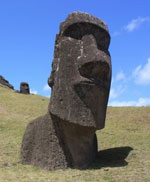 |
The Easter – Sala y Gomez Volcanic Chain |
1Lucy J. Milligan, 1Abigail Metcalfe,1Lucy K. Brooks, 1Dheyna Scholtz, 1Laura J. Scott, 1Gillian R. Foulger, 2David F. Naar, 3James
H. Natland & 4Enrico Bonatti
1Dept. Earth Sciences, Science Laboratories, South Rd., Durham DH1 3LE, U.K., l.j.milligan@durham.ac.uk ; a.l.metcalfe@durham.ac.uk ; l.k.brooks@durham.ac.uk ; dheyna.scholtz@durham.ac.uk ; l.j.scott2@durham.ac.uk ; g.r.foulger@durham.ac.uk
2University of South Florida, College of Marine Science, St Petersburg, FL 33701-5016, naar@usf.edu
3RSMAS/MGG University of Miami,
Miami, FL 33149, jnatland@msn.com
4Istituto Scienze Marine, Geologia Marina, CNR, Via Gobetti 101, 40129 Bologna, Italy; enrico.bonatti@bo.ismar.cnr.it
Introduction
This webpage briefly summarises the cases for the Plume and Plate hypotheses. The Plume hypothesis attributes intraplate volcanism to a hot diapir that rises from the core-mantle boundary and is fixed relative to other plumes. The Plate hypothesis attributes melt to lithospheric extension that permits leakage to the surface of pre-existing melt that is widespread in the shallow mantle.
Easter and Sala y Gomez Islands lie on the Nazca plate in the Eastern Pacific on a zone of high topography known as the Sala y Gomez Ridge (Figure 1). At its eastern end the topographic high continues as the Nazca Ridge. The entire feature is ~ 4,100 km long and is mainly volcanic in origin. The islands themselves comprise the small summits of tall mountains only and expose just a tiny part of the ridge (Clarke & Dymond, 1977). Easter Island is 360 km east of the East Pacific Rise (EPR) and Sala y Gomez Island is an additional 390 km further east. The Easter microplate lies just west of Easter Island and formed starting at 5.2 Ma (about magnetic chron 3; Figure 1).
The Easter-Sala y Gomez ridge itself is ~ 2,900 km long and ~150 km wide. Estimates of the true width of the bathymetric high vary and are as large as 400 km. The Nazca Ridge is ~ 1,200 km long and ~ 300 km wide. The whole zone ages, in general, to the east. Another chain of seamounts, the Pitcairn-Gambier chain, extends from the East Pacific Rise (EPR) to the west. It also features the occasional island, including Pitcairn Island which was volcanically active at ~ 3.0-0.3 Ma; Gilliou et al., 1997).
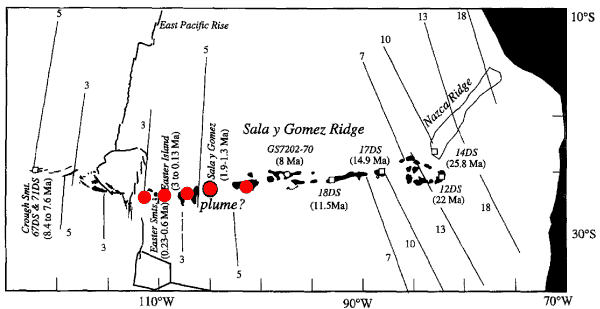
Figure 1: K-Ar ages show islands become older from 8 Ma to ~26 Ma to the east from the East Pacific Rise. Seafloor age anomalies are shown as long narrow lines, along with associated magnetic Chron numbers (approximate ages are Chron 3=5 Ma, 5=10 Ma, 7=24 Ma, 10=28 Ma, 13=34 Ma, 18=39 Ma).Red dots indicate the several locations that have been proposed for an Easter plume: Easter Island (Morgan, 1971), Sala y Gomez Island (Ray et al., 2012), 360 km E of Sala y Gomez (O'Connor et al., 1995), west of Easter Island (Haase et al., 1996) and between Easter and Sala y Gomez islands (Montelli et al., 2006). Basemap from O'Connor et al. (1995).
Current volcanism occurs along the EPR (Clouard & Bonneville, 2001) at ~ 100°W. The spreading rate just north of the Easter microplate is the higest anywhere on Earth at ~ 19 cm/a (Müller et al., 2008).
The Sala y Gomez and Nazca ridges have popularly been attributed to motion of the Nazca plate over a mantle plume (Morgan, 1971). Several different current localities for such a plume have been suggested, including one beneath Easter Island (Morgan, 1971), one beneath Sala y Gomez Island (Ray et al., 2012), one 360 km E of Sala y Gomez (O'Connor et al., 1995), one west of Easter Island (Haase et al., 1996) and one between Easter and Sala y Gomez islands (Montelli et al., 2006) (Figure 1). Subaerial volcanism on the ridge has occurred in the period 0.13 - 2.4 Ma at Easter Island (O'Connor et al., 1995) and 1.3 - 1.9 Ma at Sala y Gomez Island (Bonatti et al., 1977; Clark & Dymond, 1977).
The Sala y Gomez Ridge and its western counterpart, the Pitcairn-Gambier chain, both intersect the EPR at the southern end of the Easter Microplate (Hey et al., 1995). The tectonic evolution of this is described in detail by Naar & Hey (1991) and Rusby & Searle (1995) review its history. The microplate began to form at ~ 5.2 Ma (Chron 3) when the East Rift started to propagate northward. This lasted until ~ 2.5 Ma during which time the West Rift adjusted by segmenting along new transform faults. The transpressive northern boundary migrated with the northward-migrating East Rift tip, while the transtensional southern boundary remained approximately fixed. Some time after 2.5 Ma northward migration of the East Rift stopped and the Southwest Rift began to open up along the transtensional Pacific-Easter transform zone. Compression occurred along the northern boundary as the Nazca plate was thrust over the microplate.
Hagen et al. (1990) suggested that this microplate was initiated by a mantle plume, though this is not part of the standard plume hypothesis and microplates do not exist at most other proposed plume localities. The Nazca plate itself exhibits further complexities, including relict microplates and extinct spreading centres (Ray et al., 2012).
Other suggestions for the origin of the volcanism that built the Easter - Sala y Gomez chain include a "hot line" (Bonatti & Harrison, 1976; Bonatti et al., 1977), a leaky fracture zone (Herron, 1972; Clark & Dymond, 1977), an early stage spreading center (Mammerickx, 1981) and a zone of lithospheric extension (Mammerickx & Sandwell, 1986). Detailed bathymetric mapping of part of the volcanic zone that is ~ 1,350 km long including the southern part of the Easter microplate and Easter and Saly y Gomez islands has shown that volcanism is widespread, the distribution of the edifices is not linear and some of the volcanic ridges are not subparallel. There are over 3,000 separate volcanic centres (Liu & Naar, 1997a) and widespread volcanic flows occur over large areas of the seafloor that are unlike any other volcanic chain surveyed on the seafloor (Liu & Naar, 1997b). The effective lithospheric elastic thickness along the chain, estimated by modellling gravity data is ~1-4 km (Kruse et al., 1997). This is quite thin and is consistent with a young seafloor age at the time of loading which could result from thermal weakening.
Age dating
Ray et al. (2012) report 40Ar/39Ar incremental-heating age determinations for basalts dredged from the ridge east of Salas y Gomez Island, including the Nazca ridge. They report that, with some exceptions, ages increase from 1.4 to 30 Ma toward the east (Figure 2). From this they conclude that most of the volcanoes erupted onto seafloor 5 - 13 Ma in age (Figure 3).
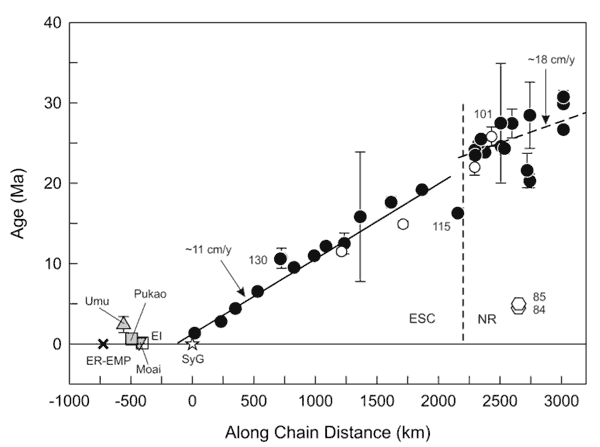
Figure 2: 40Ar/39Ar ages from the ridges east of Easter Island vs. along-chain distance from Salas y Gomez. Error bars indicate 2σ uncertainties on age (from Ray et al., 2012).
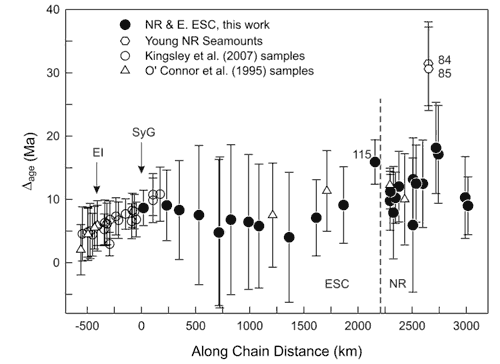
Figure 3: Estimated age of sub-seamount crust at the time of seamount lava eruption vs. along-chain distance of the sampling site from Sala y Gomez Island (from Ray et al., 2012).
Topography
Around the Sala y Gomez Ridge are shallow volcanic belts and two distinct constructional ridges with shallow summits rising to depths of just 1500 m. These are thought to be relatively recent constructional features. The western end of the ridge has an average ocean floor depth of 2,800 m (Stoffers et al., 1994). The zone of high topography lies approximately parallel to the direction of relative motion between the Pacific and Nazca plates. The islands and seamounts themselves are scattered. The width of the entire zone, at over 400 km, is much larger than that of the Hawaiian and French Polynesian lineaments (Clarke & Dymond, 1977).
Maia & Diament (1991) report a large anomalous topographic feature around Easter Island (Figure 4). A map of geoid roughness (Figure 5) shows the Easter-Sala y Gomez ridge is a continuous band comprising a high concentration of topographically elevated features such as seamounts (Maia & Diament, 1991).
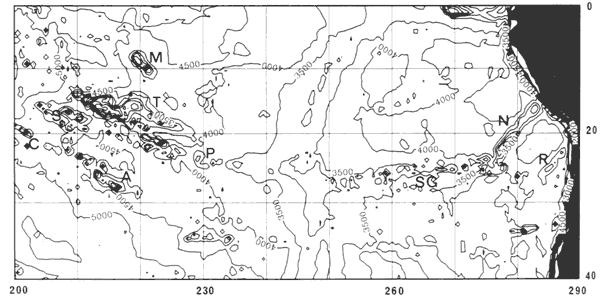
Figure 4: Bathymetry of the Easter Island region. Contour interval is 500 m. A = Austral Archipelago; C = Southern Cook Islands; M = Marquesas Archipelago; S = Society Islands; T = Tuamotu Archipelago and Plateau; P = Pitcairn-Gloucester alignment; SG = Easter-Sala y Gomez chain; N = Nazca Ridge; R = 25°S ridge. SG: Easter - Sala y Gomez chain (Maia & Diament, 1991).
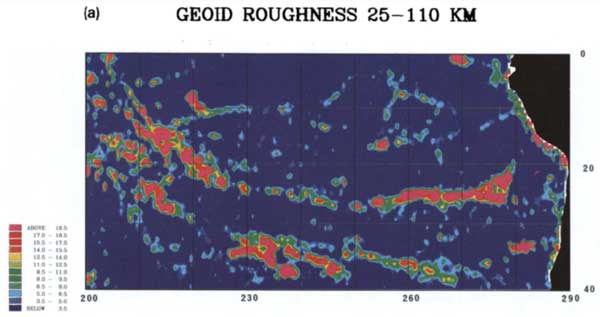
Figure 5: Geoid roughness for the 25-110 km waveband. (Maia & Diament, 1991).
Petrology and geochemistry
Easter Island is the product of three principal volcanic centres specifically Teravaka, Poike and Rano Kau. These have K-Ar ages of 3 - 0.3 Ma (Figure 1; Baker et al., 1974). The main rock types are olivine basalts and basaltic hawaiites (Figure 6). The basalts generally contain high total iron, low MgO and relatively low potassium. Na2O predominates over K2O (Baker et al., 1974, Clark & Dymond, 1977). Silica is unusually low, around 43%, with the basalts straddling the normative plane of critical silica undersaturation but lying mainly within the fields of quartz and olivine tholeiites (Baker et al., 1974).
The older end of the chain is not associated with any known initial flood basalt as is expected in the plume-head model (see The case for mantle plumes for a discussion).
Figure 6: Relative proportions of rock types from Easter Island, based on 200 samples. B: basalt, H: hawaiite, M: mugearite, Be: benmoreite, T: trachyte, R: rhyolite (from Baker et al., 1974).
Ray et al. (2012) report a lack of any time-progressive geochemical gradient along the ridge east of Salas y Gomez. Glisovic & Forte (2014), however, report that the province exhibits geochemical asymmetry with Pb, Sr and Nd isotope analysis indicating greater enrichment in the NE half of the Easter Island-Salas y Gomez ridge than the SW half. This has been interpreted in the context of the plume hypothesis to reflect structure within the plume conduit. Harpp et al. (2014) suggested bilaterally asymmetric compositional heterogeneity for the Galapagos Islands which Glisovic & Forte (2014) attributed to the geometry of geochemical reservoirs in the lower mantle (Figure 7). In the context of the Plate hypothesis, this would be interpreted as lateral variations in composition in the shallow upper mantle.
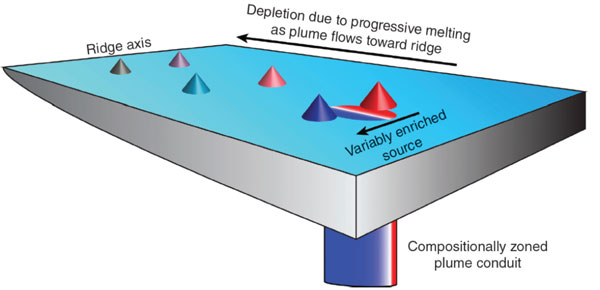
Figure 7: Schematic diagram of a plume model to explain the bilateral compositional symmetry of the Easter-Salas y Gomez volcanic ridge. The volcanism that built the ridge is attributed to an origin in the deep mantle with progressive depletion and mixing with melts derived from the depleted upper mantle during plume-ridge interaction. Despite such interaction the bilateral symmetry is preserved (from Harpp et al., 2014).
The geochemical signatures of the Easter and Galapagos systems are both more depleted than other Pacific volcanic systems. Harpp et al. (2014) suggest that, at the Galapagos, this results from plume-ridge interaction with the thinner, younger lithosphere underlying near-ridge plumes. In both the Plume and Plate hypotheses, thinner lithosphere, permitting shallower upwelling, would result in greater extents of melting, and this in turn might result in a greater percentage of the depleted MORB source in the compositions of the lavas.
Seismology
It has been proposed, from plate-motion reconstructions, that a low-velocity region at the core-mantle boundary (CMB) has underlain the EPR from 65 Ma to the present (Glisovic & Forte, 2014). The modelling approach assumed that the Pacific Large Low-Shear-Velocity Province (LLSVP) has remained stationary with respect to the CMB for this period. This assumption is difficult to test, however. Figure 8 shows the reconstruction at 5 Ma. With the assumptions used, the EPR lies directly above the eastern lobe of the Pacific LLVSP. These observations are consistent with a model whereby the EPR spreading centre was influenced by the LLSVP throughout the Cenozoic. It has been suggested that this drove flow across the CMB layer to the surface, replacing colder mantle hotter, less dense mantle. Numerical modelling suggested steep thermal gradients in the cold thermal boundary layer (Glisovic & Forte, 2014).
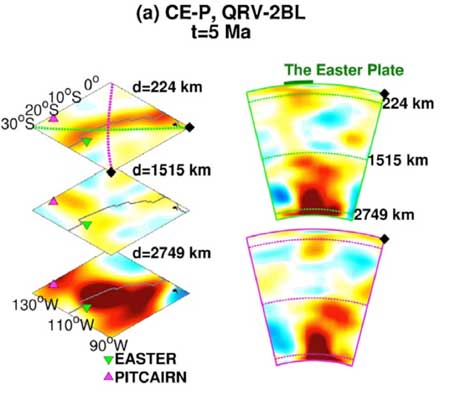
Figure 8: Seismic tomography structure under the EPR at 5 Ma. Green & magenta triangles: Easter Island & Pitcairn Island, respectively. Grey lines: plate boundaries. Red: low seismic velocities. Blue: high seismic velocities (from Glisovic & Forte, 2014).
Mantle heterogeneity beneath the EPR was estimated as part of this study and it was suggested a large-scale upwelling rises from the CMB to the surface beneath the Easter microplate. This was interpreted as a thermal plume, the shape of which varies with depth but which has constantly underlain the EPR for the last 65 Ma (Glisovic & Forte, 2014).
Montagner (2002) used seismology to detect EW-orientated channels of low velocity and anisotropy below Easter Island at depths of < 370 km and explored correlations with plate motion past and present. The results showed lateral variations on a scale exceeding 1000 km that are not predicted by plate tectonics. This suggested decoupling between the lithospheric plate and the asthenosphere. This could be related to plate cracking or secondary convection below a rigid lithosphere, and might indicate elevated temperatures (Figure 9). The low-velocity anomaly that rises to shallow depth at about 245°E this is an absolute longitude with zero at Greenwich, not E or W is assumed to be associated with seafloor spreading at the EPR. Figure 9 extends only to a depth of 350 km Comment: the caption says 370 km and so provides no information about greater depths.
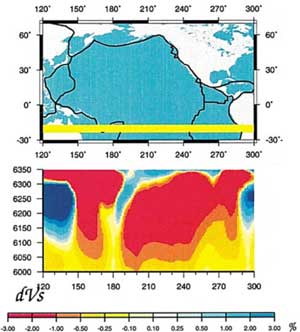
Figure 9: Vertical cross-section showing Vs anomalies beneath the Pacific Plate at 20°S from 20 to 370 km depth (from Montagner, 2014; lower panel vertical axis is labelled in kilometers above the CMB). Vertical exaggeration is ~ X 24, so most imaged features are much broader than they are tall.
Eruption Through the Easter-Sala y Gomez Fracture Zone
The entire volcanic ridge that stretches from the southern end of the Easter microplate to the western end of the Nazca Ridge (Figure 1) has erupted through and around an old fracture zone (Figure 10). The trace of this fracture zone is indicated by offsets in magnetic anomalies and can be seen continuing from the eastern end of the Easter chain, on into the Chile trench. Although very young basalts are found on Easter Island, the volcanic chain and its counterpart the Pitcairn-Gambier chain west of the EPR, clearly extend right up to the EPR.
It has been suggested that the volcanism results from transtension and "leakage" of melt from the mantle up through this permissive discontinuity (Menard & Atwater, 1968; Herron, 1972; Clark & Dymond, 1977). Fossil structures to the north, and a change in the sense of offset of the magnetic lineations between 7 and 5 Ma, are evidence that this fracture zone was re-activated in the past (Figure 1). The magnetic anomalies show that the fracture zone was right-lateral from 18 - 7 Ma (Figure 1) but has been left-lateral since 5 Ma, the approximate time when the Easter microplate began to form. It is not clear how it behaved from 5 - 7 Ma. Most of the Sala y Gomez Ridge formed in this "dark" period.
Other examples of roughly time-progressive volcanic ridges that have formed at fracture zones include the Lakshadweep-Chagos Ridge (postulated to be associated with a plume currently beneath Reunion Island) and the Ninety-East Ridge (postulated to be associated with a plume currently beneath Kerguelen Island), both in the Indian Ocean (see The Deccan beyond the plume hypothesis, Figure 5).
A modern Plate hypothesis for the Easter region would suggest that volcanism is associated with the tectonic disequilibrium that must characterise the southern end of the rotating Easter microplate (Schilling et al., 1985). Reconstructions of the tectonic history of the microplate show that its southern boundary has always beein in extension (Rusby & Searle, 1995). Extensional stress must extend throughout a region several hundred kilometers broad around the microplate. The relatively recent volcanism on Easter Island (~ 0.13 Ma; Baker et al. 1974) and older volcanism on Sala y Gomez (~ 1.9 - 1.3 Ma) suggest the region of extension that allows intraplate volcanism extends as far as 360 km from the EPR but is reduced at a distance of 750 km.
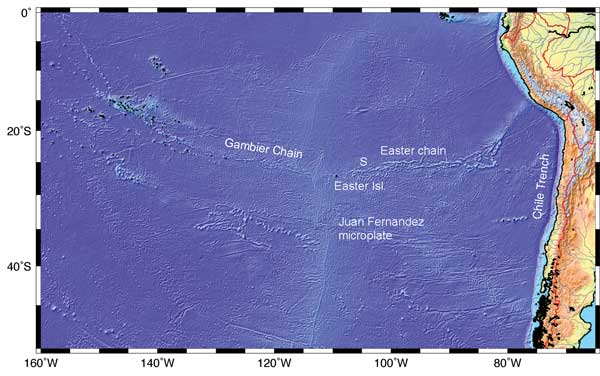
Figure 10: Bathymetry and topography in the region of the Easter - Sala y Gomez volcanic chain. S – Sala y Gomez Island. Basemap from Smith & Sandwell (1997). Figure from Foulger (2010).
Conclusions
Both Plume and Plate-based hypotheses have been suggested to explain the Easter - Sala y Gomez volcanic chain. Difficulties with the Plume hypothesis include:
- the absence of regional uplift and a flood basalt at the old (eastern) end of the volcanic chain, that might represent an arriving plume head;
- seismological work has not yet identified a plume-like structure extending from the surface down to the core-mantle boundary;
- high source temperatures for the volcanics have not yet been demonstrated.
Any model for the Easter - Sala y Gomez volcanic chain must explain the primary observables from the region. These include:
- the co-location of volcanism with a pre-existing fracture zone. This can only be explained as coincidence in the plume model;
- the mirror-image volcanic ridges that emanate to the west and the east from the EPR. It is unclear how this could be formed by a plume several hundred kilometers east of the EPR;
- the fact that these chains emerge from a position of tectonic extensional disequilibrium, i.e. the southern end of the rotating Easter microplate; and
- broadly comparable topographical features around the southern end of the Juan Fernandez microplate, 950 km further south on the EPR.
Table 1 summarizes the predictions and evidence for the two hypotheses.
Table 1: Predictions of and evidence for the Plate and Plume hypotheses.
Predictions |
Evidence |
Plume hypothesis |
Precursory uplift |
High topography at old end of chain, but not clear if related |
Flood basalt |
No |
Tail to CMB |
No, though seismic anomaly at CMB |
High temperatures |
No data |
Time progressive chain of volcanism |
To some extent |
Plate hypothesis |
Volcanism relating to lithosphere extension |
Yes. Extension at the EPR and along "leaky" fracture zone. |
Space/time distribution follows extension |
Yes, if region of strongest extension remained in the broad area around the EPR. |
Shallow mantle structure |
Yes |
Normal temperatures |
No data |
Melt exists in mantle |
Yes, in low-velocity zone |
References
-
Baker, P., Buckley, F. and Holland, J. (1974). Petrology and geochemistry of Easter Island. Contr. Min. Pet., 44(2), 85-100.
-
Bonatti, E., C. G. A. Harrison, D. E. Fisher, J. Honnorez, J. G. Schilling, J. J. Stipp, and M. Zentilli (1977), Easter volcanic chain (Southeast Pacific): A mantle hot line, J. Geophys. Res., 82, 2457-2478.
-
Bonatti, E., and C. G. A. Harrison (1976), Hot lines in the Earth's mantle, Nature, 263, 402-404.
-
Clark, J. and Dymond, J. (1977). Geochronology and petrochemistry of Easter and Sala y Gomez Islands: implications for the origin of the Sala y Gomez Ridge. J. Volc. Geo. Res., 2(1), 29-48.
-
Clouard, V. and Bonneville, A. (2001). How many Pacific hotspots are fed by deep-mantle plumes? Geology, 29(8), 695.
-
Foulger, G. R. (2010), Plates vs Plumes: A Geological Controversy, Wiley-Blackwell, Chichester, U.K., xii+328 pp.
-
Guillou, H., Garcia, M.O., and Turpin, L. (1997). Unspiked K-Ar dating of young volcanic rocks from Loihi and Pitcairn hot spot seamounts, J. Volc. Geotherm. Res., 78, 239-249.
-
Glisovic, P. and Forte, A. (2014). Reconstructing the Cenozoic evolution of the mantle: Implications for mantle plume dynamics under the Pacific and Indian plates. Earth Planet. Sci. Lett., 390, 146-156.
-
Haase, K. M., Devey, C. W. & Goldstein, S. L. (1996). Two-way exchange between the Easter mantle plume and the Easter microplate spreading axis, Nature 382, 344-346.
-
Hagen, R.A., N.A. Baker, D.R. Naar and R.N. Hey (1990), A SeaMarc II survey submarine volcanism near Easter Island, Mar. Geophys. Res, 12, 297-315.
-
Harpp, K., Mittelstaedt, E., Ozouville, N. and Graham, D. (2014). The Galápagos. American Geophysical Union, 27-39.
-
Herron, E.M. (1972). Sea-floor spreading and the Cenozoic history of the east-central Pacific. Geol. Soc. Am. Bull., 83, 1671-1692.
-
Hey, R. N., P. D. Johnson, F. Martinez, J. Korenaga, M. L. Somers, Q. J. Huggett, T. P. Le Bas, R. I. Rusby, and D. F. Naar (1995), Plate boundary reorganization at a large-offset, rapidly propagating rift, Nature, 378, 167-170.
-
Kruse, S. E., J. Z. Liu, D. F. Naar, and R. A. Duncan (1997), Effective elastic thickness of the lithosphere along the Easter seamount chain, J. Geophys. Res., 102, 27,305-27,317.
-
Liu, Z. J., and D. F. Naar (1997), Side-scan processing of GLORI-B and SeaBeam 2000, Mar. Geophys. Res., 19, 411-419.
-
Liu, Z. J., and D. F. Naar (1997), Swath bathymetry processing of GLORI-B and SeaBeam 2000, Mar. Geophys. Res., 19, 397-409.
-
Maia, M. and Diament, M. (1991). An analysis of the altimetric geoid in various wavebands in the Central Pacific Ocean: constraints on the origin of intraplate features. Tectonophysics, 190, 133-153.
-
Mammerickx, J. (1981). Depth anomalies in the Pacific: Active, fossil and precursor. Earth Planet. Sci. Lett., 53, 147-157.
-
Mammerickx, J. & Sandwell, D. (1986). Rifting of old oceanic lithosphere. J. Geophys. Res., 91, 1975-1988.
-
Menard, H.W. and Atwater, T., (1968). Changes in direction of sea-floor spreading, Nature, 219, 463-467.
-
Montagner, J. P., (2002). Upper mantle low anisotropy channels below the Pacific Plate. Earth Planet. Sci. Lett., 202(2), 263-274.
-
Montelli, R., G. Nolet, F. Dahlen, and G. Masters (2006), A catalogue of deep mantle plumes: new results from finite-frequency tomography, Geochem. Geophys. Geosys., 7, doi:10.1029/2006GC001248.
-
Morgan, W. J. (1971). Convection plumes in the lower mantle, Nature, 230, 42-43.
-
Müller, R. D., Sdrolias, M., Gaina, C. & Roest, W. R. (2008). Age, spreading rates and spreading symmetry of the world’s ocean crust, Geochem. Geophys. Geosys., 9, Q04006, doi:10.1029/ 2007GC001743.
-
Naar, D.F., and R.N. Hey, Tectonic evolution of the Easter microplate, J. Geophys. Res. 96, 7961-7993, 1991.
-
O'Connor, J., Stoffers, P. and McWilliams, M. (1995). Time-space mapping of Easter Chain volcanism, Earth Planet. Sci. Lett., 136(3-4), 197-212.
-
Ray, J. S., J. J. Mahoney, R. A. Duncan, J. Ray, P. Wessel, and D. F. Naar (2012), Chronology and geochemistry of lavas from the Nazca Ridge and Easter Seamount Chain: a ~30 Myr hotspot record, J. Pet., 53, 1417-1448.
-
Rusby, R. I., and R. C. Searle (1995), A history of the Easter microplate, 5.25 Ma to present, J. Geophys. Res., 100(B7), 12617–12640, doi:10.1029/94JB02779.
-
Schilling, J.-G., H. Sigurdsson, A. N. Davis, and R. N. Hey (1985), Easter microplate evolution, Nature, 317, 325-331.
-
Smith, W. H. F., and D. T. Sandwell (1997), Global sea floor topography from satellite altimetry and ship depth soundings, Science, 277, 1957–1962, doi: 10.1126/science.277.5334.1956.
-
Stoffers, P., Hékinian, R. and Haase, K. (1994). Geology of young submarine volcanoes west of Easter Island, Southeast Pacific, Mar. Geol., 118(3-4), 177-185.
last updated 17th January, 2017 |