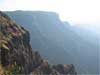 |
Plume-related
regional pre-volcanic uplift in the Deccan Traps:
Absence of evidence, evidence of absence |
Hetu
C. Sheth
Department
of Earth Sciences, Indian Institute of Technology (IIT)
Bombay, Powai, Bombay (Mumbai) 400 076 India.
hcsheth@iitb.ac.in
or hcsheth1@yahoo.co.in
This webpage is a
summary of a
paper by the same title, accepted for the forthcoming
2007 GSA “P4” Special Paper edited
by G. R. Foulger and D. M. Jurdy. See this paper for
fuller details and more references.
Click here to
download a PDF version of this webpage
Abstract
The mantle plume model
predicts regional, domal, kilometer-scale lithospheric
uplift 5-20 million years before the onset of flood
basalt volcanism. Field evidence for such uplift would
comprise progressively shallowing sedimentary sequences,
or (with differential uplift along faults) widespread
conglomerates derived from the basement rocks and underlying
the lavas. Local uplifts and subsidences cannot be used
to invoke or rule out plume-caused uplift. Over large
areas, the base of the late Cretaceous Deccan flood
basalts is in the subsurface. Basalt-basement contacts
are observed along the periphery of the province, and
in central India (the Satpura and Vindhya Ranges), where
substantial post-Deccan uplift is evident. Here, horizontal
Deccan basalt flows directly overlie extensive low-relief
planation surfaces cut on various older rocks (Archean
through Mesozoic). Locally, thin, patchy late Cretaceous
clays and limestones (the Lameta Formation) separate
the basalts and basement, but some Lameta sediments
were derived from already erupted Deccan basalt flows
in nearby areas. Thus, the eruption of the earliest
Deccan basalt lava flows onto extensive, flat planation
surfaces developed on varied bedrock, and the near-total
absence of basement-derived conglomerates at the base
of the lava pile throughout the province are evidence
against pre-volcanic lithospheric uplift (both regional
and local), and thereby the plume head model. There
has been major (>1 km) post-Deccan, Neogene uplift
of the Indian peninsula and the Sahyadri (Western Ghats)
Range which runs along the entire western Indian rifted
margin, well beyond the Deccan basalt cover. This uplift
has raised the regional, late Cretaceous planation surface
developed on the Deccan lava pile to a high elevation.
This uplift cannot reflect Deccan-related magmatic underplating,
but is partly denudational, is aided by a compressive
stress regime throughout India since the India-Asia
collision, and possibly also related to active eastward
flow of the sub-lithospheric mantle. The easterly drainage
of the Indian peninsula, famously speculated to be dome-flank
drainage caused by a plume head, predates the uplift.
Field evidence from the Deccan and India is in conflict
with a model of plume-caused regional uplift a few million
years prior to the onset of volcanism.
…one true inference
invariably suggests others. (Sherlock Holmes, in “Silver
Blaze”)
Introduction:
Flood basalts, plume heads, and lithospheric uplift
The deep mantle plume
model (Richards et al., 1989; Campbell
& Griffiths, 1990) postulates that thermal
plumes rise buoyantly from the core-mantle boundary
and develop large bulbous “heads” by entrainment
surrounding mantle. The heads remain connected to the
source region by narrow “tails”. A new (“starting”)
plume head, 1000 km in diameter, flattens to a disc
twice as wide after impinging on the base of the lithosphere,
and lifts the lithosphere up over a broad area ~1000
km across before flood basalt volcanism begins. Significant
domal uplift (1-4 km depending on parameters such as
plume temperature) is predicted 10-20 million years
(m.y.) before flood volcanism, when the plume head is
still well below the lithosphere (Campbell &
Griffiths, 1990; Farnetani & Richards,
1994). The uplift is followed by subsidence as the plume
head begins to melt extensively and the magmas are drained.
Geological evidence for
pre-volcanic uplift can take the form of pre-eruption
sedimentary sequences that reflect progressive basin
shallowing before volcanism (e.g., Rainbird &
Ernst, 2001). Alternatively, with differential
tectonic uplift along faults, rapid erosion of the basement
rocks would produce beds of conglomerate or coarse clastics
below the first lavas. If the uplift were regional,
such sediments should have a regional distribution.
It has been claimed that geological and geomorphological
evidence from flood basalt provinces of the world fullfills
the predictions and patterns of the above model (Cox,
1989; Campbell,
2005). Here I examine the evidence from the Deccan
flood basalt province and India, showing that this is
not true there.
Deccan
and Indian geology
The late Cretaceous (~65
Ma) Deccan province was associated with India-Seychelles
break-up (Figure 1) [Ed: see also other
Deccan pages]. Earlier, at ~88 Ma, Greater India
(India plus the Seychelles) broke off from Madagascar,
an event which was associated with flood basalt volcanism
on Madagascar and relatively minor volcanic-intrusive
activity in India (Storey et al., 1995; Pande
et al.,
2001). A description of the main features of Indian
and Deccan geology can be found in Sheth
(2005a).
Figure 1. Map showing
the structural framework of the Indian subcontinent,
including Precambrian cratons (boundaries approximate),
structural trends defined by Precambrian fold belts
(primarily Proterozoic), the rift zones (primarily Phanerozoic),
and the present outcrop area of the Deccan flood basalt
province (green). Modified after Sheth (2005a,b).
The inset figures show the breakup of Greater India
from Madagascar at ca. 88 Ma (Storey et al., 1995; Pande
et al., 2001), and the breakup of the Seychelles
microcontinent (located at the northern tip of the Mascarene
Plateau, black) from India at ca. 65 Ma (Norton &
Sclater, 1979).
Figure 2 shows the main
rock formations that make up the Indian shield. Over
a large part of the province, the contact of the Deccan
lavas with the pre-volcanic basement is not exposed.
The Deccan lavas overlie a complex Archaean and Proterozoic
basement along the the southern and southeastern periphery
of the province. In the northern and northeastern parts
of the province, i.e., central India, they overlie diverse
geological formations: the Vindhyan sedimentary basin
(mid-late Proterozoic), the Gondwana sedimentary basin
(Carboniferous to Jurassic-early Cretaceous), late Cretaceous
Bagh and Lameta sediments, and Archaean and early Proterozoic
crystalline rocks (granites, gneisses and metasediments).
Figure 2. Sketch-map
of western and central India showing its main geological
features, main geographic-physiographic features (italicized),
and the outcrop of the Deccan flood basalts (green).
The Bagh and Lameta sediments and the Bijawar metamorphics
are too small to show at the scale of the map, but the
locations of the Vindhyan and Satpura Gondwana Basins
are indicated. Also shown are localities mentioned in
the text. Modified after Sheth
(2005a)
Many major Indian rivers
originate in the Sahyadri Range (Western Ghats), and
instead of draining into the Arabian Sea a few tens
of kilometers to the west, they flow for hundreds of
kilometers eastward to the Bay of Bengal (Figure 3). Cox
(1989) speculated that this was a consequence of regional
domal uplift caused by a Deccan plume head. He presented
drainage maps from the Karoo (South Africa) and the
Parana flood basalt provinces, and considered each example
as the preserved half of an originally complete dome-flank
pattern produced by a plume head. Summerfield
(1990) pointed out that the Cox (1989) model
ignored aspects such as the Cenozoic drainage development
in Africa. The model also ignores and is incompatible
with well-known geological facts from India, and no
quantitative data (such as apatite fission-track ages)
have ever been offered in support of the postulated
plume-caused doming.
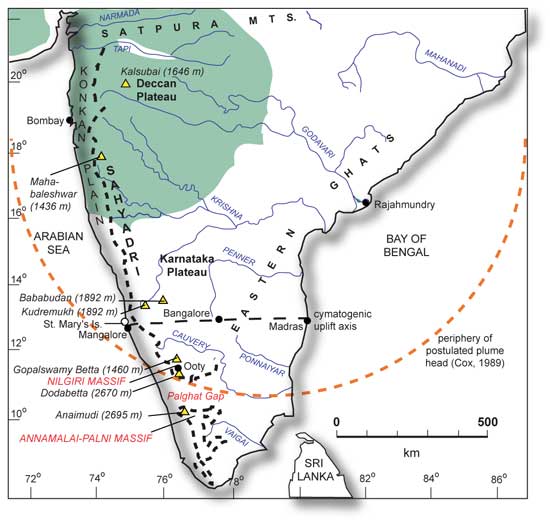
Figure 3. The main
elements of the physiography of the Indian peninsula,
based on Ollier & Powar (1985) and Sheth
(2005a). Note the pronounced easterly drainage.
The outcrop area of the Deccan flood basalts is shown
in green. The Sahyadri (Western Ghats) escarpment is
shown by the heavy broken line, and some major summits
of the Ghats are indicated, as are some localities cited
in the text. The circumference of the plume head proposed
by Cox (1989) and the axis of cymatogenic uplift at
13°S latitude proposed by Subrahmanya (1994) are
also shown. The St. Mary’s Islands rhyodacite
lavas (~85 Ma) along the west coast are older than the
Deccan Traps, and related to the India-Madagascar break-up
event (Pande
et al., 2001).
The Sahyadri Range constitutes
a NNW-SSE-trending and 1500-km-long “Great Escarpment”
along the western Indian rifted margin (Gunnell
& Radhakrishna, 2001) and has been retreating
eastward due to erosion (Widdowson, 1997a).
There is only one break in the escarpment, the controversial
“Palghat Gap” (Figure 3) which has a maximum
elevation of 300 m above sea level, an average width
of only 13 km, and which is entirely rock-floored without
alluvium cover (Gunnell & Radhakrishna,
2001). On its sides, the Ghats reach great heights of
>2.5 km above MSL. The Nilgiri massif to the north
of the Gap, and the Palni-Kodaikanal massif to the south,
are made up of Precambrian hypersthene-bearing granites
and granulites (“charnockites”). These mountains
are the highest in peninsular India, and among the highest
in shield areas anywhere (Gunnell & Louchet,
2000). Summits of the Western Ghats and the Karnataka
plateau, between the Deccan basalts and the southern
charnockite massifs, also approach 2 km in height (e.g.,
Bababudan, Figure 4) and are built of Precambrian quartzites
and gneisses. The summit of the Deccan basalts, Kalsubai,
stands at 1646 m (Figure 3).
Figure 4a,b,c,d. Views
of the Sahyadri (Western Ghats) escarpment at Mahabaleshwar,
showing the 1200-m-thick, horizontally disposed Deccan
flood basalt lava pile. The top of the lava pile is
a heavily lateritized late Cretaceous planation surface.
(d) The extensive, lateritized, heavily forested Mahabaleshwar
plateau surface. Photos by Hetu Sheth, December 2005.
The great heights of the
charnockite massifs may reflect both the original topography
and the greater weathering resistance of charnockite
than basalt (Gunnell & Louchet, 2000).
Most importantly, the entire youthful Sahyadri is
the precipitous western edge of an uplifted plateau
that has been tilted eastward, and the plateau surface
has an aged character – it is an ancient flat
land surface rejuvenated relatively recently (Neogene)
by major tectonic uplift (Radhakrishna, 1952, 1993;
Vaidyanadhan, 1977), the uplift continuing during the
Quaternary (e.g., Powar, 1993; Valdiya, 2001).
Mahabaleshwar sits atop a spectacular, ~1200-m-thick
exposed Deccan basalt sequence (Figure 4a,b,c), and
the top of the Mahabaleshwar plateau represents a regional,
low-relief, late Cretaceous palaeosurface or peneplain
developed on the uppermost basalts after the cessation
of the eruptions, represented by 25-50 m thick laterite
(Widdowson, 1997a). In southern India, laterites
or bauxites cap the high-elevation summits built of
Precambrian rocks. Sahyadri uplift, extending well beyond
the Deccan lava cover, is evidently not related to Deccan
volcanism, but is more appropriately considered “rift-shoulder
uplift”. Along with the Sahyadri, the Konkan Plain
to the west of it has also been rising during the Tertiary
(e.g., Powar, 1993).
Ollier & Powar
(1985) observed that the drainage pattern of peninsular
India is dendritic over both Deccan lavas and the older
basement, and suggested that the drainage developed
subsequent to the eruption of the Deccan lavas. The
newly formed lava field could have had a regional eastward
slope. However, they also noted that the drainage is
antecedent (prior to) the uplift of the Sahyadri Range.
Widdowson & Cox (1996) showed that around
Mahabaleshwar, the easterly drainage cuts through a
N-S-aligned, south-plunging anticlinal structure identified
from regional dips of the basalt lavas and the laterite
cap. The anticlinal structure developed subsequent to
the drainage and had no effect on the drainage lines.
The eastward-draining Godavari-Krishna River system
also was already in existence by the time the Deccan
lavas were in eruption, if the thin basalt flow sequence
at Rajahmundry on the southeastern coast of India (Figures
2,3) represents intra-canyon flows (akin to many in
the Columbia River basalt province) (Baksi et al.,
1994).
The easterly drainage
of the peninsula is also antecedent to the uplift of
the Eastern Ghats, according to Ollier & Powar
(1985). The Eastern Ghats, made up primarily of Precambrian
metamorphic rocks, form disconnected hill ranges in
eastern India and are thus unlike the Western Ghats,
except in southeastern India, and major rivers such
as the Cauvery are antecedent to them and have cut gorges
(Ollier & Powar, 1985). Even the easterly
drainage in southern India is an oversimplification,
as there is a distinct E-W axis of cymatogenic uplift
(crustal arching) at 13°N latitude, to the north
of which the rivers flow NE, and to the south of which
they flow SE (Subrahmanya, 1994; Figure 3).
Mangalore on the west coast and Madras on the east coast
are both located on this axis, and both are actively
rising relative to the sea (Bendick & Bilham,
1999). The uplift of the Sahyadri (Western Ghats), and
the attendant uplift of the Konkan Plain to the west
of it, are definitely post-Deccan, and offshore sedimentary
evidence and apatite fission-track data are consistent
with this (Gunnell & Gallagher, 2001; Gunnell
et al.,
2003). In summary, the easterly drainage of
the Indian peninsula is older than the uplift of the
Ghats (both the Western and the Eastern), and the uplift
of the Ghats is much younger than Deccan volcanism (Sheth,
2007). The proposition of Cox (1989),
that the easterly drainage is a result of a plume-generated
lithospheric dome, is therefore baseless, but has never
been examined, far less questioned, by plume enthusiasts
and regional experts influenced by them.
Deccan
basalt and basement contacts
Over most of the Deccan
province the lava-basement contact is in the subsurface.
There is a huge thickness (~1700 m exposed) of Deccan
basalts in the Sahyadri region, and ~500 m in the subsurface,
as identified from seismics, which also suggest two
linear, kilometer-thick, Mesozoic sedimentary basins
in the subsurface below the Deccan basalts in the Narmada-Tapi
region (Kaila, 1988; Sridhar & Tewari,
2001). North of the Narmada River, Deccan basalts overlie
the mid-late Proterozoic Vindhyan sediments, or older
crystalline basement directly. To the south of the river,
in the Satpura Range, Deccan lavas overlie the Gondwana
sedimentary basin (Carboniferous to Jurassic-early Cretaceous),
or the Precambrian basement. The Gondwana Basin is particularly
well-exposed in the Pachmarhi area (Figure 2) due to
substantial post-Deccan uplift along basin boundary
faults.
Two other late Cretaceous
sedimentary formations – the Bagh and Lameta –
underlie the Deccan basalts locally in central India
(e.g., Mohabey, 1996; Tandon,
2002). Both separate the Deccan basalts from the
Vindhyans, the Gondwanas, or the Archaean-Proterozoic
crystalline basement. The Bagh Formation consists of
sandstones and limestones formed during a marine transgression
in the western Narmada valley (Sheth
2005a), where one would expect maximum uplift from
a putative Deccan plume head at this exact time. The
fluvial and lacustrine Lameta Formation consists of
thin (~20 m) limestones and clays. The Lametas overlie
the Gondwana sediments or Precambrian rocks, and are
themselves overlain by the Deccan basalt flows in some
sections, based on which they were considered older
than the basalts. More recent work indicates that Lameta
clays around Jabalpur (Figure 2) were derived from the
Deccan basalts themselves, already erupting in nearby
areas (Shrivastava
& Ahmad,
2005). Pre-eruption uplift is recorded in the Lameta
sediments of the Dongargaon Basin of the Nagpur region
(Figure 2), where Tandon
(2002) recorded a clear “shallowing up”
trend from shallow lake deposits to a palaeosol before
the terrain was buried by the first Deccan basalt flow.
He invoked, however, pre-volcanic surface uplift of
the area of meters only and possibly also mock aridity
(Harris & van Couvering, 1995).
Thus there were both local
uplifts and subsidences just before volcanism in parts
of central India, but such cannot be used to support
or refute the plume model and are easily related to
the filling and emptying of magma chambers, emplacement
of intrusions, and faulting. At Rajpipla (Figure 2),
a basalt- and basement-derived conglomerate bed that
unconformably overlies tilted late Cretaceous sediments
and is overlain by the Deccan basalts (Widdowson,
2005) suggests that uplift and/or tilting and erosion
of the late Cretaceous sediments occurred in quick succession
just before volcanism. One or more basalt flows were
then erupted nearby, and continuing uplift and erosion
produced the conglomerate, which was in turn covered
by subsequent lavas. This is local uplift as can be
produced by moderately large intrusions, or faulting,
both well recognized in this area (Ray et al.,
2003). This conglomerate appears similar to conglomerates
interlayered with Palaeogene basalt flows on the Isle
of Skye, Scotland. Here, a basaltic lava flow of the
Talisker Bay Group is underlain by a conglomerate of
the Preshal Beg Conglomerate Formation (Figure 5). The
conglomerate is a very poorly sorted and chaotic deposit,
and was derived from the nearby lavas by local uplift
and probably slope instabilities (Williamson &
Bell, 1994).
(a) |
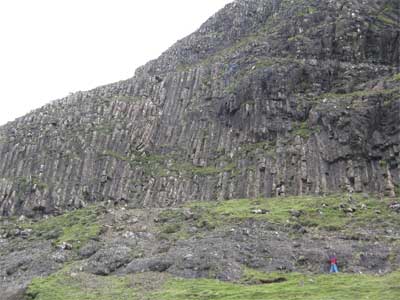 |
(b) |
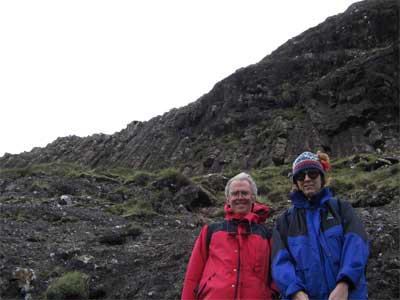 |
Figure 5. (a) Chaotic,
completely unsorted Preshal Beg Formation conglomerate
under a spectacularly columnar-jointed olivine tholeiite
lava flow of the Talisker Bay Group, Isle of Skye, Scotland.
Person near lower right corner of photo provides a scale.
(b) Closer view of the chaotic conglomerate and the
columnar lava flow above, with more recognizable folk
for scale. Photos by Hetu Sheth, September 2005.
What, then, is the evidence
in central India for or against regional pre-eruption
uplift? Planation surfaces are a key evidence bearing
on this issue.
Planation
surfaces: key evidence
Planation surfaces are
regional, flat or nearly flat surfaces produced by advanced
erosion (usually fluvial) of any earlier topography
to the existing base level of erosion, in most cases
the sea level. (Large, high inland plateaus like Tibet
have their own base level, and a large river may act
as a sub-regional base level.) Planation surfaces are
also called erosion surfaces or peneplains. Palaeosurfaces
(Widdowson, 1997b) constitute a broader category
of ancient land surfaces that can be exogenic (erosion/weathering)
or endogenic (e.g., lava plains). A planation
surface is therefore a kind of exogenic palaeosurface.
On a peneplain, relief
is minor to absent, and erosion is therefore negligible,
but deep in situ weathering and lateritization and ferricretization
are typical (Summerfield, 1991). A planation
surface indicates long-term tectonic stability and the
absence of uplift and erosion. If a low-lying peneplain
is uplifted to form a plateau, the dissection of the
plateau by further erosion works towards forming a younger
planation surface at a lower level, broken only by some
surviving remnants (“monadnocks”) of the
higher surface (Figure 6a). Also, because no process
can produce a flat erosional surface out of jagged terrain
at high elevation, a geologically young planation surface
at high elevation today suggests rapid and recent uplift
(Ollier & Pain, 2000).
It is important to recognize
planation surfaces correctly. They are products of bedrock
erosion, not sediment deposition. Horizontally-bedded
rocks tend to produce flat surfaces which are purely
structural. A planation surface is most easily recognized
when it cuts across, or bevels, diverse rocks with varied
internal structures (Figure 6b). Bevelled cuestas over
a wide area of folded or tilted rocks are a good indicator
of a former planation surface, because in the absence
of a former planation surface, cuestas – structurally
controlled landforms on dipping strata – would
show a sharp ridge crest, never a level top (Figure
6c,d). “Accordant summit levels” –complexly
deformed rocks of widely varying types with their summits
at roughly the same general level – also suggest
a former land surface somewhat higher and getting dissected
now (Figure 6e). Planation surfaces are of great significance
in tectonics, as Ollier & Pain (2000) show
with many examples worldwide. Planation surfaces at
rifted continental margins such as of East Greenland
have been used (Bonow et al., 2006a,b; Japsen
et al., 2006) to track post-volcanic uplift (post-mid-Eocene
and mostly Neogene) – there are obvious parallels
with the Sahyadri, which has experienced major uplift
in the Neogene.
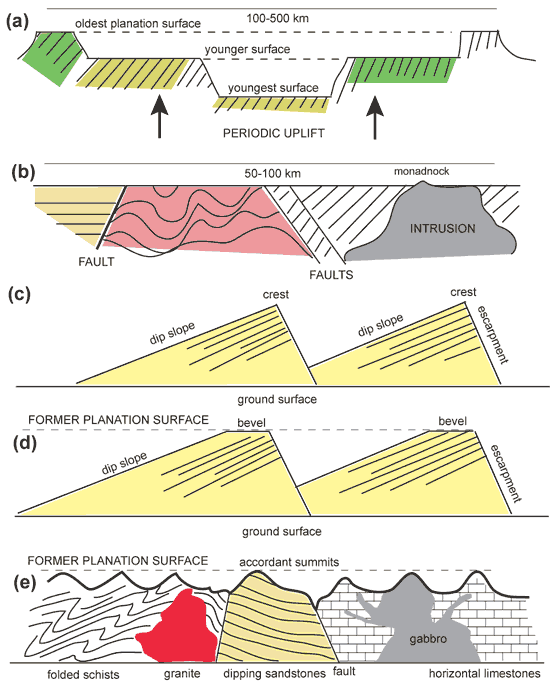
Figure 6. Schematic
sketches showing (a) Multiple, successive planation
surfaces in an area undergoing periodic uplift, i.e.,
a “polycyclic” landscape. (b) A planation
surface cut on complex rocks with a resistant monadnock
left behind. (c) Cuestas on dipping resistant rocks
normally have sharp crests. View is along strike direction.
(d) Bevelled cuestas representing a former planation
surface. (e) Accordance of summit levels in a lithologically
diverse and complexly deformed terrain suggesting a
former planation surface. Based on Ollier & Pain
(2000). The vertical scale is greatly exaggerated in
(a), (b), and (c).
Figure 7 shows a schematic
NNW-SSE profile (after Gunnell, 1998) through
southern India showing the multiple planation surfaces
that bevel varied bedrock types and structures. S0
(~2500 m elevation) is the original, Gondwanic planation
surface (late Jurassic or early Cretaceous), S1
(~2200 m) is a late Cretaceous surface well seen in
the Nilgiri massif (around Ooty, Figure 3) and surrounding
highlands, S2 probably an early- or mid-Tertiary
surface (identified partly based on bauxite occurrences
and suggested to be the top of the Deccan basalt pile),
and S3 a late Tertiary surface. S4
is the lowest and youngest surface, Mio-Pliocene in
age. Post-denudational upwarping has produced a fanning
of the surfaces S1 to S3, which
according to Gunnell (1998) is probably unrelated
to the rift-flank uplift of the Western Ghats, but to
crustal loading by the Deccan basalt pile and late Neogene
intraplate crustal deformation in the Indian Ocean Basin
that has been affecting peninsular India as well (Subrahmanya,
1996).

Figure 7. Schematic
NNW-SSE profile through the polycyclic landscape of
southern India, showing the multiple planation surfaces.
After Gunnell (1998). Click here
or on figure for enlargement.
Sub-Deccan
planation surfaces in central India
As noted, the top of the
Deccan basalt pile in the Western Ghats, such as at
Mahabaleshwar (1436 m), is a heavily lateritized planation
surface of late Cretaceous to early Tertiary age (Figure
4a,b,c), developed after the eruptions during stable
tectonic conditions (Widdowson & Cox, 1996;
Widdowson, 1997a; Widdowson & Gunnell,
1999). Its present high elevation today reflects post-Deccan
uplift, very probably of >1 km. A planation surface
below the Deccan basalt pile in a region would
indicate long-term tectonic stability before
flood volcanism.
Evidence for pre-Deccan
planation surfaces is best sought in central India,
where the basalt-basement contacts are exposed over
large areas, and the basement is very diverse (Figure
2). Dixey (1970, reprinted in Subbarao,
1999) and Choubey (1971) identified erosion
surfaces under the Deccan lavas in central India, and
noted their usefulness in deciphering subsequent tectonics.
Choubey (1971) noted that in central India
most Vindhyan and Bijawar hills stand approximately
at a level of 590 m, a remarkable summit accordance
and considered these the remnants of a vast Cretaceous
peneplain. The Deccan basalts cap this surface. At lower
elevations, the basalts cap another planation surface,
developed on the softer Gondwana sediments. Both surfaces
existed before the Deccan eruptions, and represented
a long inverval of weathering with a deep lateritic
saprolith. Their main observations from specific areas
have been compiled by Sheth
(2007).
The sub-Deccan Gondwana Basin is spectacularly exposed
in the Satpura mountain range due to post-Deccan cymatogenic
uplift (Figure 8a,b) (Venkatakrishnan, 1984,
1987). Pachmarhi town sits on a thick (~1000 m) lens
of mid-Triassic sandstone containing subordinate shales
and conglomerates, and capped by extensive ferricrete.
A very spectacular feature is the ENE-WSW-trending,
160-km-long, up to 280 m high, south-facing, free-face
scarp that has been carved into the Pachmarhi Sandstone
(Figure 8a,b). Many outliers of this sandstone lie scattered
south of the present position of the scarp, which has
been retreating northwards. The Pachmarhi sandstones
are underlain by the Bijori Formation (Permian) dominated
by shales (Figure 8b). Many Deccan dolerite dykes and
intrusions can be found in the Pachmarhi and Bijori
rocks at lower elevations. The dipping strata are beveled
by planation surfaces recognized by Venkatakrishnan
(1984, 1987). The lowest and youngest is the Bijori
Surface (640 m) that is currently forming, and slopes
northwards towards Pachmarhi from the basalt cliffs
to the south. Older and higher is the Pachmarhi Surface
(920 m), and still older and higher is the Dhupgarh
Surface (~1300 m) preserved in the three accordant sandstone
summits of Dhupgarh (1352 m), Mahadeo (1330 m) and Chauragarh
(1308 m) that rise over Pachmarhi. Venkatakrishnan
(1984) suggested that the Narmada river valley to the
north of Pachmarhi has been recurrently acting as a
local base level, periodically rising and falling, for
streams eroding the plateau.
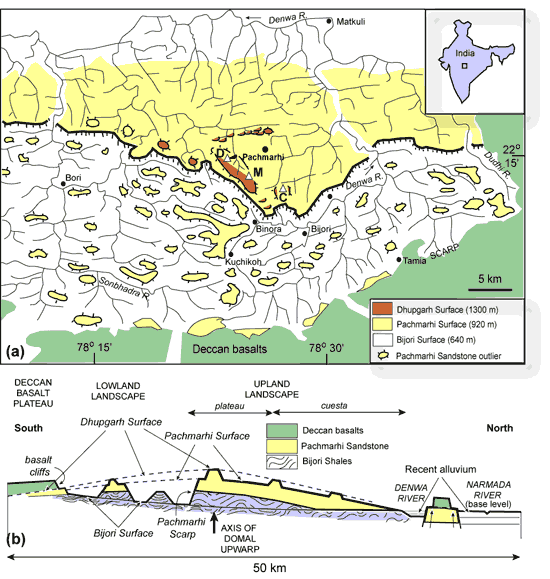
Figure 8. (a) Geomorphological
map of the Pachmarhi region on the Satpura dome, showing
the Pachmarhi scarp, planation surfaces, structural
elements, Pachmarhi Sandstone outliers, drainage lines,
and some localities. The many Deccan Trap dykes and
intrusions in the sandstones are not shown for clarity.
D is Dhupgarh (1352 m), M is Mahadeo (1330 m) and C
is Chauragarh (1308 m). Based on Venkatakrishnan (1984).
(b) Generalized geological cross-section of the Pachmarhi
area showing the main landscape elements, slightly modified
from Venkatakrishnan (1987). Note the upwarped planation
surfaces, and that the surfaces bevel the internal structure
of the Pachmarhi Sandstones and the underlying Bijori
Shales. The surfaces are also characterized by laterite/ferricrete
development. The highest and oldest planation surface
is the Dhupgarh Surface (only approximate in (a), and
it is the pre-Deccan-Trap erosion surface of Choubey
(1971), and the late Cretaceous peneplain of Dixey (1970).
On Dhupgarh, the highest
peak in the Satpura Range, a Deccan basalt flow unconformably
overlies the north-dipping Pachmarhi Sandstone (Venkatakrishnan,
1984), and he considered the base of the basalt flow
– the Dhupgarh Surface – to be the same
as the late Cretaceous peneplain of Dixey (1970),
the sub-Deccan Trap erosion surface of Choubey
(1971), and probably also the inter-continental Gondwana
or African Surface of King (1953). Though the
sandstone-basalt contact at Dhupgarh is an unconformity,
this does not mean uplift and erosion right before the
Deccan eruptions, because the entire rock record from
mid-Triassic to late Cretaceous is missing. Also, because
the unconformity is a regional planation surface, indicating
long-term tectonic stability, the missing rock record
cannot be ascribed to rapid erosion just before volcanism.
Venkatakrishnan
(1984, 1987) observed that the multiple planation surfaces
around Pachmarhi were warped as a result of post-Deccan
uplift. He identified the doming and warping in the
Satpura region on the basis of the warped contact between
the Pachmarhi sandstones and the Bijori shales, reversals
of structural dips, warped planation surfaces, cave
levels, and the drainage pattern. A key observation
by him is that the rivers such as Denwa and Dudhi
originate well to the south of the present-day scarp,
several hundred meters below the Pachmarhi Surface,
and have cut their way northward through the evolving
Satpura dome in spectacular gorges (Figure 8a).
He suggested that the most reasonable explanation of
this is drainage antecedence.
To summarize, the uplift
and warping of the Pachmarhi block, with its planation
surfaces, is post-Deccan (just as the uplift of the
Sahyadri and the Indian peninsula is post-Deccan). What
is the cause of this post-Deccan uplift? The Sahyadri
uplift (all along the rifted margin) cannot be related
to any magmatic underplating that may have occurred
during Deccan volcanism, because such essentially thermal
uplift decays rapidly after magmatism (Clift,
2005). The uplift and deformation of large regions
of peninsular India may be related at least in part
to the compressional stress regime throughout peninsular
India since the India-Asia collision at ~55 Ma (Valdiya,
1998), which is causing reactivation of ancient and
older weak zones and much recent intraplate seismicity
(Subrahmanya, 1994; Valdiya, 2001;
Vita-Finzi, 2002). Sahyadri uplift is denudational
in part (Widdowson & Cox, 1996; Widdowson
& Gunnell, 1999), and may also be potentially
related also to active eastward flow of the sub-lithospheric
mantle relative to the lithosphere as hypothesized by
Doglioni
et al.
(2003).
Discussion
and conclusions
Campbell & Griffiths
(1990) cited Pachmarhi as the center of a broad, uplifted
dome produced by their conjectured plume head, quoting
Choubey (1971) that the lava-basement contact
at Pachmarhi is over 1 km above sea level. But the doming
of the Pachmarhi region and the entire Satpura Range,
is not pre-Deccan but post-Deccan. Casshyap
& Khan
(2000) argued for “pre-Deccan Trap doming”
of the Indian landmass, based on sedimentological studies
around Pachmarhi, but this doming cannot be related
to a putative Deccan plume by any means. They identified
three separate uplift events, the youngest of which
resulted in late Jurassic to earliest Cretaceous sediments
derived from northwestern India. An uplift event centered
on northwestern India, and preceding Deccan volcanism
by ~70 m.y., cannot be considered pre-volcanic uplift
from the Deccan plume.
The flatness of the pre-Deccan
landscape constructed on various older rocks in central
India, the horizontality of the Deccan basalt flows
over long distances, and laterites found on the pre-Deccan
landscape, together form compelling evidence for pre-Deccan
planation surfaces and long-term tectonic stability
prior to the eruptions. This, along with a near-universal
absence of indicators of pre-eruption uplift throughout
the province, runs counter to the idea that a large
plume head produced regional domal uplift, the current
drainage pattern, and Deccan flood basalt volcanism.
There has been major, kilometer-scale post-Deccan uplift,
which has brought the pre-Deccan planation surfaces
from their originally low elevations (relative to the
then-existing base level) to their present high elevations.
A very similar scenario has been noted in the flood
basalts of Yemen by Menzies et al. (1997).
How are we to explain
the lack of pre-eruption uplift in flood basalts? Campbell
& Griffiths (1990) argued that pre-volcanic
regional domal uplift due to a plume head may not be
significant due to lateral migration of magma in the
crust, or difficult to recognize as early uplift may
be overprinted by later subsidence. These are not satisfactory
explanations. Subsidence that overprints and erases
evidence of early pre-eruption uplift is simply not
seen in flood basalts like the Deccan, which show major
post-volcanic uplift instead (Sheth,
2007). This uplift should expose evidence of early,
pre-eruption uplift, if there ever was any. Burov
& Guillou-Frottier (2005) argue that uplift
patterns can be complex depending on factors such as
lithospheric strength and plume excess temperature,
buoyancy and shape; all plumes do not cause uplift and
flood basalt provinces without pre-volcanic uplift may
well be plume-generated [Ed: See also Lithosphere
Uplift page]. If so the model makes no specific
and testable predictions for field geologists and cannot
be evaluated against their observations. The plume head
model would become untenable, however, when a flood
basalt province shows not merely an absence of evidence
for pre-volcanic uplift, but also evidence for absence
of such uplift. This is exactly the case in the Deccan.
Nevertheless, Campbell
(2005) claims that all the predictions of the model,
including that of pre-volcanic regional uplift, are
supported by data from flood basalt provinces of the
world, the Deccan included.
In summary, the uplift
of the Sahyadri (Western Ghats), India, is not related
to a Deccan mantle plume: it is not domal, it occurred
all along the western Indian rifted margin, well beyond
the Deccan lava cover, and is most appropriately considered
a rift-shoulder uplift affecting the Deccan basalt pile
and the basement rocks equally, and maintained at least
in part by denudational unloading (Widdowson,
1997a). Planation surfaces below the basalts reflect
long-term tectonic stability prior to volcanism, and
their significant elevations today reflect post-Deccan
uplift, major uplift having occurred in the Neogene
(i.e., <23 m.y.). The uplift may have been aided
by a compressional regime throughout the Indian shield
since about 55 Ma. The easterly drainage of the Indian
peninsula is not dome-flank drainage produced by a plume
head but is antecedent to the uplift. The uplift of
both the Western and the Eastern Ghats postdates Deccan
volcanism and the easterly drainage. There is thus not
only an absence of evidence for pre-volcanic regional
uplift in the Deccan flood basalt province, but actual
evidence for absence thereof. The abundant plume conjecture
in the voluminous Deccan literature has no factual basis
(Sheth, 2005a,b,
2007).
Acknowledgements
This article is a condensed
version of Sheth
(2007), which greatly benefitted from very helpful
reviews by Richard Ernst, Mike Widdowson, Yanni Gunnell,
Colin Pain, and an anonymous reviewer, unofficial comments
by Rajat Mazumder and Peter Japsen, and editorial comments
by Gillian Foulger and Donna Jurdy.
References
-
Baksi, A. K., Byerly,
G. R., Chan, L.-H., and Farrar, E., 1994, Intracanyon
flows in the Deccan province, India? Case history
of the Rajahmundry Traps: Geology, v. 22,
p. 605-608.
-
Bendick, R., and
Bilham, R., 1999, Search for buckling of the southwest
Indian coast related to Himalayan collision, in
McFarlane, A., Sorkhabi, R. B., and Quade, J., eds.,
Himalaya and Tibet: Mountain roots to mountain tops:
Geological Society of America Special Paper,
v. 328, p. 313-321.
-
Bonow, J. M., Lidmar-Bergström,
K., and Japsen, P., 2006a, Palaeosurfaces in central
West Greenland as reference for identification of
tectonic movements and estimation of erosion: Global
and Planetary Change, v. 50,
p. 161-183.
-
Bonow, J. M., Japsen,
P., Green, P. F., Chalmers, J. A., and Lidmar-Bergström,
K., 2006b, Significance of elevated planation surfaces
for conclusions of uplift and tectonic events on
passive margins: Geophysical Research Abstracts,
v. 8, 09869.
-
Burov, E., and Guillou-Frottier,
L., 2005, The plume head-continental lithosphere
interaction using a tectonically realistic formulation
for the lithosphere: Geophysical Journal International,
v. 161, p. 469-490.
-
-
Campbell, I. H.,
and Griffiths, R. W., 1990, Implications of mantle
plume structure for the evolution of flood basalts:
Earth and Planetary Science Letters, v.
99, p. 79-93.
-
-
Choubey, V. D.,
1971, Pre-Deccan Trap topography in central India
and crustal warping in relation to Narmada rift
structure and volcanic activity: Bulletin of
Volcanology, v. 35, p. 660-685.
-
Clift,
P. D., 2005, Sedimentary evidence for modest mantle
temperature anomalies associated with hotspot volcanism,
in Foulger, G. R., Natland, J. H., Presnall, D.
C., and Anderson, D. L., eds., Plates, plumes,
and paradigms: Boulder, Colorado, Geological
Society of America Special Paper 388, p. 279-287.
-
Cox, K. G., 1989,
The role of mantle plumes in the development of
continental drainage patterns: Nature,
v. 342, 873-877.
-
Dixey, F., 1970,
The geomorphology of Madhya Pradesh, India, in Murthy,
T. V. V. G. R. K., ed., Studies in earth sciences:
W. D. West Commemoration Volume, Today and
Tomorrow Publishers, New Delhi, p. 195-224.
-
-
Farnetani, C., and
Richards, M. A., 1994, Numerical investigations
of the mantle plume initiation model for flood basalt
events: Journal of Geophysical Research,
v. 99, p. 13,813-13,833.
-
Gunnell, Y., 1998,
The interaction between geological structure and
global tectonics in multistoreyed landscape development:
A denudation model for the South Indian shield:
Basin Research, v. 10,
p. 281-310.
-
Gunnell, Y., and
Gallagher, K., 2001, Short- and long-term denudation
rates in cratonic environments: Estimates for Precambrian
Karnataka (South India), in Gunnell, Y., and Radhakrishna,
B. P., eds., 2001, Sahyadri: The great escarpment
of the Indian subcontinent: Bangalore, Geological
Society of India Memoir 47
(1-2), p. 445-461.
-
Gunnell, Y., and
Louchet, A., 2000, Rock hardness and long-term divergent
weathering as factors of relief development in the
charnockite massifs of South Asia: Zeitschrift
für Geomorphologie, v. 44,
p. 33-57.
-
Gunnell, Y., and
Radhakrishna, B. P., eds., 2001, Sahyadri: The
great escarpment of the Indian subcontinent:
Bangalore, Geological Society of India Memoir 47
(1-2), 1054 p.
-
Gunnell,
Y., Gallagher, K., Carter, A., Widdowson, M., and
Hurford, A. J., 2003, Denudation history of the
continental margin of western peninsular India since
the early Mesozoic – reconciling apatite fission-track
data with geomorphology: Earth and Planetary
Science Letters, v. 215, p.
187-201.
-
Harris, J., and
van Couvering, J., 1995, Mock aridity and the palaeoecology
of volcanically influenced ecosystems: Geology,
v. 23, p. 593-596.
- Japsen,
P., Bonow, J. M., Green, P. F., Chalmers, J. A., and
Lidmar-Bergstrom, K., 2006, Elevated, passive continental
margins: Long-term highs or Neogene uplifts? New evidence
from West Greenland. Earth and Planetary Science
Letters (in press).
-
Kaila, K. L., 1988,
Mapping the thickness of Deccan Trap flows in India
from DSS studies and inferences about a hidden Mesozoic
basin in the Narmada-Tapti region, in Subbarao,
K. V., ed., Deccan Flood Basalts: Bangalore,
Geological Society of India Memoir 10, p. 91-116
- King, L. C., 1953, Canons of landscape
evolution. Geological Society of America Bulletin,
v. 64, 721-752.
-
Menzies, M. A.,
Baker, J., Chazot, G., and Al’Kadasi, M.,
1997, Evolution of the Red Sea volcanic margin,
western Yemen, in Mahoney, J. J., and Coffin, M.
F., eds., Large igneous provinces: Oceanic,
continental, and planetary flood volcanism:
Washington, D.C., American Geophysical Union Geophysical
Monograph 100, p. 29-43.
-
Mohabey, D., 1996,
Depositional environment of Lameta Formation (Late
Cretaceous) of Nand-Dongargaon inland basin, Maharashtra:
The fossil and lithological evidences, in Sahni,
A., ed., Cretaceous stratigraphy and palaeoenvironments:
Bangalore, Geological Society of India Memoir 37,
p. 363-386.
-
Norton, I. O., and
Sclater, J. G., 1979, A model for the evolution
of the Indian Ocean and the breakup of Gondwanaland:
Journal of Geophysical Research, v. 84,
p. 6803-6830.
-
Ollier, C. D., and
Powar, K. B., 1985, The Western Ghats and the morphotectonics
of peninsular India: Zeitschrift fuer Geomorphologie,
Suppl. Bd., v. 54, p. 57-69.\
-
Ollier, C. D., and
Pain, C. F., 2000, The origin of mountains:
London, Routledge, 368 p.
-
Pande,
K., Sheth, H. C., Bhutani, R., 2001. 40Ar/39Ar
age of the St. Mary's Islands volcanics, southern
India: record of India-Madagascar break-up on the
Indian subcontinent, Earth Planet. Sci. Lett.,
193, 39-46.
-
Powar, K. B., 1993,
Geomorphological evolution of Konkan coastal belt
and adjoining Sahyadri uplands with reference to
Quaternary uplift: Current Science, v.
64, p. 793-796.
-
Radhakrishna, B.
P., 1952, The Mysore plateau: Its structural and
physiographical evolution. Bulletin of the Mysore
Geologists Association, v. 3,
p. 1-53. (Reprinted in Gunnell and Radhakrishna,
2001).
-
Radhakrishna, B.
P., 1993, Neogene uplift and geomorphic rejuvenation
of the Indian peninsula: Current Science,
v. 64, p. 787-793.
-
Rainbird, R. H.,
and Ernst, R. E., 2001, The sedimentary record of
mantle plume uplift, in Ernst, R. E., and Buchan,
K. L., eds., Mantle plumes: Their identification
through time: Boulder, Colorado, Geological
Society of America Special Paper 352, p. 227-245.
-
Ray, J. S., Pande,
K., and Pattanayak, S. K., 2003, Evolution of the
Amba Dongar carbonatite complex: Constraints from
40Ar/39Ar chronologies of the inner basalt and an
alkaline plug: International Geology Review,
v. 45, p. 857-862.
-
Richards, M. A.,
Duncan, R. A., and Courtillot, V. E., 1989, Flood
basalts and hotspot tracks: Plume heads and tails:
Science, v. 246, p. 103-108.
-
Sheth,
H. C., 2005a. From Deccan to Réunion: no
trace of a mantle plume. In: Foulger, G. R., Natland,
J. H., Presnall, D. C., Anderson, D. L. (Eds.),
Plates, Plumes, and Paradigms. Geol. Soc. Am.
Spec. Pap. 388, 477-501.
-
-
Sheth,
H. C., 2007, Plume-related regional pre-volcanic
uplift in the Deccan Traps: Evidence of absence,
absence of evidence, in Foulger, G. R., and Jurdy,
D. M., eds., The origins of melting anomalies:
Plates, plumes, and planetary processes:
Boulder, Colorado, Geological Society of America
Special Paper, accepted.
-
-
Sridhar, A. R.,
and Tewari, H. C., 2001, Existence of a sedimentary
graben in the western part of the Narmada zone:
Seismic evidence: Journal of Geodynamics,
v. 31, p. 19-31.
-
Storey, M., Mahoney,
J. J., Saunders, A. D., Duncan, R. A., Kelley, S.
P., and Coffin, M. F., 1995, Timing of hotspot-related
volcanism and the breakup of Madagascar and India:
Science, v. 267, p. 852-855.
-
Subbarao, K. V.,
ed., 1999, Deccan volcanic province, v. 1-2: Bangalore,
Geological Society of India Memoir 43,
947 p.
-
Subrahmanya, K.
R., 1994, Post-Gondwana tectonics of peninsular
India: Current Science, v. 67,
p. 527-530.
-
Subrahmanya, K.
R., 1996, Active intraplate deformation in South
India: Tectonophysics, v. 262,
p. 231-241.
-
Summerfield, M.
A., 1990, Geomorphology and mantle plumes: Nature,
v. 344, p. 387-388.
-
Summerfield, M.
A., 1991, Sub-aerial denudation of passive margins:
Regional elevation versus local relief models:
Earth and Planetary Science Letters, v. 102,
p. 460-469.
-
-
Vaidyanadhan,
R., 1977, Recent advances in geomorphic studies
of peninsular India: A review: Indian Journal
of Earth Sciences, S. Ray Volume, p. 13-35.
-
Valdiya, K. S.,
1998, Dynamic himalaya: Bangalore, Universities
Press, 198 pp.
-
Valdiya, K. S.,
2001, Tectonic resurgence of the Mysore plateau
and surrounding regions in cratonic southern India:
Current Science, v. 81,
p. 1068-1089.
-
Venkatakrishnan,
R., 1984, Parallel scarp retreat and drainage evolution,
Pachmarhi area, Madhya Pradesh, central India: Journal
of Geological Society of India, v. 25,
p. 401-413.
-
Venkatakrishnan,
R., 1987, Correlation of cave levels and planation
surfaces in the Pachmarhi area, Madhya Pradesh:
A case for base level control: Journal of Geological
Society of India, v. 29, p.
240-249.
Vita-Finzi, C., 2002, Intraplate neotectonics in
India: Current Science, v. 82,
p. 400-402.
-
Widdowson, M., 1997a,
Tertiary palaeosurfaces of the SW Deccan, western
India: Implications for passive margin uplift, in
Widdowson, M., ed., Palaeosurfaces: Recognition,
reconstruction, and palaeoenvironmental interpretation:
London, Geological Society Special Publication,
v. 120, p. 221-248.
-
Widdowson, M., 1997b,
The geomorphological and geological importance of
palaeosurfaces, in Widdowson, M., ed., Palaeosurfaces:
Recognition, reconstruction, and palaeoenvironmental
interpretation: London, Geological Society
Special Publication, v. 120, p. 1-12.
-
Widdowson, M., 2005,
The Deccan basalt – basement contact: Evidence
for a plume-head generated CFBP? American Geophysical
Union Chapman Conference “The Great Plume
Debate”, Scotland, p. 69-70 (abstract).
-
Widdowson, M., and
Cox, K. G., 1996, Uplift and erosional history of
the Deccan Traps, India: Evidence from laterites
and drainage patterns of the Western Ghats and Konkan
coast: Earth and Planetary Science Letters,
v. 137, p. 57-69.
-
Widdowson, M., and
Gunnell, Y., 1999, Lateritization, geomorphology
and geodynamics of a passive continental margin:
The Konkan and Kanara coastal lowlands of western
peninsular India: Special Publication of the
International Association of Sedimentologists,
v. 27, p. 245-274.
-
Williamson, I. T.,
and Bell, B. R., 1994, The Palaeocene lava field
of west-central Skye, Scotland: Stratigraphy, palaeogeography
and structure: Transactions of the Royal Society
of Edinburgh: Earth Sciences, v. 85,
p. 39-75.
last updated 29th
August, 2006 |