in the modelling are those given
in Table 2 of Keskin et al. [1998]. The
FC vectors have been modelled using the "FC-Modeler
program" of Keskin [2002].
Phase combinations for
the vectors:
1. plg.5+cpx.3+olv.2
(B); 2. plg.5+cpx.5
(B) or ~plg.5+cpx.3+olv.2
(I); 3. plg.5+amp.5
(B) or plg.5+cpx.5 (I)
4. plg.2+opx.1+cpx.6+olv.1
(I); 5. plg.5+cpx.5
(A); 6. plg.5+amp.5
(I); 7. plg.4+amp.4+gt.2
(I); 8. plg.5+amp.5
(A); 9. plg.4+amp.4+gt.2
(A).
plg: plagioclase,
cpx: clinopyroxene, opx: orthopyroxene, olv: olivine,
amp: amphibole, gt: garnet
B: basic, I: intermediate, and
A: acid magma compositions.
3.3.3.
Modelling AFC process
AFC modelling results indicate that the degree of magma-crust
interaction is larger in the south than in the north
(Fig.18). Radiometric dating
results indicate that volcanic activity began earlier
in the north than in the south, migrating south over
time (Figs. 7 and 8).

Figure
18. Diagrams showing the results of assimilation-fractional
crystallisation (AFC) modelling. The modelling was conducted
using the AFC equations of De Paolo [1981]. Bulk
partition coefficients are inset in the diagrams. Parental
magma compositions correspond to the basaltic sample
MK139 (Erzurum-Kars Plateau; Keskin et al., 1998) and
sample 2362 (Mus-Nemrut-Tendurek: Pearce et al., 1990),
and the average crustal composition of Taylor &
McLennan [1985].
3.3.4.
Modelling partial melting process
Melting modelling (Fig.
19) was carried out using the fractional and batch
melting equations of Shaw [1970], the bulk
partition coefficient values given in the inset of Fig.
19 (for the source of Kd values,
see the caption of Fig. 19)
and modal mineralogy of spinel- and garnet-peridotites
proposed by Wilson [1989] (see the caption
of Fig. 19). The trace element
composition of the garnet-peridotite is taken from Frey
[1980], while the composition of the spinel-peridotite
is the average composition of spinel peridotite xenoliths
(see C0 values in the inset of Fig.
19) in young (i.e., Miocene-Pliocene) alkaline
basalts from the Thrace region, NW Turkey [Esenli
& Genc, submitted]. Most of the lavas of the
EKP plot on the batch-melting curve of the spinel peridotite,
while two of them (MK144: the oldest, 11 Ma, sample
from the bottom of the Horasan area, and three lava
samples from the Middle Stage in the Dumlu area) fall
close to the beginning of the fractional melting curve.
Therefore, magmas that fed the volcanism on the Erzurum-Kars
Plateau seem to have been generated by partial batch
melting of a spinel peridotite mantle source. The degree
of melting might be quite high for the lavas clustering
around the end of the batch-melting curve.
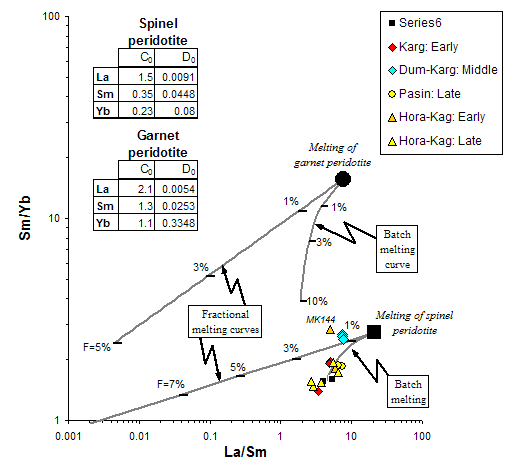
Figure
19. La/Sm vs. Sm/Yb plot showing theoretical melting
curves plotted along with the basic samples (SiO2<57%)
from the Erzurum-Kars Plateau. Fractional and batch
melting equations of Shaw [1970] were used to construct
the melting model. F: weight fraction of melt produced.
Modal mineralogy for the spinel- and garnet-peridotites
are taken from Wilson [1989], and ol.66+opx.24+cpx.08+sp.02
and ol.63+opx.30+cpx.02+gt.05
respectively (ol: olivine, opx:orthopyroxene, cpx: clinopyroxene,
sp: spinel, gt: garnet). Trace element composition of
the spinel-peridotite (C0 values) is the
average composition of spinel peridotite xenoliths in
young (Miocene) alkaline basalts of the Thrace region,
NW Turkey [Esenli & Genc, submitted], while that
of garnet peridotite is from Frey [1980]. Kds
between the basaltic melts and minerals given in the
inset are compiled from Irving & Frey [1978], Fujimaki
et al. [1984], McKenzie & O'Nions [1991] and Rollinson
[1993]. Bulk partition coefficient (Ds) of each element
has been calculated for garnet and spinel peridotite
source rock compositions by taking the modal mineralogy
of these end members into consideration. The coefficients
are given in the inset.
Coherence
of the data points from different stages of the volcanism
in Fig. 19 indicates that the
nature of the mantle source and the mode of the melting
process varied little with time. This is also consistent
with the results obtained from chondrite-normalized
REE and MORB-normalized multi-element patterns (see Fig. 15); basic lavas erupted
during the early and late stages display similar patterns
all over the EKP. Similar modelling was conducted for
the lavas of the Karacadag and Tendurek volcanoes in
the south by Sen et al. [2004], and produced
similar results.
3.4.
Summary of the geophysical and geochemical findings
The geochemical and geophysical findings are presented
together in the cross section in Fig.
8. The geochemical evidence presented so far indicates
that volcanic
products in the north around the EKP and Mt. Ararat
are calc-alkaline in character and likely to have been
derived from an enriched mantle source containing a
distinct subduction signature (Fig.
8). This signature decreases to the south and diminishes
around the Mus-Nemrut-Tendurek volcanoes, where the
lavas are alkaline and display an intraplate signature.
Results from AFC modelling show that the degree of magma-crust
interaction is larger in the south than in the north
(Fig. 18). Radiometric dating
results indicate that volcanic activity begin earlier
in the north than in the south, and migrated south over
time (Fig. 7).
The
striking results of the Eastern
Turkey Seismic Experiment project along
with the geochemical findings discussed above lead us
to question the validity of geodynamic models proposed
for the Eastern Anatolian Collision Zone in a number
of studies reported in the literature. Therefore, prior
to focusing on the issue of what process was responsible
for the loss of mantle lithosphere, I first review the
competing geodynamic models and their discrepancies. |
4.
Competing geodynamic models & their discrepancies
Ten different geodynamic
models have been proposed for the genesis of collision-related
magmatism beneath the Eastern Anatolian collision zone.
Some of the earlier studies
[e.g., the tectonic escape model of McKenzie,
1972, and the lithospheric thickening model of Dewey
et al., 1986] did not address the problem of why
and how huge volumes of magmas were generated beneath
the region. Any geodynamic model proposed for the Eastern
Anatolian collision zone should, however, answer this
critical question since the topographic expression,
tectonic elements and magma generation are clearly all
associated with the same mechanism.
There appear to be inconsistencies
in all models except for the delamination and the slab-steepening
& breakoff models. In what follows, each model is
discussed thoroughly with its weaknesses and strengths.
1.
The tectonic escape of micro-plates to the east
and west [McKenzie, 1972]. |
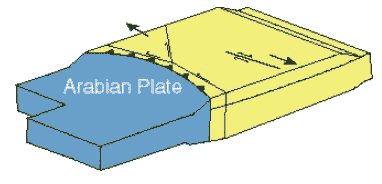
Figure
20
|
Discrepancies: A close examination of the model of McKenzie [1972] reveals that it does not account entirely
for the strain induced by the 2.5 cm/yr convergence
of the Arabian and Eurasian plates [Dewey
et al., 1986]. In addition, this model cannot
explain why and how huge volumes of magma were
generated beneath the region and how the region
was elevated to form an extensive plateau now
2 km above sea level. It also does not provide
an answer to why the lithospheric mantle is absent
beneath a greater portion of Eastern Anatolia. |
2.
Renewed subduction of the Arabian plate beneath
the Pontides and Eastern Anatolia [Rotstein
& Kafka, 1982].
|
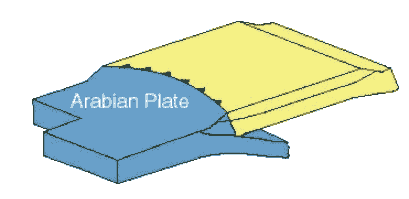
Figure
21
|
Discrepancies:
this model is not supported by any seismic evidence.
There are no seismic data for a north-dipping Benioff
zone beneath the Eastern Anatolia region. Tomographic
images obtained from the ETSE project [Al-Lazki
et al., 2003] indicate that a slab beneath
the region does not exist. |
3.
Detachment and northward movement of a subducting
slab beneath Eastern Anatolia [Innocenti et
al., 1982a,b]. |
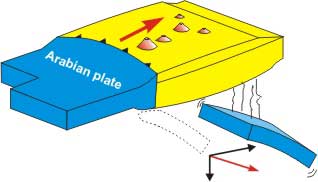
Figure
22
|
On
the basis of their dating results and chemical
zonation in volcanic units across the collision
zone, Innocenti et al. [1982a,b] suggested
that the andesitic volcanic front migrated northward
by 150-200 km during the Pliocene. According to
them, this is evidence for detachment of the subducted
slab immediately after continental collision.
According to their model, the detached slab moved
northward while it was sinking in the asthenosphere.
They suggest that this movement generated progressively
lower intensity magmatism from south to the north.
In their view, volcanism becomes younger from
south to north. In this model, calc-alkaline magmas
that formed the Plio-Quaternary volcanic belt
in the north were generated above the subducting
slab, while the alkaline magmas representing the
Miocene volcanic belt in the south were derived
from the asthenosphere upwelling through the gap
behind the detached subducting slab.
Although
the model of Innocenti et al. [1982a,b]
is one of the earliest, it is remarkable in that
the possibility of slab detachment and consequential
effects in the Eastern Anatolian Collision Zone
were envisaged 13 years earlier than the "slab-breakoff
model" was proposed by Davies & von
Blanckenburg [1995]. The latest geodynamic
model,"slab-steepening & breakoff beneath
a large subduction-accretion complex", by
Keskin [2003] also proposes a similar
slab-detachment process, although the slab in
the model of Keskin [2003] does not move
northward after breakoff but instead steepens
beneath a large subduction-accretion complex until
it breaks off, creating a gradually widening mantle
wedge beneath the region.
Discrepancies: A more detailed study
of collision-related volcanism on the Erzurum-Kars
Plateau [Keskin, 1994], which comprises
the northernmost part of the Eastern Anatolian
volcanic province, has shown that volcanism initiated
at ~ 11 Ma in the north [Keskin et al.,
1998] and then migrated south over time [Keskin,
2003]. These findings are the opposite of what
is proposed by Innocenti et al. [1982a,b].
In addition, there is no seismic evidence for
a currently subducting slab beneath the region. |
4.
Rifting along E-W oriented Late Miocene-Pliocene
basins [Tokel, 1985] possibly accompanied
by decompression melting of "normal asthenosphere"
due to extension [McKenzie & Bickle,
1988]. |
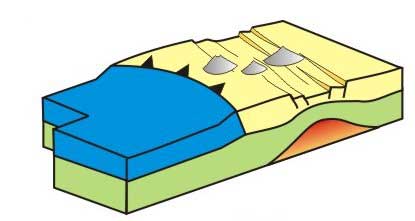
Figure
23
|
Tokel [1985] cited
data from drilling cores gathered from E-W oriented
Upper Miocene-Pliocene basins in Eastern Anatolia.
He argued that these basins are bounded by gravity
faults and are filled with at least 2000 m of
limnic and fluvial deposits intercalated with
voluminous “tholeiitic” and “alkaline” volcanic
products. He suggested that recent tectonics in
Eastern Anatolia were dominated by an extensional
stress regime. On the basis of the mathematical
model of Turcotte [1983], he proposed
that these depressions and the sediments deposited
therein were related to a "rifting event"
in the region.
Discrepancies:
The fault plane solutions of earthquakes in the
region indicate that the faults are either strike
slip or reverse, which is inconsistent with extension(i.e.
a rift setting). A close examination of the E-W
oriented basins in the region reveals that the
they are not rift-related but are, instead, dominantly
pull-apart basins related to strike slip fault
systems.
Decompression melting
of normal asthenosphere as a result of regional
extension [McKenzie & Bickle, 1988]
requires a stretching factor of about 2.5 to generate
melts in dry asthenosphere at a depth of 50 km
and a temperature of around 1280°C. As is
well known the region is not being stretched so
at first sight this does not seem to be a likely
scenario. However, it is now almost certain that
nearly all the mantle lithosphere was detached
from beneath the region and thus, at present,
the lithosphere is much thinner than normal (~
38-50 km). Even if this is the case, however,
it is theoretically difficult to melt dry asthenosphere
in the absence of extension. The asthenospheric
mantle beneath the region is not completely dry,
but instead it contains a distinct subduction
component which increases in importance from
south to north as deduced from the chemistry of
collision-related volcanics in the region [Pearce
et al., 1990; Keskin, 1998]. The
existence of a subduction component (with water)
in the asthenospheric mantle may thus significantly
decrease the melting temperature and permit the
generation of voluminous basic magma at
this depth (38 to 45 km). |
5.
Continental collision and subsequent thickening
of the Anatolian crust/lithosphere [Dewey
et al., 1986]. |
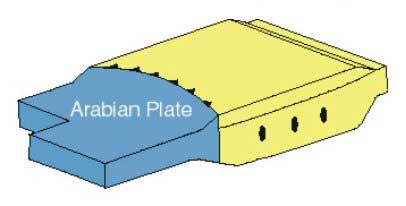
Figure
24 |
Dewey
et al. [1986] argued that the Eastern Anatolia
region owes its high elevation to a doubled (~
300 km) lithospheric thickness. According to them,
this thickening occurred as a result of continental
collision between the Arabian and Eurasian continents.
They also point out that the lavas were erupted
through both N-S cracks that extend into the Arabian
foreland and through transcurrent pull-aparts.
In this model magma generation is linked to local
extension and small-scale delamination events
beneath the pull-apart basins (e.g,.
the Erzincan, Karasu-Pasinler-Horasan and Mus
basins).
Following the model
of Dewey et al. [1986], Yilmaz et
al. [1987] suggested that the young volcanism
in Eastern Anatolia could be linked to heating
of the lower continental crust and mantle lithosphere
which had been subjected to lithospheric thickening.
Similarly, on the basis of their geochemical data,
Koronovskiy & Demina [1996] argued
that heating due to crustal thickening may explain
the young volcanism of the Lesser Caucasus, adjacent
to the Eastern Anatolia region.
Discrepancies: It is now well understood
that the region would not have been isostatically
elevated to ~ 2 km if a 250-300 km thick and dense
(3.2-3.3 g/cm3) mantle lithosphere
had been attached to the base of a lighter (2.7-2.8
gr/cm3) crust [Sengor et al.,
2002; Sengor et al., 2003]. The model
is not supported by recent tomographic data either
[e.g., Al-Lazki et al., 2003; Gok
et al., 2003]. Results of the Eastern
Turkey Seismic Experiment
project indicate that mantle lithosphere beneath
the region is very thin or completely absent over
a great distance in the middle of the region (Fig.
8).
Collision-related
volcanic units are not confined to pull-apart
basins. Instead, they cover a much greater area
away from these basins. This indicates that volcanism
in the region cannot be explained by the pull-apart
model alone.
Pearce et al.
[1990] discuss the point that a 50% increase in
thickness of the metasomatised mantle lithosphere
lowers a significant portion of this layer to
a depth below that of amphibole breakdown, forming
garnet and releasing water. This may initiate
localised melting but it also lowers the geotherm.
When this happens, most of the metasomatised layer
remains significantly below the solidus and thus
does not produce magma [Pearce et al.,
1990]. Therefore, it is difficult to explain the
huge volumes of magma generated in the region
by the models of Yilmaz et al. [1987]
and Koronovskiy & Demina [1996]. |
6.
Hot spot activity related to a mantle plume [discussed
by Pearce et al., 1990]. |
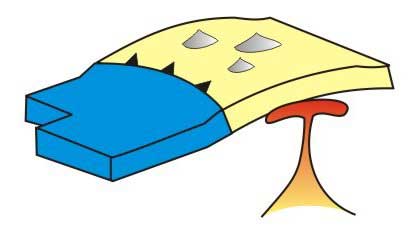
Figure
25 |
The possibility of plume-related
"hot spot" activity in Eastern Anatolia
was previously discussed by Pearce et al.
[1990]. They point out that the remarkable correlation
between topographic and volcanic expressions in
Eastern Anatolia. The Eastern Anatolia topographic
uplift has an asymmetric (i.e. deformed)
dome shape [Sengor et al., 2003] whose
long-axis aligns approximately E-W. The overall
volcanic expression is also asymmetric, extending
about 300 km in the direction of compression but
900 km perpendicular to it [Pearce et al.,
1990]. This remarkable correlation between the
topography and volcanic expression brings into
question whether there is a mantle plume beneath
the Eastern Anatolia Collision Zone.
Sengor et al.
[2003] compared the E-W topographic profile of
the Eastern Anatolian Plateau along the
40° parallel with the plume-generated Ethiopian
High Plateau [Sengor, 2001] and
found a striking similarity between them (Fig.
11). They low-pass filtered both profiles
at 125 km to remove plastic effects. On the basis
of the similarity of the profiles, they argued
that the cause of the domal uplift in both regions
was the same: hot, rising asthenosphere beneath
crust bereft of underlying mantle lithosphere
[Sengor et al., 2003].
Although domal uplift related to
a mantle plume is expected to have a symmetrical
shape, in theory, it may acquire an asymmetrical
shape in a collision setting due to compression.
However, there is no modern or ancient example
anywhere in the world of a plume-related dome
structure deformed by shortening in a collision
zone.
Discrepancies:
Dome structures formed by plumes
are expected to contain fault systems and dyke
swarms distributed radially. Such faults and dykes
are absent in Eastern Anatolia. Fault plane solutions
of earthquakes imply that the faults are either
transform or reverse; not normal as would be expected
in a plume-related domal structure. A plume model
cannot explain why volcanic units contain a distinct
subduction component in the north of Eastern Anatolia,
and why this component gradually diminishes to
the south. It is also difficult to explain by
a plume model why volcanism migrated south with
time, and why there is a gradual change in magma
chemistry from calc-alkaline in the north to alkaline
in the south. As pointed out by Pearce et
al. [1990], volcanic activity over the last
6 Myr displays a temporal change from more regional-scale
activity to localised activity on a set of aligned
central volcanoes. Such an evolutionary sequence
is the reverse of what is expected in plume-related
volcanic activity.
On the basis
of these discrepancies, I argue that a plume setting
is not a viable model for the Eastern Anatolian
Collision Zone. |
7.
Delamination of mantle lithosphere beneath the
region [Pearce et al., 1990; Keskin
et al., 1998]. |
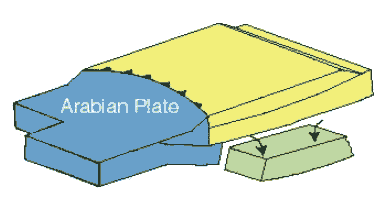
Figure
26 |
Delamination of a thickened
thermal boundary layer is plausible since it is
colder and hence denser than the underlying asthenosphere
[Ed: See also Lithospheric
Delamination page]. It could therefore be
convectively replaced by asthenosphere [Houseman
et al., 1981; England & Houseman,
1988]. Platt & England [1993] argue
that magmatism in mountain belts could be evidence
of delamination of the lower part of the thickened
mantle lithosphere. Figs.
27a and b illustrate
the delamination model in a three-dimensional
block diagram for the Eastern Anatolia region
[modified from Keskin, 1994]. This process
is likely to be an effective mechanism for generating
large volumes of collision-related magma across
the region, since asthenosphere is brought into
close contact with the thickened layer of metasomatised
lithosphere [Pearce et al., 1990]. When
delamination occurs, it causes a perturbation
in what is left of the mantle lithosphere, raising
some parts of it above its solidus. While sinking
into the asthenosphere, the delaminated block
of the mantle lithosphere may release water that
also promotes melting. These two mechanisms play
an important role in the generation of extensive
partial melting in the mantle, and can produce
widespread volcanism in the region (Fig.
27b).
Pearce
et al. [1990] argue that the region is characterised
by a set of mantle domains that run parallel to
the collision zone. They suggest that each domain
has yielded magmas of particular composition since
the beginning of the magmatism in the region.
This may also be regarded as supporting evidence
for the delamination model.
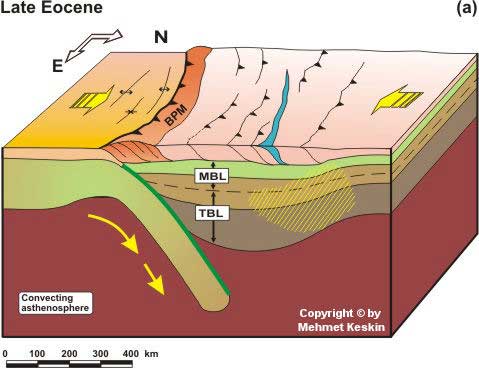
Click
on the figure for enlargement.
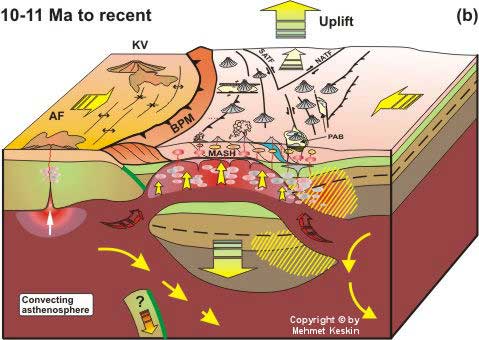
Click
on the figure for enlargement.
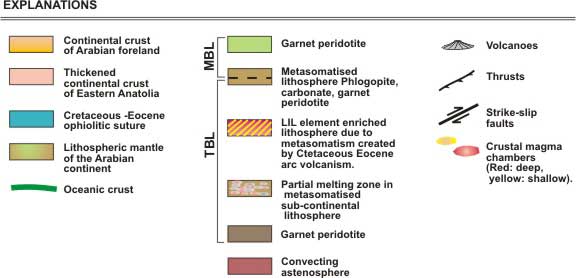
Figure
27. Block diagrams illustrating the delamination
model for the Eastern Anatolian Collision Zone.
Modified from Keskin [1994].
On the basis
of estimates of the active slip rates, total convergence
and timing of collision-related deformation across
the Arabia-Eurasia collision zone, coupled with
the interpretation of a cross-section produced
by the National Iranian Oil Company [1977],
Allen et al. [2004] suggest that the
collision-related magmatism, which initiated at
~ 11 Ma [Keskin et al., 1998] pre-dates
shortening of the crust in the region. Therefore,
they argue, a sudden and regional delamination
event is not be a viable model. However, results
obtained from two independent seismic studies:
-
the Eastern
Turkey Seismic Experiment
Project [Al-Lazki et al., 2003; Gök
et al., 2000; 2003; Sandvol et al.,
2003] and
-
the
Surface Waveform Tomography study of Maggi
& Priestley [2005]
reveal
that most of the Eastern Anatolian Collision Zone
is devoid of a mantle lithosphere. Therefore,
geophysical findings support a major lithospheric
detachment beneath the region and contradict the
interpretation of Allen et al. [2004].
Discrepancies: As
discussed in Section 2, new data obtained from
the Eastern
Turkey Seismic Experiment
indicate that there appears to be no lithospheric
mantle over a greater portion of the area beneath
the region. If this is the case, then the delamination
must have been a shallow event involving the whole
lithospheric mantle and perhaps even the lower
crust. In the absence of metasomatised lithospheric
mantle, the source region would then be asthenospheric
mantle.
Sengor
et al. [2003] point out that the basement
of a great portion of the Eastern Anatolia Region
between the Aras River in the north and Lake Van
in the south is represented by a subduction-accretion
complex (i.e. EAAC in Fig.
12). This area also coincides with the area
under which a lithospheric mantle lid is missing
[Sengor et al., 2003]. In contrast to
continental blocks, large subduction-accretion
complexes are devoid of their own lithospheric
roots, as they are produced on, and supported
by, subducting oceanic slabs. Therefore, in theory,
this area should have been underlain by a subducting
slab, not by sub-continental mantle lithosphere,
before the lithospheric detachment event.
As the delamination
process requires the presence of mantle lithosphere,
what took place beneath the region could not have
been a delamination event. As tomography provides
no evidence for a mantle lid beneath the region,
then the underlying slab must have detached and
sunk into the asthenosphere possibly immediately
prior to the domal uplift of the region at ~ 13
Ma. In view of these arguments, a model
involving steepening and breakoff of a subducting
slab beneath a huge subduction-accretion complex
can explain better the geodynamic evolution of
the Eastern Anatolian Collision Zone [Keskin,
2003; Sengor et al., 2003]. As discussed
below, this model is not only consistent with
the geology of the region but also explains better
the variations in magma age and chemistry across
the region (Fig. 8). |
8.
Localized extension associated with pull-apart
basins in strike-slip systems [Pearce et al.,
1990; Keskin et al., 1998]. |
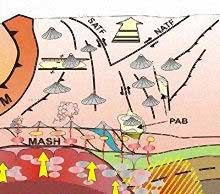
Figure
28 |
In
their pioneering study, Dewey et al.
[1986] highlighted the connection between the
formation of pull-apart basins and volcanism.
They pointed out that there are two different
neotectonic magmatic suites in the region:
- the
nepheline-hypersthene normative alkaline basalts
of mantle origin, and
- the
silicic-to-mafic calc-alkaline suite.
They suggested that
both suites occur in pull-apart basins in strike
slip regimes and N-S extensional fissures. They
argue that the position and shape of magmatic
intrusions might have been controlled by "flaking
of the elastic lid" particularly beneath
the pull-apart basins. They argue that rapid lithospheric
stretching and small-scale delamination beneath
pull-apart basins can generate melting in the
mantle.
Although Pearce
et al. [1990] consider delamination to be
the dominant process that caused voluminous magma
generation beneath the region, they also argue
that it might have been accompanied by other stretching
mechanisms, such as the creation of pull-apart
basins. They also suggested that deviatoric stress
perpendicular to the principal direction of compression
might also have some effect.
Keskin et al.
[1998] emphasised the role of strike-slip faulting
in pull-apart basins in focussing magmas on the
Erzurum-Kars Plateau, north of the region. They
point out that, compared to nearby areas, a much
thicker (2-4 km) sequence of volcanic/volcano-clastic
rocks was deposited in these gradually subsiding
basins. However, it is not clear whether these
faults simply provide fractures that enable magma
to reach the surface or whether the associated
localised extension in pull-apart basins also
encourages melting in the mantle.
More recently Cooper
et al. [2002] suggested a similar model for
the origin of mafic magmas beneath northwestern
Tibet and argued that these lavas might have been
created by mantle upwelling beneath the releasing
bends of the strike-slip fault systems [Ed: see
also Ridge-transform
intersections page].
Discrepancies:
As mentioned the Introduction, collision-related
volcanic units in the region are not confined
to pull-apart basins, but cover a much greater
area. Therefore, it is plausible that a pull-apart
model cannot explain the genesis of all the collision-related
magmatism in the region. Some other mechanism
must also have been operational. |
9.
Inflow of lower crust driven by the isostatic
response to denudation and sedimentation in surrounding
areas [Mitchell & Westaway, 1999]. |
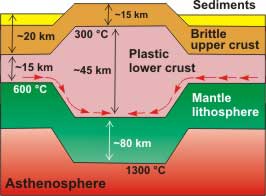
Figure
29 |
On the basis of their study
of Neogene-Quaternary uplift and magmatism in
the Greater Caucasus, Mitchell & Westaway
[1999] proposed an alternative model to explain
the formation of high mountain ranges and plateaus
such as the Greater and Lesser Caucasus including
the Armenian highlands adjacent to Northeastern
Anatolia. They argue that the rate and spatial
scale of uplift of the Caucasus are too great
to be the result of plate convergence alone, and
therefore some other processes must have been
operational.
Mitchell &
Westaway [1999] argue that when crustal material
is hotter than 300°C, it starts to behave
in a ductile way, deforming plastically. The depth
at which this temperature is reached (~ 15-20
km) broadly corresponds to the boundary between
the plastic lower crust and the brittle upper
crust. In the lower crust, the direction of movement
(i.e. direction of flow) is determined
by pressure gradients caused by lateral variations
in the depth of the base of the brittle layer
[Mitchell & Westaway, 1999]. In this
model, most of the crustal deformation occurs
in the lower crust in an atectonic fashion [e.g.
Kaufman & Royden, 1994].
The model of Mitchell
& Westaway [1999] is dramatically different
from the rest of the competing models in that
crustal thickening is not caused directly by
plate motions. Their model involves lateral inflow
of ductile lower crust, driven by the isostatic
response to denudation of a mountain range and
sedimentation in its surroundings. According
to these authors, the start of uplift of the Caucasus
and surrounding areas relates to changes in environmental
conditions in the Late Miocene. The Messinian
drawdown of sea-level in the Mediterranean region
resulted in complete desiccation of the Black
Sea [Giavanoli, 1979]. This was accompanied
by drawdown of Caspian sea level. Not only did
this result in an increase in subaerial relief,
but also in an increase in the denudation rate
of the Greater Caucasus. Coupled denudation and
sedimentation (Fig. 29)
caused lateral inflow into the lower crust towards
the base of the mountain range, resulting in uplift
along the length of the Caucasus.
Mitchell &
Westaway [1999] suggest that atectonic thickening
of the continental crust keeping mantle lithosphere
thickness constant would raise the temperature
in the mantle lithosphere, resulting in melting
and magma generation as suggested by Koronovskiy
& Demina [1996]. They argue that this
process was responsible for both uplift and volcanism
in the Lesser Caucasus, including Armenia, adjacent
to Eastern Anatolia. They also suggest that this
process could be a viable model for Eastern Anatolia
[Rob Westaway, personal communication,
2002].
Discrepancies: In
the model of Mitchell & Westaway
[1999], thickening occurs only in the lower crust
by means of lateral flow driven by plastic deformation.
In such a case, a normal thickness of lithospheric
mantle is still expected beneath the thickened
crust, as there is no reason why it should have
been detached from the base of the crust or along
the thermal boundary layer. However, there is
strong seismic evidence for a major lithospheric
detachment event beneath the region [from the
Eastern Turkey
Seismic Experiment Project Al-Lazki
et al., 2003; Gök et al., 2000;
2003; Sandvol et al., 2003 and the Surface
Waveform Tomography study by Maggi & Priestley,
2005].
Moreover, as previously
discussed, an increase in the thickness of the
lithosphere is not able to generate a significant
amount of magma, as it remains well below its
solidus [Pearce et al., 1990]. Therefore,
the model of Mitchell & Westaway
[1999] is not consistent with new geophysical
findings and fails to explain the volume and variability
of magmatic products across the region. |
10.
Slab steepening and breakoff beneath a subduction-accretion
complex [Keskin, 2003]. |
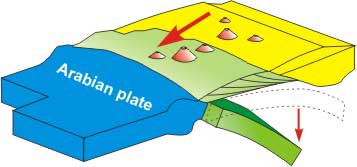
Figure
30 |
Sengor
et al. [2003] pointed out that areas with
no mantle lithosphere, located in the south of
the EKP, coincide broadly with the East Anatolian
Accretionary Complex (EAAC), a huge subduction-accretion
prism of late Cretaceous to earliest Oligocene
age. Following the subduction-accretion hypothesis
[Sengor & Yilmaz, 1981; Sengor
& Natal’in, 1996], Sengor et al.
[2003] argue that the EAAC can be regarded as
a remnant of a large accretionary prism located
between the Pontides and the Bitlis-Pötürge Massif,
having formed on northward-subducting oceanic
lithosphere. Large subduction-accretion complexes
are devoid of lithospheric roots; instead they
are underlain by subducting slabs [Sengor
et al., 2003]. Since this was probably the
case for the EAAC, then shallow delamination could
not be a viable alternative model for collision-related
magma generation beneath the aforementioned portion
of Eastern Anatolia in the absence of sub-continental
lithospheric mantle [Keskin, 2003; Sengor
et al., 2003]. As a subducting slab is also
absent beneath the EAAC, this can be ascribed
to the past breakoff of the inferred slab beneath
the EAAC (Figs. 30 and
4a,b). |
|
5.
Discussion
Keskin [2003]
showed that volcanic activity began earlier in the north
than in the south, migrating south with time (Figs.
7 and 8). This migration
was accompanied by significant variation in lava chemistry
in the N-S direction between the EKP in the north and
the Mus-Nemrut-Tendurek volcanoes in the south. As discussed
earlier in Section 2, volcanic products erupted in the
north around the EKP were calc-alkaline in character
with a distinct subduction signature in contrast to
the ones in the south around the Mus-Nemrut-Tendurek
volcanoes which were alkaline with an intraplate signature
[Pearce et al., 1990]. The volcanic units of
the Bingol and Suphan volcanoes display transitional
chemical characteristics (Fig. 8;
also see Fig. 13).
Keskin [2003]
pointed out that these spatial and temporal variations
in magma genesis, coupled with the uplift history of
the region, can be explained by a model involving steepening
of a northward subducting slab beneath a large subduction-accretion
complex, namely the EAAC, followed by breakoff at around
10-11 Ma. He also argues that the slab, whose subduction
was generating the Pontide arc in the north, was not
attached to the Arabian plate. Instead, it was possibly
attached to the Bitlis-Poturge block before breakoff
(Fig. 31).
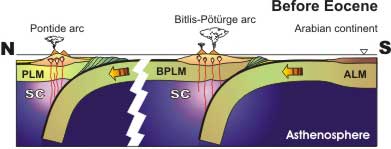 |
Figure
31. PLM, BPLM and ALM: lithospheric
mantle of the Pontides, Bitlis-Poturge Massif,
and Arabian continent respectively. SC: subduction
component. Figures 31 to 37 are from Keskin [2003].
|
The oceanic realm
between the Bitlis-Poturge Massif and the Arabian plate
had been closed much earlier (i.e. in the Late
Eocene; Fig. 32 and 33).
Therefore, it is not surprising that researchers failed
to reach a consensus regarding timing of the collision
event in the region. Tomographic images of the region
provide no evidence for a lithospheric fragment currently
sinking into the asthenosphere beneath the Eastern Anatolia
region. What this may indicate is that the detachment
of the oceanic lithosphere of the Arabian plate took
place in the past, perhaps millions of years ago (i.e.
10-13 Ma; Figs. 33 and 34).
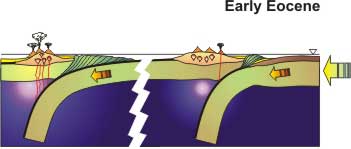 |
Figure
32 |
|
Figure
33. EAAC: the Eastern Anatolian Accretionary Complex. |
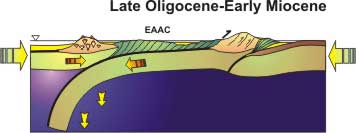 |
Figure
34 |
According to Sengor
et al. [2003], the oceanic realm between the Pontides
and the Bitlis-Poturge Massif was completely closed
in the Oligocene (Figs. 33
and 34). After a period between
the Oligocene and Serravalian (i.e. 13-15 Ma),
during which the EAAC was shortened and thus thickened
over the slab, the hidden subduction possibly stopped
(Fig. 34). As a result, being
left unsupported by subduction, the oceanic lithospheric
slab may have steepened and finally detached from the
EAAC, opening out an asthenospheric mantle wedge, gradually
widening to the south [Keskin, 2003]. This
possibly created suction on the asthenosphere, generating
mantle flow to the south (Figs.
35b and 36). Emplacement
of the asthenospheric mantle with a subduction component
and a potential temperature of 1280°C at shallow depths
(~ 45-50 km) beneath the EAAC would have generated extensive
adiabatic decompression melting. Also, it probably generated
regional block uplift, producing the regional dome-like
structure (Figs. 36 and 37).
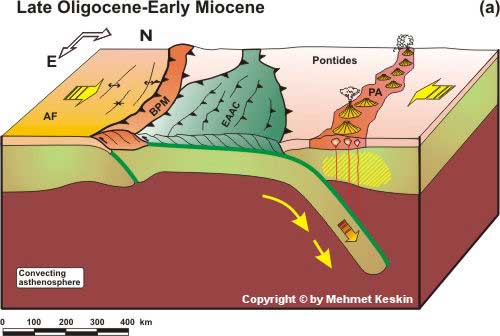
Click
on figure for enlargement.
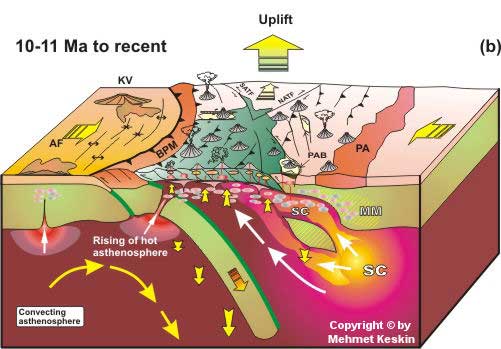
Click
on figure for enlargement.
Figure 35. Block diagrams
illustrating the slab-steepening & breakoff model
for the Eastern Anatolian Collision Zone. Modified from
Keskin [2003]. SC: subduction components. White arrows
indicate the flow direction of the asthenosphere.
The presence of such asthenospheric
flow may provide an answer to the question of why the
volcanic activity initiated much earlier in the north
on the EKP. Similarly, it explains better why the volcanic
products are calc-alkaline with a distinct subduction
signature in the north and this chemical signature changes
gradually to alkaline (i.e. intraplate) to
the south (Figs. 35a and b).
The model proposed for Eastern Anatolia differs from
the original model of Davies & von Blanckenburg
[1995] since it involves a large accretionary complex
and the steepening of the slab beneath it.
A number of recent studies address the
importance of the slab breakoff process in the generation
of magmatism in collision zones [e.g., Maheo et
al., 2002; Maury et al., 2000; Williams
et al., 2004]. The slab-steepening and breakoff
process beneath large subduction-accretion complexes,
accompanied by magma generation and the emplacement
of magmas, may be a very important process in the making
of continental crust in Turkic-type orogenic belts [Sengor
& Natal’in, 1996] that comprise a large part
of the Asian continent. It should be noted that the
slab-steepening & breakoff model is viable only
if the basement of a greater part of the region is represented
by the EAAC as proposed by Sengor et al. [2003],
and if there was only one north-dipping subducting slab
beneath this accretionary prism. As the collision-related
volcanic sequence masks the basement units over great
distances, it is difficult to find evidence that sheds
light on whether this interpretation is correct or not.
|
Concluding
remarks
The Eastern Anatolian
high plateau can be regarded as a hot spot or "melting
anomaly" coinciding with a regional domal structure
which is squeezed in a collision zone in the N-S direction.
By virture of these features, the region closely resembles
a mantle plume setting. However, the Eastern Anatolian
domal uplift lies in a collision zone, in contrast to
plume-related hot spots located in intraplate settings
(e.g., the Ethiopian high plateau).
The Eastern Anatolian
lithosphere is, at present, bereft of its mantle component
beneath a huge region [Sengor et al., 2003].
This indicates that a huge piece (perhaps almost the
whole thickness) of the mantle lithosphere was detached
from the overlying crust in the past. If this removal
of the denser mantle material is responsible for both
the regional uplift and coeval volcanism, then the detachment
must have occurred at about 13 Ma, at the same time
as onset of those events. The volume opened up by the
removal of the mantle lithosphere would have been filled
by a hot, fertile asthenospheric upwelling, which
would result in both the formation of the regional domal
structure [Sengor et al., 2003] and extensive
magma generation and volcanism due to adiabatic decompression
melting [Keskin, 2003].
I suggest that the mantle
source region owed its exceptional fertility either
to a subduction component inherited from a previous
subduction event (i.e. the subduction beneath
the Pontides during the Eocene and Oligocene), to the
oceanic crustal material previously subducted beneath
the region, or to a combination of both. A process similar
to the latter has recently been proposed by a number
of researchers [e.g., Gasparik, 1997; Anderson,
2000; 2004a; Balyshev & Ivanov, 2001; Ivanov,
2003; Foulger et al., 2005] to explain low
velocity anomalies in the mantle as well as the genesis
of magmatism in exceptionally fertile mantle domains
(e.g., the Icelandic hot spot; Foulger
et al., 2005). As pointed out by Anderson
[2004b], melting anomalies can result from fertile patches
or regions of shallow mantle with low melting point,
and this seems to be the case for Eastern Anatolia.
On the basis of combined
geologic, geophysical and geochemical data, I thus argue
that the Eastern Anatolian domal uplift [Sengor
et al., 2003] is not related to a mantle plume.
Instead its formation is linked to plate tectonic processes;
namely either to slab-steepening and breakoff beneath
a subduction-accretion complex [Keskin, 2003;
Sengor et al., 2003] or to lithospheric delamination
[Pearce et al., 1990; Keskin et al.,
1998]. These processes can explain the voluminous magma
generation and resultant volcanism in addition to the
formation of the domal uplift across the region better
than other competing geodynamic models.
The Eastern Anatolian
example is particularly important as it shows that shallow
plate tectonic processes can generate both regional
lithospheric domal structures and great volumes of magma
in the absence of a mantle plume.
This observation contradicts the proposal of Sengor
[2001] who argues that all hotspots and long-wavelength
domes on the Earth's surface are related to mantle plumes.
Temporal and spatial variations
in lava chemistry coupled with the uplift history and
age relationships of the volcanic products in the Eastern
Anatolian Collision Zone may be linked to slab-steepening
and breakoff beneath a subduction-accretion complex
in the south, where the mantle lid is absent (Fig.
35b, also see Fig. 12).
Slab-steepening was possibly associated with asthenospheic
flow that resulted in gradual change in the geochemical
character of the volcanics erupted. I argue that lithospheric
delamination might be a still more viable model for
the northern areas (e.g. the Erzurum-Kars Plateau;
Fig. 35b).
In addition to these two
processes, strike-slip faulting might have played an
important role in focusing magmas by generating localized
extension and volcanism in associated pull-apart basins
[Dewey et al., 1986; Pearce et al.,
1990; Keskin et al., 1998]. In a recent studyCooper
et al. [2002] support this view and suggest that
the mafic magmas beneath NW Tibet might have been created
by a mantle upwelling beneath the releasing bends of
the strike-slip fault systems. They also present a model
for magma generation in such systems. Therefore, like
the Tibetan Plateau, the uplift and magmatism history
of Eastern Anatolia may be related to more than one
geodynamic process [e.g., Williams et al.,
2004].
Further research is needed
for a better understanding of collision-related magma
genesis in Eastern Anatolia and its connection with
slab breakoff and other alternative processes. Issues
regarding source characteristics, melting mechanisms,
the mode and extent of magma-crust interaction and crustal
melting also needs further investigation. |
References
-
Al-Lazki,
A., D. Seber, E. Sandvol, N. Turkelli, R. Mohamad,
and M. Barazangi, Tomographic Pn velocity
and anisotropy structure beneath the Anatolian plateau
(eastern Turkey) and the surrounding regions, Geophys.
Res. Lett., Lett., 30(24),
8043, doi:10.1029/2003GL017391, 2003.
-
Allen, M.,
J. Jackson, and R. Walker, Late Cenozoic reorganization
of the Arabia-Eurasia collision and the comparison
of short-term and long-term deformation rates,
Tectonics, 23, TC2008, doi:10.1029/2003TC001530,
2004.
-
Anderson,
D.L., The thermal state of the upper mantle: no
role for mantle plumes, Geophys. Res. Lett.,
27, 3623-3626, 2000.
-
-
-
Barazangi,
M., Continental collision zones: Seismotectonics
and crustal structure, in Encyclopedia of Solid
Earth Geophysics, edited by D. James, 58-75,
Van Nostrand Reinhold Company, New York, 1989.
-
Balyshev S.O.
and A.V. Ivanov, Low-density anomalies in the mantle:
ascending plumes and/or heated fossil lithospheric
plates? Doklady Earth Sciences, 380,
no: 7, 858-862, 2001.
-
Cooper, K.M.,
M.R. Reid, N.W. Dunbar, and W.C. McIntosh, Origin
of mafic magmas beneath northwestern Tibet: Constraints
from 230Th-238U Disequilibria,
Geochem. Geophys. Geosys., 3/1,
art. no. 1065, 2002.
-
Davies, J.H.,
and F. von Blanckenburg, Slab breakoff: a model
of lithosphere detachment and its test in the magmatism
and deformation of collisional orogens, Earth
Planet. Sci. Lett., 129, 85-102,
1995.
-
De Paolo,
D. J., Trace element and isotopic effects of combined
wall-rock assimilation and fractional crystallisation,
Earth Planet. Sci. Lett., 53,
189-202, 1981.
-
Dewey J.
F., M.R. Hempton, W.S.F. Kidd, F. Saroglu, and A.M.C.
Sengor, Shortening of continental lithosphere: the
neotectonics of Eastern Anatolia – a young
collision zone, in Collision Tectonics, Geological
Society special publications no: 19,
edited by M.P. Coward and A.C. Ries, 3-36, 1986.
-
England,
P.C. and G.A. Houseman, The mechanics of the Tibetan
Plateau, Philos. Trans. Royal. Soc. London,
A326, 301-320, 1988.
-
Ercan, T.,
T. Fujitani, J-I. Madsuda, K. Notsu, S. Tokel, and
U.I. Tadahide, Dogu ve guneydogu Anadolu Neojen-Kuvaterner
volkanitlerine iliskin yeni jeokimyasal, radyometrik
ve izotopik verilerin yorumu, M.T.A. Dergisi,
110, 143-164, 1990.
-
Esenli, F.
and S.C. Genc, Preliminary report on the petrography,
petrology and geochemistry of mantle peridotite
xenoliths in alkali basalts from the Eastern Thrace
region, NW Turkey, Geologica Capratica, submitted.
-
-
-
Frey, F.A.,
The origin of pyroxenites and garnet pyroxenites
from Salt Lake Crater, Oahu, Hawaii: trace element
evidence, Am. J. Sci., 280-A,
427-449, 1980.
-
Fujimaki,
H., M. Tatsumoto, and K. Aoki, Partition coefficients
of Hf, Zr and REE between phenocrysts and groundmasses.
Proceedings of theFourteenth Lunar and Planetary
Science Conference, Part 2, J. Geophys. Res.
Suppl., 89, B662-672, 1984.
-
Gasparik,
T., A model for the layered upper mantle, Phys.
Earth Planet. Int., 100, 197-212,
1997.
-
Gelati, R.,
Miocene marine sequence from Lake Van, Eastern Turkey,
Riv. Ital. Paleont. Stratigr., 81, 477-490,
1975.
-
-
Gök, R.,
E. Sandvol, N. Türkelli, D. Seber, and M. Barazangi,
Sn attenuation in the Anatolian and Iranian
plateau and surrounding regions, Geophys. Res.
Lett., 30(24), 8042, doi:10.1029/2003GL018020,
2003.
-
Gök, R.,
N. Turkelli, E. Sandvol, D. Seber, and M. Barazangi,
Regional wave propogation in Turkey and surrounding
regions, Geophys. Res. Lett., 27(3),
429-432, 2000.
-
Hearn, T.N.,
and J.F. Ni, Pn velocities beneath continental
collision zones: The Turkish-Iranian Plateau,
Geophys. J. Int., 117, 273-283, 1994.
-
Housman,
G.A., D.P. McKenzie, and P. Molnar, Convective instability
of a thickened boundary layer and its relevance
for the thermal evolution of continental collision
belts, J. Geophys. Res., 86,
6115-6132, 1981.
-
Innocenti,
F., P. Manetti, R. Mazzuoli, G. Pasquaré, and L.
Villari, Anatolia and north-western Iran, in Andesites,
Ed. R.S. Thorpe, John Wiley & Sons, 1982a.
-
Innocenti,
F., R. Mazzuoli, G. Pasquaré, F. Radicati di Brozolo,
and L. Villari, Tertiary and Quaternary volcanism
of the Erzurum-Kars area (Eastern Turkey): geochronological
data and geodynamic evolution, J. Volc.
Geotherm. Res., 13, 223-240,
1982b.
-
Irvine, T.N.
and W.R.A. Baragar, A guide to the chemical
classification of the common volcanic rocks, Canadian
J. Earth Sci., 8, 523-548,
1971.
-
Irving, A.J.
and F.A. Frey, Distribution of trace elements between
garnet megacrysts and host volcanic liquidus of
kimberkitic to rhyolitic composition, Geochim.
Cosmochim. Acta, 42, 771-787,
1978.
-
-
Karapetian
, S.G., R.T. Jrbashian, and A.K. Mnatsakanian, Late
collision rhyolitic volcanism in the north-eastern
part of the Armenian Highland, J.
Volc. Geotherm. Res.,
112 (1-4), 189-220, 2001.
-
Kaufman,
P.S. and L.H. Royden, Lower crustal flow in an extensional
setting: Constraints from the Halloran Hills region,
eastern Mojave Desert, California. J. Geophys.
Res., 99, 15723–15739, 1994.
-
Keskin, M.
Genesis of collision-related volcanism on the Erzurum-Kars
Plateau, Northeastern Turkey, Unpublished PhD
thesis, Univ. Durham, U.K., 1994.
-
Keskin, M.,
FC-Modeler: a Microsoft® Excel©
spreadsheet program for modeling Rayleigh fractionation
vectors in closed magmatic systems, Computers &
Geosciences, 28/8, 919-928, 2002.
-
Keskin, M.,
J.A. Pearce, and J.G. Mitchell, Volcano-stratigraphy
and geochemistry of collision-related volcanism
on the Erzurum-Kars Plateau, North Eastern Turkey,
J. Volc. Geotherm.
Res., 85(1-4), 355-404,
1998.
-
Keskin, M.,
J.A. Pearce, P.D. Kempton and P. Greenwood, Magma-crust
interactions and magma plumbing in a collision setting:
Sr-Nd-Pb-O isotope and trace element geochemistry
of the lavas from the Erzurum-Kars Plateau, Northeastern
Anatolia, Turkey, under revision.
-
Keskin,
M. , Magma generation by slab steepening
and breakoff beneath a subduction-accretion complex:
An alternative model for collision-related volcanism
in Eastern Anatolia, Turkey, Geophys. Res. Lett.,
30(24), 8046, doi:10.1029/ 2003GL018019,
2003.
-
Koronovskiy,
N.V. and L.I. Demina, A model for the collision
volcanism of the Caucasian segment of the Alpine
Fold Belt, Dokl. Akad. Nauk Rossi., 350,
519-522 (in Russian), 1996.
-
Kuno, H.,
Lateral variation of basalt magma types across continental
margins and island arcs, Bull. Volcanol.,
29, 195-222, 1966.
-
Maggi A.
and K. Priestley, Surface waveform tomography of
the Turkish-Iranian plateau, Geophys. J. Int.,
160 (3): 1068-1080, 2005.
-
Maggi, A.,
K. Priestley, and D. McKenzie, Seismic structure
of the Middle East, Eos Trans. AGU, 83(47),
Fall Meet. Suppl., Abstract S51B-1041, 2002.
-
Maheo, G.,
S. Guillot, J. Blichert-Toft, Y. Rolland, and A.
Pecher, A slab breakoff model for the Neogene thermal
evolution of South Karakorum and South Tibet,
Earth Planet. Sci. Lett, 195(1-2),
45-58, 2002.
-
Maury, R.C.,
S. Fourcade, C. Coulon, M. El Azzouzi, H. Bellon,
A. Coutelle, A. Oubadi, B. Semroud, M. Megartsi,
J. Cotton, Q. Belanteur, A. Louni-Hacini, A. Pique,
R. Capdevila, J. Hernandez, and J. P. Rehaulp, Post-collisional
Neogene magmatism of the Mediterranean Maghreb margin:
a consequence of slab breakoff, Comptes Rendus
de la Academie Des Sciences Serie II Fascicule A
Sciences de la Terre et des Planets, 331(3),
159-173, 2000.
-
McKenzie,
D. P., and M. J. Bickle, The volume and composition
of melt generated by extension of the lithosphere,
J. Pet., 29, 625-679, 1988.
-
McKenzie,
D.P. and R.K. O'Nions, Partial melt distribution
from inversion of rare earth element concentrations,
J. Pet., 32, 1021-1091,
1991.
-
McKenzie,
D.P., Active tectonics of the Mediterranean, Geophys.
J. R. Astr. Soc., 30(2), 109-185,
1972.
-
Mitchell,
J. and R. Westaway, Chronology of Neogene and Quaternary
uplift and magmatism in the Caucasus: constraints
from K - Ar dating of volcanism in Armenia, Tectonophysics,
304, 157-186, 1999.
-
National
Iranian Oil Company, Geological cross-sections north-west
Iran, Natl. Iranian Oil Co., Tehran, 1977.
-
Pearce, J.A.,
J.F. Bender, S.E. De Long, W.S.F. Kidd, P.J. Low,
Y. Guner, F. Saroglu, Y. Yilmaz, S. Moorbath, and
J.G. Mitchell, Genesis of collision volcanism in
Eastern Anatolia, Turkey, J. Volcanol. Geotherm.
Res., 44, 189-229, 1990.
-
Platt, J.P.
and P.C. England, Convective removal of lithosphere
beneath mountain belts: Thermal and mechanical consequences.
Am. J. Sci., 293, 307-336,
1993.
-
Reilinger,
R., S. McClusky, B. Oral., R. King, N. Toksoz, A.
Barka, I. Kinik, O. Lenk, and I. Sanli, Global positioning
system measurements of present-day crustal movements
in the Arabia-Africa-Eurasia plate collision zone,
J. Geophys. Res., 102, 9983-9999,
1997.
-
Rollinson,
H.R., Using geochemical data: evaluation, presentation,
interpretation, Longman Scientific & Technical,
John Wiley Sons, New York, 344 pp, 1993.
-
Rotstein,
Y., and A.L. Kafka, Seismotectonics of the southern
boundary of Anatolia, eastern Mediterranean region,
subduction, collision and arc jumping, J. Geophys.
Res., 87, 7694-7706, 1982.
-
Sandvol,
E., D. Seber, M. Barazangi, N. Turkelli, C. Gurbuz,
S. Kuleli, H. Karabulut, E. Zor, R. Gok, T. Bekler,
E. Arpat, and S. Bayraktutan, Eastern Turkey Seismic
Experiment, IRIS Newsletter, 2000(1),
2000.
-
Sandvol,
E., N. Turkelli, and M. Barazangi, The Eastern Turkey
Seismic Experiment: The study of a young continent-continent
collision, Geophys. Res. Lett., 30(24),
8038, doi:10.1029/2003GL018912, 2003a.
-
Sandvol,
E., N. Turkelli, E. Zor, R. Gok, T. Bekler, C. Gurbuz,
D. Seber, and M. Barazangi, Shear wave splitting
in a young continent-continent collision: An example
from Eastern Turkey, Geophys. Res. Lett.,
30(24), 8041, doi:10.1029/2003GL017390,
2003b.
-
Sen, P.A.,
A. Temel and A. Gourgaud, Petrogenetic modelling
of Quaternary post-collisional volcanism: a case
study of central and eastern Anatolia, Geol.
Mag., 141(1), 81–98, DOI: 10.1017/S0016756803008550,
2004.
-
Sengor,
A.M.C., The Turkish-Iranian High Plateau as a falcogenetic
structure, Workshop on the Tectonics of Eastern
Turkey and the Northern Anatolian plate, Erzurum,
Turkey, p. 28, 2002.
-
Sengor, A.M.C.,
and B. Natal’in, Turkic-type orogeny and its role
in the making of the continental crust, Annu.
Rev. Earth Planet. Sci., 24,
263-337, 1996.
-
Sengor, A.M.C.,
and W.S.F. Kidd, Post-collisional tectonics of the
Turkish-Iranian Plateau and a comparison with Tibet,
Tectonophysics, 55, 361-376,
1979.
-
Sengor, A.M.C.,
and Y. Yilmaz, Tethyan evolution of Turkey: a plate
tectonic approach, Tectonophysics, 75,
181-241, 1981.
-
Sengor, A.M.C.,
Elevation as indicator of mantle-plume activity,
Geol. Soc. Am., Sp. Pap., 352,
183-225, 2001.
-
Sengor, A.M.C.,
S. Ozeren, E. Zor, and T. Genc, East Anatolian high
plateau as a mantle-supported, N-S shortened domal
structure, Geophys. Res. Lett., 30(24),
8045, doi:10.1029/2003GL017858, 2003.
-
Shaw, D.M.,
Trace element fractionation during anatexis,
Geochim. Cosmochim. Acta, 34,
237-243, 1970.
-
Sun, S.S.
and W.F. McDonough, Chemical and isotopic systematics
of oceanic basalts: implications for mantle composition
and processes: In Magmatism in Ocean Basins
(A.D. Saunders & M.J. Norry eds.), Geol.
Soc. Lond. Spec. Pub., 42,
313-345, 1989.
-
Taylor, S.R.
and S.M. McLennan, The continental crust: its
composition and evolution, Geoscience Texts,
Blackwell Scientific Publications, London, 312 pp.,
1985.
-
Topuz, G.,
R. Altherr, A. Kalt, M. Satör, O. Werner, and
W.H. Schwarz, Aluminous granulites from the Pulur
complex, NE Turkey: a case of partial melting, efficient
melt extraction and crystallization, Lithos,
72, 183-207, 2004.
-
Tokel, S.,
Mechanism of crustal deformation and petrogenesis
of the Neogene volcanics in East Anatolia, special
publication of Turkiye Jeoloji Kurumu, Ketin
Symposium, Eds. T. Ercan and M.A. Caglayan,
121-130, 1985.
-
Turkelli,
N., E. Sandvol, E. Zor, R. Gok, T. Bekler,
A. Al-Lazki, H. Karabulut, S. Kuleli, T. Eken, C.
Gurbuz, S. Bayraktutan, D. Seber and M. Barazangi,
Seismogenic zones in Eastern Turkey, Geophys.
Res. Lett., 30(24), 8039, doi:10.1029/2003GL018023,
2003.
-
Turcotte,
D.L. Mechanism of crustal deformation, J. Geol.
Soc. Lond., 140, 701-724, 1983.
-
Williams,
H.M., S.P. Turner, J.A. Pearce, S.P. Kelley, and
N.B.W. Harris, Nature of the source regions for
post-collisional, potassic magmatism in Southern
and Northern Tibet from geochemical variations and
inverse trace element modeling, J. Pet.,
45(3), 555-607, 2004.
-
Wilson, M.,
Igneous petrogenesis: a global tectonic approach,
Unwin Hymen, London, 466 pp., 1989.
-
Yilmaz Y,
Y. Guner and F. Saroglu, Geology of the quaternary
volcanic centers of the east Anatolia, J. Volc.
Geotherm. Res., 85(1-4), 173-210,
1998.
-
Yilmaz, Y.,
O. Tuysuz, E. Yigitbas, S.C. Genc, and A.M.C. Sengor,
Geology and tectonic evolution of the Pontides,
in Regional and Petroleum geology of the Black
Sea and Surrounding Region, Ed. A.G. Robinson,
AAPG Memoir 68, 183-226,
1997.
-
Zor, E.,
Gurbuz, C., Turkelli, N., Sandvol, E., Seber, D.
and Barazangi, M., The crustal structure of the
East Anatolian Plateau from receiver functions,
Geophys. Res. Lett., 30(24),
8044, doi:10.1029/2003GL018192, 2003.
|
last
updated 20th June, 2005 |
|
|