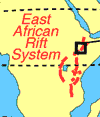 |
Control of pre-rift lithospheric structure on the architecture and evolution of continental rifts: insights from the Main Ethiopian Rift, East Africa |
Giacomo Corti1, Paola Molin2, Andrea Sembroni2, Ian D. Bastow3, Derek Keir4,5
1Consiglio Nazionale delle Ricerche, Istituto di Geoscienze e Georisorse, Via G. La Pira, 4, 50121 Florence, Italy; giacomo.corti@igg.cnr.it
2Università degli Studi di Roma Tre, Dipartimento di Scienze, Largo San Leonardo Murialdo, 1, 00146 Roma, Italy; paola.molin@uniroma3.it ; andrea.sembroni@uniroma3.it
3Department of Earth Science and Engineering, Imperial College London, London, SW7 2AZ, U.K.; i.bastow@imperial.ac.uk
4Ocean and Earth Science, University of Southampton, Southampton, SO14 3ZH, U.K.; D.Keir@soton.ac.uk
5Dipartimento di Scienze della Terra, Università degli Studi di Firenze, via G. La Pira 4, 50121 Florence, Italy
Continental rifts often display considerable along-strike variations in architecture and magmatism, which are commonly explained in terms of variations in parameters such as rift kinematics, extension rate, mantle characteristics, pre-existing lithospheric rheology (e.g., Keir et al., 2015; Brune, 2016). Since continental rifting results from the application of extensional stresses to a pre-deformed, anisotropic lithosphere, pre-existing plate structure is expected to play a major role in controlling the rifting process (e.g., Ziegler & Cloetingh, 2004; Corti, 2012; Brune, 2016). Recent studies support this and indicate that along-axis variations in basement fabrics have a strong control on rift segmentation, inducing significant variations in the characteristics of the rift margins (e.g., vertical throw on major boundary faults) and the symmetry or asymmetry of the rift basins (Lao-Davila et al., 2015; Grimmer et al., 2017). Along-axis variations in rift symmetry/asymmetry may be also influenced by surface processes (dynamic coupling between flexural uplift and fluvial erosion). Cross-sectional basin architecture is also interpreted to be time-dependent, with a progression from asymmetric to symmetric rifting, eventually followed by axial focusing of the volcanism and faulting (e.g., WoldeGabriel et al., 1990; Hayward & Ebinger, 1996).
To address these problems, we investigated the along-axis variations in architecture, segmentation and evolution of the Main Ethiopian Rift (MER), East Africa (Figure 1), to relate these characteristics to the regional geology, lithospheric structure and surface processes. The MER is a classic example of continental rifting developed within a highly anisotropic lithosphere that has experienced several pre-rift tectonic events (e.g., Abbate et al., 2015). It is an ideal study locale for the analysis of rift structure in conjunction with surface processes since the expression of different stages in the evolutionary rift sequence, from initial rifting in the south to incipient break-up in the north, are all subaerially exposed and rigorously studied at depth using geophysical techniques (e.g., Hayward & Ebinger, 1996).
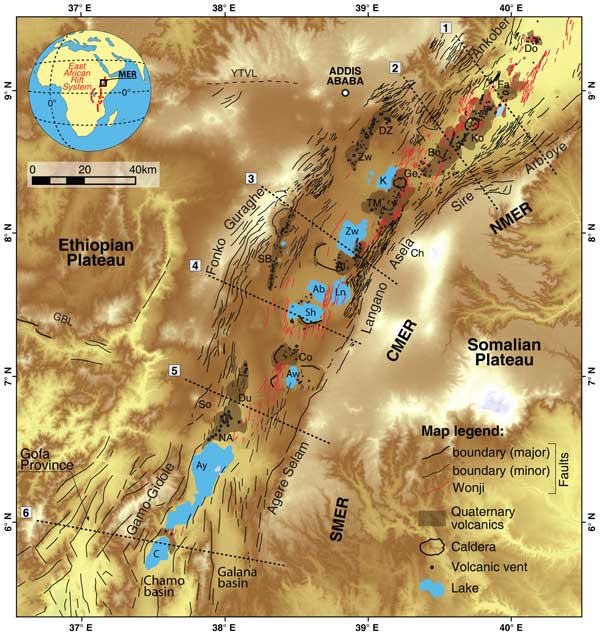
Figure 1: Fault pattern and Quaternary volcanics in the Main Ethiopian Rift (MER) superimposed on a NASA Shuttle Radar Topography Mission (SRTM) digital elevation model. The three main rift sectors are labelled NMER, Northern MER; CMER, Central MER; SMER, Southern MER. Dashed lines with numbers indicate cross-sections illustrated in Figure 2. Volcanic fields and calderas are labelled Al: Aluto; Bo, Boseti; Co, Corbetti; Do, Dofan; Du, Duguna; DZ, Debre Zeyt; Fa, Fantale; Ge, Gedemsa; Ko, Kone; NA, North Abaya; SB, Silti-Butajira; TM, Tulu Moye; Zw, Zikwala. Lakes are labelled Ab, Lake Abijata; Ay, Abaya; Aw, Awasa; C, Lake Chamo; Ch: Chilalo; K, Lake Koka; Ln, Langano; Sh, Shala; Zw, Lake Ziway. Other labels: GBL: Goba-Bonga transversal lineament; So: Soddo; YTVL: Yerer-Tullu Wellel volcano-tectonic lineament.
Analysis of simplified geological cross-sections of different portions of the rift (Figure 2), show significant along-axis variations in the distribution of extension-related deformation and basin architecture. In particular, ~70% of the 500 km-long MER is asymmetric, with most asymmetric sectors characterized by a master fault system on the eastern margin. More than 70% of the eastern margin is marked by a major boundary fault; the rest is marked by minor faulting and monoclines. The symmetry or asymmetry of the rift is reflected in the topography and hydrography. The rift margin topography gradients differ by <40% in the symmetric portions and >100% in the asymmetric ones. Steep rivers deeply incise the footwall of the major border faults. This erosional unloading increases the isostatic uplift of footwall, enhancing the symmetric/asymmetric topography of each sector of the rift.
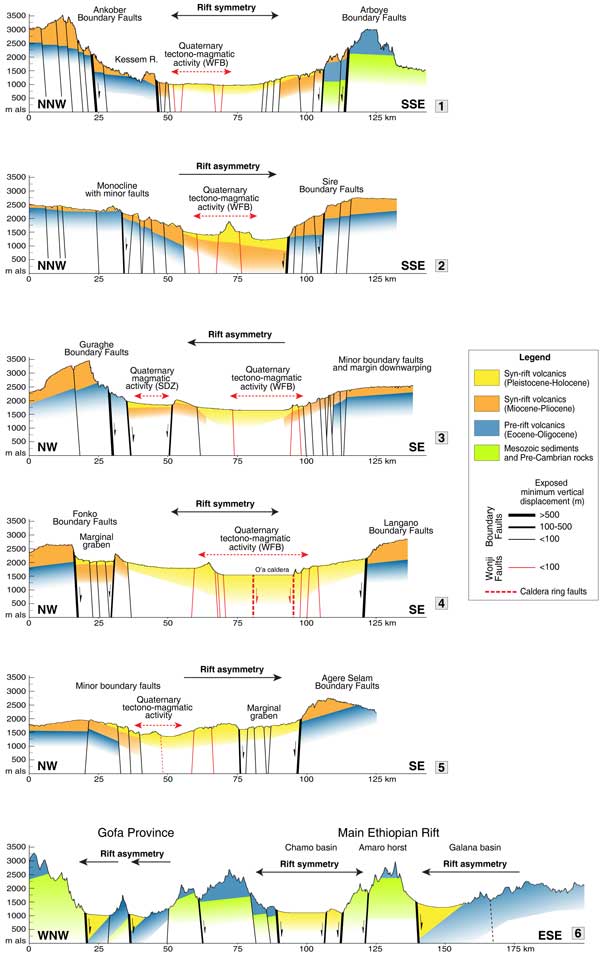
Figure 2: Simplified geological profiles across different sectors of the MER. Topographic profiles are extracted from SRTM digital elevation models, with vertical exaggeration of 10. WFB: Wonji Fault Belt; SDZ: Silti Debre Zeit volcanic belt. Click here or on Figure for enlargement.
Our analysis provides strong evidence that rift architecture and its along-axis variations are controlled by the pre-rift lithospheric structure, especially the contrasting nature of the two plateaus surrounding the rift (Figure 3). Specifically, the limited along-axis variations in architecture of the eastern rift margin, dominated by large boundary escarpments along almost its entire length, likely reflect the strong and homogeneous nature of the lithosphere beneath the Somalian Plateau (e.g., Keranen & Klemperer, 2008). Conversely, the more irregular nature of the western margin, with segments characterised by large boundary faults alternating with segments marked by gentle flexures towards the rift axis, likely reflects the more complex structure of the Ethiopian plateau lithosphere. This latter is interpreted to comprise two different portions: a strong northern portion, strengthened by cooled (pre-rift) mafic underplate, and a southern portion, with thinner crust and weaker rheology (Figure 3b; e.g., Keranen & Klemperer, 2008). This southern portion is marked by the presence of two major transversal lineaments, resulting from the Cenozoic reactivation of pre-existing Neoproterozoic weaknesses (e.g., Abebe Adhana, 2014). Where these pre-existing weak heterogeneities intersect the rift at a high angle, the western margin lacks major boundary faults, which are instead replaced by gentle monoclines. This, together with well-developed faults on the eastern side, results in an overall asymmetry of the rift (Figure 3).
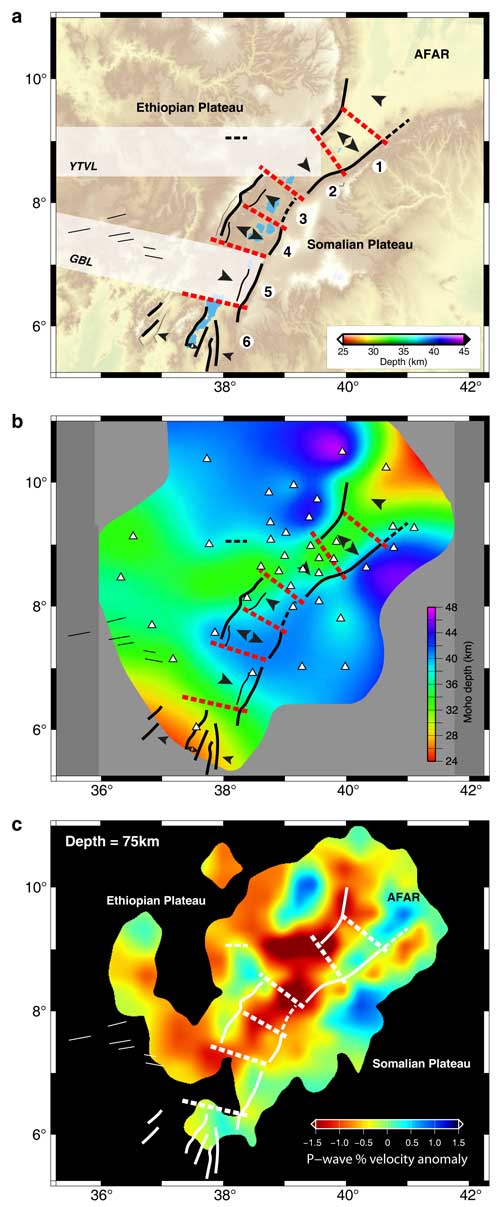
Figure 3: a) Interpreted along-axis variations in the structure of the Main Ethiopian Rift. Single arrows indicate the polarity of different rift sectors; double arrows indicate symmetry of rifting. Whitish boxes indicate the hypothesised extent of the transverse Yerer-Tullu Wellel (YTWL) and Goba-Bonga (GBL) volcano-tectonic lineaments. b) Contour map of Moho depth in the MER (after Keranen et al., 2009). c) Depth slices through the P-wave velocity model of Bastow et al. (2008) at 75 km depth. See text for further details.
Finally, we show how the data provided by this integrated analysis suggest that rift sectors characterised by asymmetric architecture can directly progress to focused axial tectono-magmatic activity, without transitioning into a symmetric rifting stage. This challenges previous views of evolution of rifting in the East African Rift System (e.g., WoldeGabriel et al., 1990; Hayward & Ebinger, 1996). These observations have important implications for the asymmetry of continental rifts and conjugate passive margins worldwide, which has often been explained in the context of low-angle detachment faults and models involving a lithospheric-scale simple shear deformation (e.g., Wernicke, 1985).
References
-
Abbate, E., Bruni P., and Sagri, M. (2015). In: P. Billi (ed.), Landscapes and Landforms of Ethiopia, World Geomorphological Landscapes, Springer Science+Business Media, Dordrecht, pp. 33-64.
-
Abebe Adhana, T. (2014). J. Afr. Earth Sci., 99, 374-385.
-
Bastow, I. D., Nyblade, A. A., Stuart, G. W., Rooney, T. O., and Benoit, M. H. (2008). Geochem. Geophys. Geosyst., 9, Q12022, doi:10.1029/2008GC002107.
-
Brune, S. (2016), In by J. C. Duarte and W. P. Schellart, Plate Boundaries Nat. Hazards, 11–37, doi:10.1002/9781119054146.ch2.
-
Corti, G. (2012). Tectonophysics, 522-523, 1-33, http://dx.doi.org/10.1016/j.tecto.2011.06.010
-
Grimmer, J. C., Ritter, J. R. R., Eisbacher, G. H., and Fielitz, V. (2017). Int J Earth Sci (Geol Rundsch), 106, 827–853, DOI 10.1007/s00531-016-1336-x.
-
Hayward, N.J. and Ebinger, C.J. (1996). Tectonics, 15, 244-257.
-
Keir, D., Bastow, I.D., Corti, G., Mazzarini, F., and Rooney, T.O. (2015). Tectonics, 34, 464-477, DOI: 10.1002/2014TC003698.
-
Keranen, K., and S.L. Klemperer (2008). Earth and Planetary Science Letters, 265, 96-111, doi:10.1016/j.epsl.2007.09.038.
-
Keranen K., Klemperer S.L., Julia J., Lawrence J.L., and Nyblade A. (2009). Geochem. Geophys. Geosyst., 10, Q0AB01, doi:10.1029/2008GC002293.
-
Laó-Dávila, D.A., Al-Salmi, H.S., Abdelsalam, M.G., and Atekwana, E.A. (2015). Tectonics, 34, 2399–2417, doi:10.1002/2015TC003953.
-
Wernicke, B. (1985). Can. J. Earth Sci., 32, 108-125.
-
WoldeGabriel, G., Aronson, J. L., and Walter, R. C. (1990). Geological Society America Bulletin, 102, 439-458.
-
Ziegler, P. A. and S. Cloetingh (2004). Earth-Sci. Rev., 64, 1–50, doi:10.1016/S0012-8252(03)00041-2.
last updated 2nd January, 2019 |