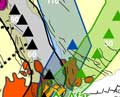 |
Hotspot paths below Arabia and the Horn of Africa: evidence and consequences |
S. Vicente de Gouveia1, J. Besse1, D. Frizon de Lamotte2, M. Greff-Lefftz1, M. Lescanne3, F. Gueydan4 and F. Leparmentier5
1Institut de Physique du Globe de Paris - Sorbonne Paris Cité, Université Paris Diderot, UMR CNRS 7154. 1 rue Jussieu, 75252 Paris 05, France, vicente@ipgp.fr ; besse@ipgp.fr ; greff@ipgp.fr
2Département Géosciences et Environnement, Université de Cergy-Pontoise, Cergy-Pontoise, France, Dominique.Frizon-de-Lamotte@u-cergy.fr
3Total EP, Pau, France, marc.lescanne@total.com
4Géosciences Montpellier, Université de Montpellier, CNRS UMR 5243, Montpellier, France, frederic.gueydan@umontpellier.fr
5Total EP, Paris La Défense, France, francois.leparmentier@total.com
This webpage is a summary of: Vicente de Gouveia, S., J. Besse, D. Frizon de Lamotte, M. Greff-Lefftz, M. Lescanne, F. Gueydan, and F. Leparmentier (2018), Evidence of hotspot paths below Arabia and the Horn of Africa and consequences on the Red Sea opening, Earth planet. Sci. Lett., 487, 210-220, doi:https://doi.org/10.1016/j.epsl.2018.01.030.
We investigate the history of three hotspots of the East African area widely described in the literature (Afar, East-Africa and Lake-Victoria) with the aim of retrieving their ancient traces, and linking their activity to the evolution of the Red Sea Rift system. Hotspot activity is episodic and irregular, as shown by the discontinuously spaced volcanic edifices on oceanic hotspot tracks, while the tracks themselves have a finite duration of tens to hundreds of millions of years. With the exception of continental flood basalts, knowledge of hotspot locations under the continental lithosphere remains poor, and their tracks are particularly difficult to know as the lithospheric thickness filters their surface effects. We defined from various lines of evidence present day hotspot coordinates. We then predicted past locations of our East African hotspots using a hotspot reference frame classically established on oceanic plates, which assumes that hotspots are approximately fixed and stable features over time in the mantle. Our preferred model is that of O’Neill et al. (2005) (for a summary, see de Gouveia et al., 2018).
Our starting point is to refine as much as possible the present location of:
- the Afar hotspot. Its present location is defined by the Stratoid Series at 4 Ma and by tomographic interpretation of a large low-velocity anomaly below the Afar triple junction (12°N, 42°E) (Montelli et al., 2006; Ritsema et al., 2011);
- the East-Africa hotspot. Its present position (6°N, 34°E) is indirectly established, using our plate model, from the mean position of the Ethiopian-Yemen traps;
- the Lake-Victoria hotspot. Its current position (3°S, 38°E) was determined from tomographic studies (Montelli et al., 2006; Ritsema et al., 2011; French & Romanowicz, 2015).
The computed hotspot trajectories are shown on Figure 1 from the present to 110 Ma. The track expected for the Afar hotspot started from northeast of Arabia and continued along the eastern boundary of the plate close to the present Zagros Front (Figure 1). At 50 Ma it changed direction and moved toward the present Afar triple junction, where it is currently located (Figure 1). The track expected for the East-Africa hotspot followed exactly the incipient plate boundary between Africa and Arabia, i.e., the present Red Sea, from 90 Ma to 40 Ma. At 40 Ma. It underlies the site of the present Afar triple junction, and continued to its present location (Figure 1). The Lake-Victoria hotspot came from the middle part of the present Red Sea and travelled southward to its current location (Figure 1).
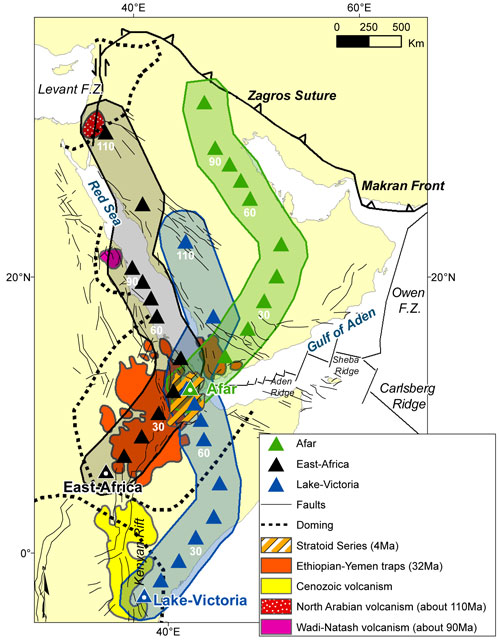
Figure 1: Geological context of the Arabian and African plates. The computed hotspot tracks were determined at 10 Ma time-intervals, according to O'Neill et al. (2005). Triangles with white dots – present day hotspot locations. Shaded area – minimum influence area of a hotspot with a diameter of 500 km. The Ethiopian-Yemen traps lie at the junction of the East-Africa and Lake-Victoria hotspot paths. Map presented as a Robinson projection.
We then searched for evidence of hotspot activity along the predicted paths. We particularly focused on:
- heat-flow anomalies;
- uplift due to plume buoyancy (Campbell, 2001);
- geochemical signatures of alkali basalt volcanism, indicating plume-like magmas (Campbell, 2001).
We supposed that any hotspot effects would be seen within a circle of 500 km diameter at the surface, keeping in mind that this area could be wider if the plume head had spread beneath the continental lithosphere. We also assumed that all hotspot effects occurred above the hotspot’s expected location. We considered the advection of plumes to be of minor significance.
Evidence for long-lived hotspot activity along the predicted hotspots tracks may be summarized (Figure 1):
Along the Lake-Victoria hotspot track:
- Cenozoic volcanism (Pik et al., 2006), with a mantle plume isotopic signature (Pik et al., 2006; Halldórsson et al., 2014),
- a long-wavelength dome (Ebinger et al., 1989; Koptev et al., 2015).
Along the Afar hotspot track:
- the 4 Ma Stratoid Series,
- a long-wavelength dome (Ebinger et al., 1989),
- a thermal anomaly (Figure 2). From a heat-flow study on the Arabian plate, using temperature data from 399 boreholes, complemented by 123 other heat-flow data (Morgan et al., 1985; Rolandone et al., 2013), we suggest that the high heat-flow observed on the eastern part of the Arabian plate corresponds to the Afar hotspot thermal memory.
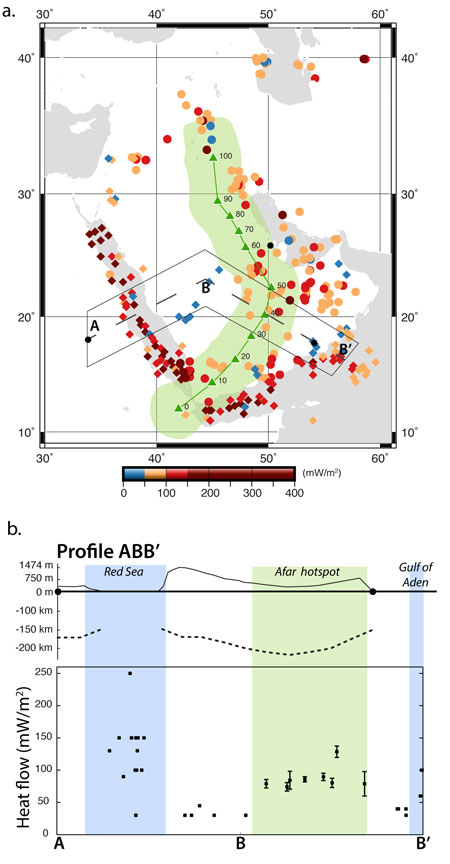
Figure 2: (a) Map of Arabian plate heat flow. Diamonds – heat-flow data from Morgan et al. (1985) and Rolandone et al. (2013). Dots – heat-flow data estimated from borehole data (TOTAL database). Green triangles symbolise the Afar hotspot location over time, according to the hotspot reference frame of O'Neill et al. (2005). The green shaded area indicates the minimum influence area within which hotspot consequences should be found. (b) Heat-flow profile along ABB' which crosses the Afar hotspot path at ~45 Ma. The blue shaded area indicates high heat-flow values. Only data with an error of less than 20 mW/m2 are plotted. The heat-flow map is presented as a cylindrical projection. Corresponding topographic profiles relative to the sea floor (solid line), and the thickness of the lithosphere (dashed line) were determined using Google Earth and the lithospheric model of Globig et al. (2016).
Along the East-Africa hotspot track:
- three episodes of volcanic activity, coupled with regional uplift, likely associated with hotspot activity, (1) at 100 Ma in the Levant region (Stein & Hofmann, 1992; Garfunkel, 1998; Gvirtzman et al., 1998; Ziegler, 2001); (2) at 90 Ma in the Wadi-Natash area (Ressetar et al., 1981; Crawford et al., 1984; Hubbard et al., 1987; Mohamed, 2001) and (3) between 40 Ma and 30 Ma, the Ethiopian-Yemen traps (Halldórsson et al., 2014),
- a large-scale dome related to a mantle plume extends into the Ethiopian-Yemen traps area (Ebinger et al., 1989).
East-Africa and Afar hotspot activity was found at least episodically back to 110 Ma and 90 Ma respectively. No activity was found for the Lake-Victoria hotspot prior to the Cenozoic. The results for the East-Africa hotspot track showed that the Afar hotspot cannot be the origin of the Ethiopian-Yemen traps at 30 Ma because it was located too far away. Conversely, our reconstruction shows that the East-Africa hotspot is the best candidate for explaining this volcanic activity. The existence of activity along the East-Africa track as far back as the Cretaceous suggests that this hotspot was episodically active long before the eruption of the Ethiopian-Yemen traps, and may indicate that continental flood basalts are related to an earlier-existing hotspot, and not necessarily to the birth of a new one. Finally, the coincidence between the Red Sea axis and the East-Africa hotspot path supports a causal relationship between thermal weakening along the hotspot path trajectory and the subsequent Red Sea rift system location.
References
-
Campbell, I.H., 2001, Identification of ancient mantle plumes: Geological Society of America Special Paper 352, 5-21
-
Crawford, W.A., Coulter, D.H., and Hubbard, H.B., 1984, The areal distribution, stratigraphy and major element chemistry of the Wadi Natash volcanic series, Eastern Desert, Egypt: Journal of African Earth Sciences, 2, 119-128.
-
Davaille, A., Stutzmann, E., Silveira, G., Besse, J., and Courtillot, V., 2005, Convective patterns under the Indo-Atlantic «box»: Earth and Planetary Science Letters, 239, 233-252.
-
Ebinger, C.J., Bechtel, T.D., Forsyth, D.W., and Bowin, C.O., 1989, Effective elastic plate thickness beneath the East African and Afar plateaus and dynamic compensation of the uplifts: Journal of Geophysical Research, 94, 2883-2901.
-
French, S.W., and Romanowicz, B., 2015, Broad plumes rooted at the base of the Earth's mantle beneath major hotspots. Nature, 525, 95-99.
-
Garfunkel, Z., 1998, Constrains on the origin and history of the Eastern Mediterranean basin: Tectonophysics, 298, 5-35.
-
Globig, J., Fernàndez, M., Torne, M., Vergés, J., Robert, A., and Faccenna, C., 2016, New insights into the crust and lithospheric mantle structure of Africa from elevation, geoid, and thermal analysis. Journal of Geophysical Research, 121, 5389-5424.
-
Gvirtzman, Z., Garfunkel, Z., and Gvirtzman, G., 1998, Birth and decay of an intracontinental magmatic swell: Early Cretaceous tectonics of southern Israel: Tectonics, 17, 441-457.
-
Halldórsson, S.A., Hilton, D.R., Scarsi, P., Abebe, T., and Hopp, J., 2014, A common mantle plume source beneath the entire East African Rift System revealed by coupled helium-neon systematics: Geophysical Research Letters, 41, 2304-2311.
-
Hubbard, H.B., Wood, L.F., and Rogers, J.J., 1987, Possible hydration anomaly in the upper mantle prior to Red Sea rifting: Evidence from petrologic modeling of the Wadi Natash alkali basalt sequence of eastern Egypt: Geological Society of America Bulletin, 98, 92-98.
-
Koptev, A., Calais, E., Burov, E., Leroy, S., and Gerya, T., 2015, Dual continental rift systems generated by plume-lithosphere interaction. Nature Geoscience, 8, 388-392.
-
Mohamed, F.H., 2001, The Natash alkaline volcanic field, Egypt: geochemical and mineralogical inferences on the evolution of a basalt to rhyolite eruptive suite. Journal of Volcanology and Geothermal Research, 105, 291-322.
-
Montelli, R., Nolet, G., Dahlen, F.A., and Masters, G., 2006, A catalogue of deep mantle plumes: New results from finite-frequency tomography: Geochemistry, Geophysics, Geosystems, 7, 11.
-
Morgan, P., Boulos, F.K., Hennin, S.F., El-Sherif, A.A., El-Sayed, A.A., Basta, N.Z., and Melek, Y.S., 1985, Heat flow in eastern Egypt: the thermal signature of a continental breakup: Journal of Geodynamics, 4, 107-131.
-
O'Neill, C., Müller, D., and Steinberger, B., 2005, On the uncertainties in hot spot reconstructions and the significance of moving hot spot reference frames: Geochemistry Geophysics Geosystems, 6, Q04003.
-
Pik, R., Marty, B., and Hilton, D.R., 2006, How many mantle plumes in Africa? The geochemical point of view: Chemical Geology, 226, 100-114.
-
Ressetar, R., Nairn, A.E.M., and Monrad, J.R., 1981, Two phases of Cretaceous-Tertiary magmatism in the eastern desert of Egypt: Paleomagnetic, chemical and K-Ar evidence: Tectonophysics, 73, 169-193.
-
Ritsema, J., Deuss, A., Van Heijst, H. J., and Woodhouse, J. H., 2011, S40RTS: a degree-40 shear-velocity model for the mantle from new Rayleigh wave dispersion, teleseismic traveltime and normal-mode splitting function measurements. Geophysical Journal International, 184, 1223-1236.
-
Rolandone, F., Lucazeau, F., Leroy, S., Mareschal, J.C., Jorand, R., Goutorbe, B., and Bouquerel, H., 2013, New heat flow measurements in Oman and the thermal state of the Arabian Shield and Platform: Tectonophysics, 589, 77-89.
-
Stein, M., and Hofmann, A.W., 1992, Fossil plume head beneath the Arabian lithosphere?: Earth and Planetary Science Letters, 114, 193-209.
-
Ziegler, M.A., 2001, Late Permian to Holocene paleofacies evolution of the Arabian Plate and its hydrocarbon occurrences, GeoArabia, 6, 445-504.
last updated 12th April, 2018 |