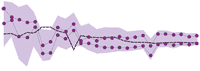 |
Upper & Lower Crustal Recycling
in the Source of CAMP Basaltic Dykes,
SE North America
|
Sara Callegaro1, Hervé Bertrand2, Andrea Marzoli1, Giuliano Bellieni1, Laurie Reisberg3, Massimo Chiaradia4, Christine Meyzen1, Renaud Merle5
1Dipartimento di Geoscienze, Università degli Studi di Padova, via Gradenigo 6, 35131 Padova, Italy, sara.callegaro@unipd.it ; andrea.marzoli@unipd.it ; giuliano.bellieni@unipd.it ; christine.meyzen@unipd.it
2Laboratoire de Géologie de Lyon, UMR-CNRS5276, Université Lyon1 and Ecole Normale Supérieure de Lyon, 46, Allée d’Italie, 69364 Lyon, France, Herve.Bertrand@ens-lyon.fr
3Centre de Recherches Pétrographiques et Géochimiques (CRPG) - CNRS UMR 7358, Université de Lorraine, BP 20, 54501 Vandoeuvre-les-Nancy Cedex, France, reisberg@crpg.cnrs-nancy.fr
4Section des Sciences de la Terre, Université de Genève, 13 rue des Maraîchers, 12011 Genève, Switzerland, Massimo.Chiaradia@unige.ch
5Department of Applied Geology, Curtin University, GPO Box 25 U1987, Perth, WA 6845, Australia, R.Merle@curtin.edu.au
This webpage is a summary of: Callegaro, Sara, Andrea Marzoli, Hervé Bertrand, Massimo Chiaradia, Laurie Reisberg, Christine Meyzen, Giuliano Bellieni, Robert E. Weems, Renaud Merle, Upper and lower crust recycling in the source of CAMP basaltic dykes from southeastern North America, Earth Planet. Sci. Lett., 376, 186-199, 2013.
The densest dyke swarm of the Central Atlantic Magmatic Province (CAMP; Figure 1) occurs in southeastern North America (SENA) and was intruded between 202 and 195 Ma during Pangea break-up. New combined geochemical data (major and trace elements, Sr-Nd-Pb-Os isotopes) constrain the mantle source of these magmatic bodies and their evolution path.
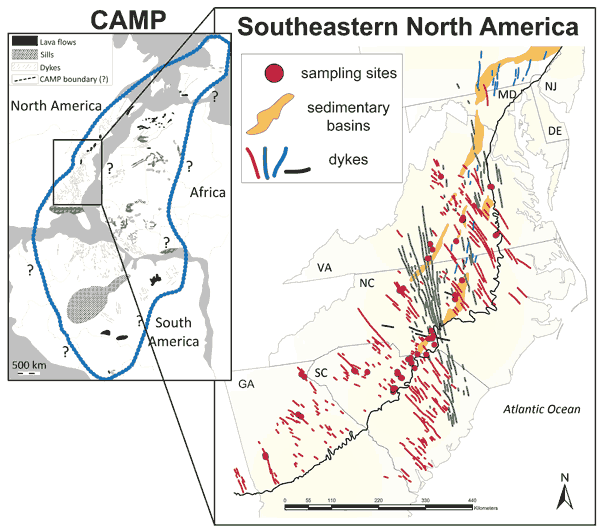
Figure 1: Sampling sites on an overview map of the southeastern North American diabase dyke swarm. Inset: CAMP outcrops are represented on a Late Triassic geographic reconstruction.
We sampled 76 tholeiites (basalts and basaltic andesites). All SENA rocks are moderately enriched in incompatible elements (IE). Unlike E-MORBs and within-plate oceanic basalts in general, SENA dykes are depleted in Nb, Ta and Ti and enriched in Rb, Ba and Pb, therefore displaying a clear continental signature as observed for most CAMP basalts (Figure 2).
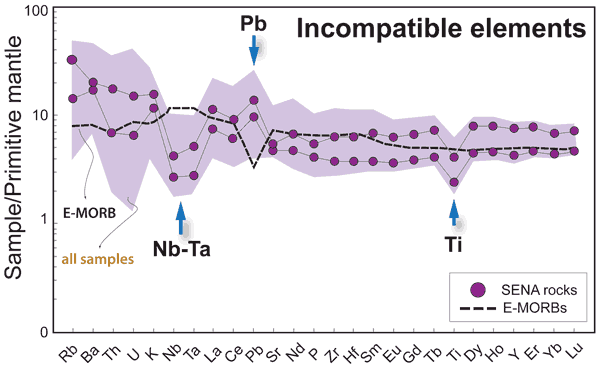
Figure 2: Primitive mantle-normalized trace element contents of analyzed SENA tholeiites (lilac field). The compositions of two representative and rather primitive (MgO-rich) samples are detailed. For comparison, E-MORB average composition is shown (black dashed line). Pb peak and Nb-Ta and Ti troughs are highlighted.
While Sr-Nd isotopic compositions for SENA rocks (87Sr/86Sr200Ma 0.70438-0.70880 and 143Nd/144Nd200Ma 0.51251-0.51204) fall within the low-Ti CAMP field, Pb-Pb isotopes (206Pb/204Pb200Ma 17.46-18.85, 207Pb/204Pb200Ma 15.54-15.66, 208Pb/204Pb200Ma 37.47-38.76) are peculiar to this area of the CAMP and cover a considerable range of compositions (Figure 3).
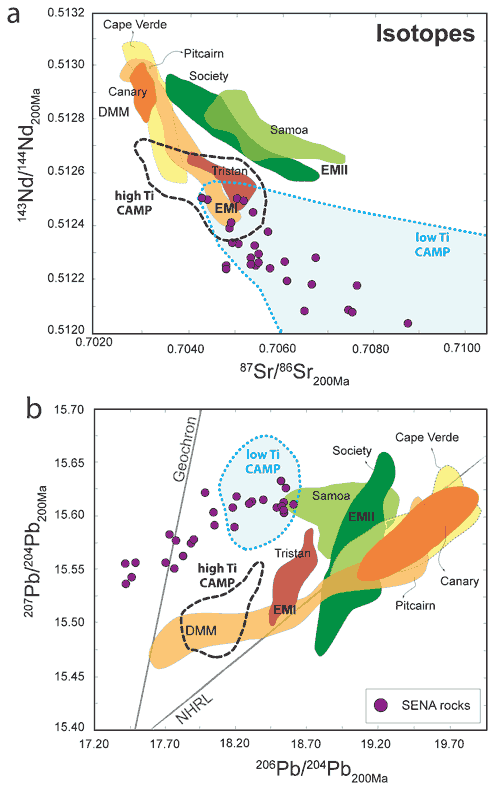
Figure 3: (a)143Nd/144Nd vs. 87Sr/86Sr and (b) 207Pb/204Pb vs. 206Pb/204Pb initial compositions for SENA dykes are plotted along with several OIB compositional fields representative of C (Canary, Cape Verde), EMI (Tristan da Cunha, Pitcairn) or EMII-type (Society, Samoa) mantle components (Willbold & Stracke, 2010; Gibson, 2005; Hofmann, 2003; Zindler & Hart, 1986). Low-Ti and high-Ti fields are also outlined for other CAMP tholeiites (data from Merle et al., 2011).
Given the low Os isotopic compositions (187Os/188Os200Ma 0.127-0.144; Figure 4) and the lack of correlation of Os isotopes with other geochemical markers, crustal contamination during the evolution of SENA dykes must have been limited (less than 10 wt%). ECAFC (Energy Constrained Assimilation and Fractional Crystallization; Spera & Bohrson, 2001) calculations hinge on Os isotopes. Starting from the relatively low 187Os/188Os200Ma (0.1354; [Os] 276 ppt), the highest observed 187Os/188Os200Ma values (e.g., 0.144, [Os] 213 ppt) are reached with a very limited amount of assimilation of either upper (ca. 5%) or lower (ca. 7-9%) crust. Even lower amounts of assimilation would be permitted if higher 187Os/188Os, more typical of average continental crust, were assumed for the contaminant. These limited maximum amounts of crustal assimilation would allow for only very minor shifts of Sr-Nd-Pb isotopic ratios, unless extreme isotopic compositions of SENA crustal rocks are considered. Thus, the isotopic variability is interpreted to reside primarily within the mantle source.
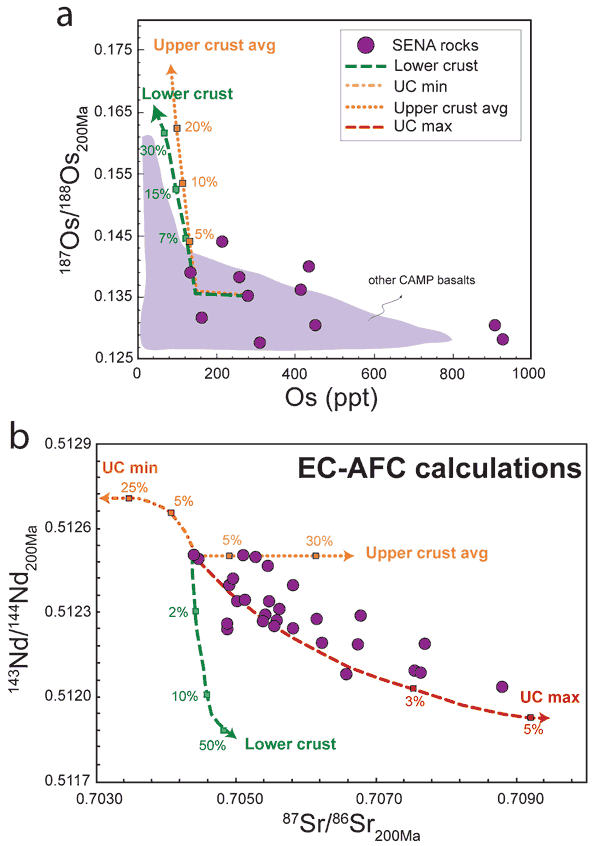
Figure 4: Contamination paths for the upper and the lower crust are calculated with EC-AFC modeling and plotted in a 187Os/188Os200Ma vs. [Os] and 143Nd/144Nd200Ma vs. 87Sr/86Sr200Ma. Three compositions were hypothesized for the upper crust, namely a maximum (with more ‘evolved’ composition), average and minimum values.
Typical continental signatures in trace elements therefore require another means of conveying a continental flavor to these magmas. That is hypothesized here to be the shallow recycling within the upper mantle of subducted lower and upper crustal materials. Pseudo-ternary mixing models (Figure 5) show that a maximum of 10% recycled crust is enough to explain both the trace element patterns and the isotopic heterogeneity.
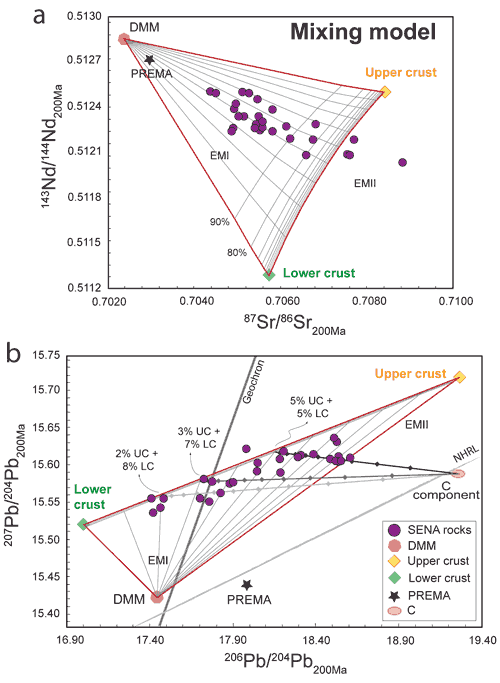
Figure 5: Pseudo-binary mixing calculations between DMM, lower crust and upper crust. The DMM pole is calculated from a mid-Atlantic ridge MORB compilation, because of geographic proximity. Composition of the upper crust reflects that of local Meguma upper crust. Lower crust isotopic values are selected to be within the range of global lower crustal xenolith data (compiled by Meyzen et al., 2005). Mixing lines with a C-flavoured (Hanan & Graham, 1996) mantle plume are calculated starting from mixed lower- and upper-crust recycled components.
The thermal contribution of a mantle plume cannot be ruled out because of the relatively high mantle potential temperatures (1430°-1480°C) calculated for highly forsteritic (Fo>87) olivines from high-MgO (>12 wt%) SENA basalts. Mantle potential temperatures were calculated for dry conditions on the same rocks with the geothermometer of Putirka (2008), yielding values of 1487–1466°C, and with PRIMELT2 (Herzberg & Asimow, 2008), yielding values of 1440-1432°C.
Although we cannot exclude a thermal contribution from a mantle plume, our geochemical results show that C- or EM-flavored plume material does not exist in the source of SENA tholeiites. The only possible composition that could be argued for a plume source for these rocks is that of the non-chondritic deep mantle reservoir PREMA (Jackson & Carlson, 2011), similar to the prevalent mantle (PREMA component) of Zindler & Hart (1986), enriched by 5-20% recycled crustal material. However, in agreement with Jackson & Carlson (2011), we prefer to exclude the CAMP from the LIPs originating from the non-chondritic deep reservoir. In our opinion, an upper mantle source enriched with recycled lower- and upper-crustal material is the most likely source for the SENA CAMP.
References
-
Gibson, S.A., Thompson, R.N., Day, J.A., Humphris, S.E., Dickin, A.P., 2005. Melt-generation processes associated with the Tristan mantle plume: Constraints on the origin of EM-1. Earth Planet. Sc. Lett. 237, 744– 767.
-
Hanan, B.B., Graham, D.W., 1996. Lead and helium isotope evidence from oceanic basalts for common deep source of mantle plumes. Science 272, 991–995.
-
Herzberg, C., Asimow, P.D., 2008. Petrology of some oceanic island basalts: PRIMELT2.XLS software for primary magma calculation. Geochemistry, Geophysics, Geosystems 9, doi:10.1029/2008GCQ09001.
-
Hofmann, A.W., 2003. Sampling Mantle Heterogeneity through Oceanic Basalts: Isotopes and Trace Elements. In: Holland. H.D., Turekian, K.K., (Eds.), Treatise on geochemistry, The Mantle and Core. Oxford, UK, pp. 61-101.
-
Jackson, M.G., Carlson, R.W., 2011. An ancient recipe for flood-basalt genesis. Nature 476, 316-319.
-
Marzoli, A., Jourdan, F., Puffer, J.H., Cuppone, T., Tanner, L.H., Weems, R.E., Bertrand, H., Cirilli, S., Bellieni, G., De Min, A., 2011. Timing and duration of the Central Atlantic magmatic province in the Newark and Culpeper basins, eastern U.S.A. Lithos 122, 175-188.
-
Merle, R., Marzoli, A., Bertrand, H., Reisberg, L., Verati, C., Zimmermann, C., Chiaradia, M., Bellieni, G., Ernesto, M., 2011a. 40Ar/39Ar ages and Sr–Nd–Pb–Os geochemistry of CAMP tholeiites from Western Maranhão basin (NE Brazil). Lithos 122, 137-151.
-
Meyzen, C.M., Ludden, J.N., Humler, E., Luais, B., Toplis, M.J., Mével, C., Storey, M., 2005. New insights into the origin and distribution of the DUPAL isotope anomaly in the Indian Ocean mantle from MORB of the Southwest Indian Ridge. Geochem., Geophys., Geosyst. 6. Q11K11, doi:10.1029/2005GC000979.
-
Putirka, K., 2008. Thermometers and Barometers for Volcanic Systems. In: Putirka, K., and Tepley, F., eds., Minerals, Inclusions and Volcanic Processes. Reviews in Mineralogy and Geochemistry, Mineralogical Society of America 69, 61-120.
-
Spera, F.J., Bohrson, W.A., 2001. Energy-Constrained Open-System Magmatic Processes I: General Model and Energy-Constrained Assimilation and Fractional Crystallization (EC-AFC) Formulation. Journal of Petrology 42, 999-1018.
-
Willbold, M., Stracke, A., 2010. Formation of enriched mantle components by recycling of upper and lower continental crust. Chem. Geol. 276, 188–197.
-
Zindler, A., Hart, S.R., 1986. Chemical geodynamics. Ann. Rev. Earth Planet. Sci. 14, 493–571.
last updated 27th September, 2013 |