Eruptive
Temperatures of the Paleogene Picrites of West
Greenland and Baffin Bay
|
|
James
H. Natland
Rosenstiel School
of Marine and Atmospheric Science, University of Miami,
Miami, FL 33149
jnatland@rsmas.miami.edu
Click here to
download a PDF version of this webpage
Abstract
Tholeiitic picrites from West
Greenland and Padloping Island on either side of
Baffin Bay have long been considered to be high-temperature
eruptives from the ancestral Iceland mantle plume.
Olivine-liquid FeO-MgO equilibria have been used
to backtrack to calculated parental compositions,
giving as much as 20.2% MgO in the melt, and to estimate
eruptive temperatures for them of close to 1500°C.
Crystallization histories, however, indicate that
these picrites are hybrids between olivine-charged
crystal sludges and differentiated magmas that followed
a low-pressure olivine-plagioclase-clinopyroxene
cotectic during differentiation. The glasses give
eruptive T = 1170-1220°C. Much of the olivine
in the rocks, especially the very magnesian olivine
(Fo92-93),
was incorporated by magma mixing and likely scavenging
of minerals from mantle wall rock. The rocks are thus
hybrids and the olivine in them neither lies along
a single olivine-controlled liquid line of descent,
nor can it be linked to the host compositions, which
are too high in iron. Therefore they are unsuitable
for the backtrack procedure. Compositions of Cr-spinel
support a mixing history for the picrites, but the
procedure of Poustovetov & Roeder (2000)
suggests temperatures of spinel crystallization no
higher than about 1340°C, generally less than 1300°C,
and MgO contents no higher than ~16% in the most magnesian
parental melt strains.
Introduction
I have applied criteria for identification
of material suitable for calculation of eruptive and
potential temperatures to Paleogene picrites of West
Greenland and Padloping Island on either side of Baffin
Bay (Francis,
1985; Robillard et al., 1992; Pedersen & Larsen,
2000; Yaxley et al., 2002). The locations
are at 65°N and 71°N on the eastern and western
coasts of Baffin Bay, respectively (Figure 1). Robillard
et al. (1992), Larsen & Pedersen (2002)
and Yaxley et al. (2002), as well as earlier
workers, attribute picrite eruption to the influence
of a high-temperature ancestral Iceland plume. The
last two of these studies, plus Herzberg & O’Hara (2002),
used olivine-melt MgO-FeO relationships to conclude
that the rocks are the hottest basalts of a plume head
that impacted North America at some 60 Ma, the tail
of which now is supposed to underlie Iceland. Altogether,
no studies other than those of Baffin Bay picrites
provide so adequate a data set, including compositions
of bulk-rocks, glass, melt inclusions, olivine, and
spinel, with which to judge the question of temperature
associated with this postulated plume.
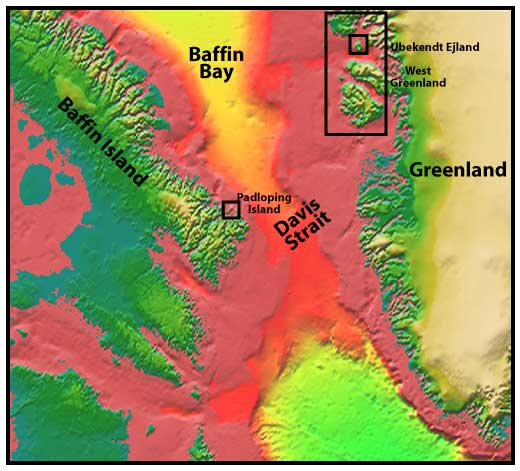
Figure 1. Location
of localities around Baffin Bay mentioned in
the text. West
Greenland includes samples of Larsen & Pedersen
(2000) and Graham et al. (1998). Padloping
Island includes samples of Francis (1985), Robillard
et al. (1992) and Yaxley et al. (2002). Ubekent
Ejland is the location of xenoliths of Bernstein
et al. (2006). |
An unusual number of basalts on both
sides of Baffin Bay are indeed picrites, with up to
29.8% MgO content in the bulk rock. They contain crystals
of olivine as magnesian as Fo92.9 that are both euhedral
and normally zoned, and the olivine also occludes magmatic
spinel. The minerals occur in many specimens and thus
are not a special case. The abundance of so many picrites
and the presence in some of them of strongly forsteritic
olivine has long lent the aura of heat to petrological
interpretations of this region (starting with Clarke,
1970 and Clarke & O’Hara, 1979).
The question is, how hot was it?
Petrological Backdrop
Following Natland (2007),
basaltic glass in Baffin Bay picrite and associated
basalt, analyses of which are plotted in Figure 2,
strongly resembles glass found in Iceland in that it
comprises tholeiitic low-Ti basalt (parental TiO2 at
MgO = 8% = Ti8 < 1.4%), tholeiitic
higher-Ti basalt and, in some formation members, nepheline-normative
alkali olivine basalt (Larsen
et al., 2003). The stratigraphy also includes
silicic lava or tuff (mainly dacite and rhyolite).
In some studies, the tholeiitic higher-Ti basalt is
termed E-MORB. The rocks are also similar to those
of Iceland chemically, showing no “global trends” among
variable Na8, Fe8 and Ti8 (oxide
values corrected for differentiation to a nominal near-parental
value of MgO = 8%; Klein & Langmuir, 1987).
As at Iceland, the rocks are not, as MORB might be,
related by simple variations in extent of partial melting
of a homogeneous mantle.
Somewhat surprisingly, no glass has
Ti8 <1.2%, whereas many primitive Icelandic
tholeiites have Ti8 lower than 1%, reaching
as low as 0.5% (Natland, 2007). Glass in tholeiitic
basalt and picrite from Padloping Island has lower
TiO2 and somewhat higher SiO2 and
MgO than in basalt from west Greenland. Another surprise,
however, is that Baffin Bay tholeiitic basalt and picrite
have low ΔNb (Fitton et al., 1997), with
most low-Ti8 basalts and picrites, dacite
and rhyolite having negative ΔNb (by definition,
less than Iceland), and most higher-Ti8 basalt
and picrite having positive ΔNb. Assimilation
of silicic material by basalt, or basalt-rhyolite mixing,
could explain the wide ranges in Nb/Y and Zr/Y and
low ΔNb of many of the rocks (e.g., Natland,
2007). Graham et al. (1998) also consider
that high 3He/4He (R/Ra), (up
to values of 30.6) in crushed olivine taken from West
Greenland picrite is evidence for a mantle plume. However,
the literature abounds in interpretations of geochemistry
invoking crustal contamination or derivation of some
fraction of the basalts by partial melting of ancient,
previously melted continental lithosphere. Is very
forsteritic olivine, unusually abundant in these rocks,
and perhaps also the high 3He/4He
(R/Ra), which might be contained in fluid inclusions
within it, a relic of an ancient melting event?
Glasses are Cotectic Liquid Compositions
with Olivine Xenocrysts
For evaluation of temperature, the
most important aspect of analyzed glass on either side
of Baffin Bay (Francis, 1985; Robillard
et al., 1992; Pedersen & Larsen,
2000) is that none of it is olivine-controlled (Figure
2).
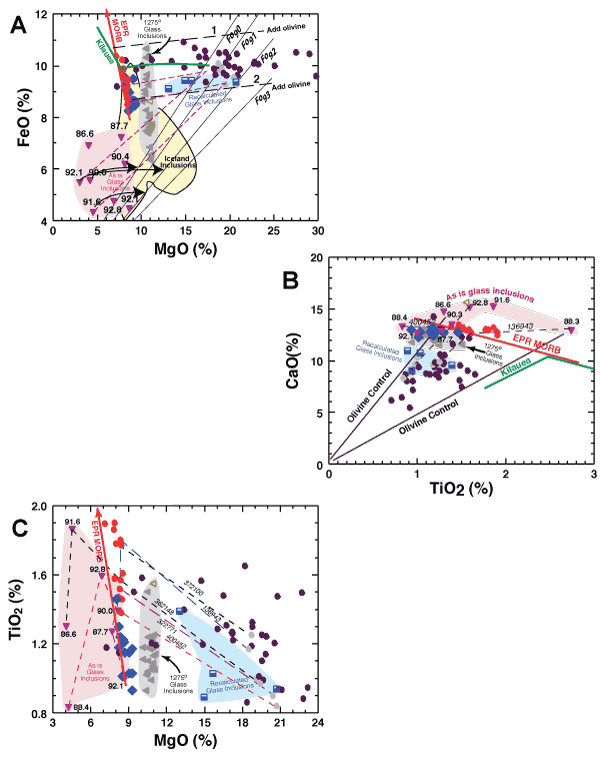
Figure 2: Compositions
of glass, bulk rocks, and melt inclusions from
West Greenland and Padloping Island. A. MgO
versus FeO, where FeO =0.9 X FeOTOTAL. B. TiO2 versus
CaO. C. MgO versus TiO2. Data sources
are West Greenland - Larsen & Pedersen
(2002); Padloping Island - Francis (1985),
Robillard et al. (1992), and Yaxley et al.
(2002). Trends for East Pacific Rise (EPR)
MORB glasses are regressions for analyses from
the Smithsonian
glass group data set ( (Magma Batch File, version
100199). Trends for Kilauea glasses are
regressions for olivine-controlled (flat) and
cotectic (steep) differentiation sequences,
from Clague et al. (1995). Add olivine control
lines 1 and 2 in A are drawn at upper and lower
bounds on bulk rock compositions but with olivine-added
trends using average olivine compositions in
the samples; these are olivine accumulation,
not liquid trends. Symbols in all diagrams
are as follows. Glasses are: red circles – West
Greenland; blue circles: Padloping Island.
Whole rocks are: West Greenland – black
and gray circles, with gray circles containing
analyzed melt inclusions. Melt inclusions from
West Greenland, as analyzed (as-is), are inverted
maroon triangles. Next to these are forsterite
contents (Fo) of adjacent olivine, and variously
dashed and colored lines connect them to their
host glass and bulk-rock compositions (given
by sample number in C). Glass inclusions from
Padloping Island are: from experiments at 1275°C – left-pointing
gray triangles and one open triangle, with
higher CaO and lower Na2O and SiO2 (see
text); selected inclusions recalculated to
have MgO > 12% - half-filled blue squares.
Inclusions are also enclosed in fields of different
colors: West Greenland = light purple; Padloping
Island – 1275°C = light gray; Padloping
Island – recalculated = light blue. In
A, the data field for Iceland glass inclusions
is from Foulger
et al. (2005).
A also shows isopleths for MgO and FeO content
in equilibrium with olivine of compositions
from Fo90 to Fo93, from
Herzberg
& O’Hara (2002). Arrowhead tips of the curving
arrows give estimated as-is glass inclusion compositions
in host olivines, interpolated to the olivine isopleth
compositions. Click here or
on Figure for enlargement. |
Glass compositions fall along a low-pressure
plagioclase-olivine-clinopyroxene cotectic similar
to that of depleted Pacific Mid-Ocean Ridge basalt
(MORB; red arrows in Figure 2), reaching ferrobasalt
compositions, and the glass margins of the rocks contain
both olivine and plagioclase microphenocrysts. The
Padloping Island lava contains dunite fragments and
broken crystals with kink bands. Francis (1985)
originally and accurately described the kink-banded
olivine as xenocrysts, and these were the only olivine
crystals in his study with composition >Fo91.
Some of these very forsteritic crystals are normally
zoned to about Fo88, and have faceted outlines;
these are xenocrysts with overgrowths. Therefore all
these picrites are undeniably hybrid rocks.
Following the rationale developed for Samoa (Natland,
2008), backtrack calculations of Larsen & Pedersen (2000)
to estimate ultimate parental MgO content and eruptive
temperature cannot be correct when applied to cotectic
glass compositions. Estimated parental MgO contents
are too high as are estimates of crystallization and
potential temperatures because the host glasses are
iron enriched by crystallization along the low-pressure
cotectic. Assumption of olivine control thus gives
calculated parental MgO contents that are too high
for melt MgNo (= Mg/[Mg+Fe2+]) adequate to be in equilibrium
with Fo91-93 olivine in mantle sources.
Melt Inclusions
Larsen & Pedersen (2002)
determined compositions of melt inclusions in very
forsteritic olivine. These systematically have lower
FeO, lower MgO and higher CaO than host glasses (Figure
2). The most forsteritic olivine, which is by no means
the most abundant olivine in the rocks, contributes
to olivine-addition, as indicated by tie lines between
glass and corresponding whole rock compositions. The
inclusions contribute to the notion that the forsteritic
olivine in which they reside is foreign to their host
basalt, not lying along a common liquid line of descent
with other olivine, nor linked to the host glass first
by olivine-controlled and then by cotectic crystallization;
this is why the rocks in general, whether taken as
glasses or whole rock compositions, are unsuitable
for backtrack calculations.
Yaxley et al. (2002) subjected
their specimens to reheating to 1275°C in Pt capsules
in order to re-equilibrate and homogenize partially
crystalline glass inclusions in olivine (gray triangles
in Figure 2). Considering that this experimental temperature
might still be too
low, they further adjusted the compositions of
melt inclusions to a fixed value of total iron as FeO
to take into account what they took to be post-crystallization
re-equilibration with the surrounding olivine. This
resulted in some of the glass inclusions having estimated
original MgO contents of 13-20.7% (half-filled blue
squares in Figure 2).
Considered as a group, however, the
cluster of high-MgO inclusions melted at 1275°C
does not fall along an olivine-controlled liquid line
of descent, but parallels and spans the natural cotectic
trend at somewhat higher MgO contents. All inclusions
originally along a single olivine-controlled liquid
line of descent would cluster tightly on this diagram. Yaxley
et al. (2002) describe the rocks subjected to
experiment as being “essentially identical” to
Padloping Island picrites of the earlier studies of Francis (1985)
and Robillard et al. (1992), which have low-pressure
cotectic glass compositions and both faceted plagioclase
and olivine in glass. The re-equilibrated inclusions
are thus most likely simply host glass in cross sections
of olivine embayments that actually have some of the
adjacent olivine melted into them at an experimental
temperature greater than that of cotectic
liquids (Figure 3). The experimental temperature is
thus too high to represent equilibrium for
all inclusions. Based on this and also petrography,
the compositions of liquids that hosted the phenocrysts
studied by Yaxley et al. (2002) are not olivine
controlled.
Using an original inference of Francis (1985)
about uniformity of bulk compositions across lava flows,
however, Yaxley et al. (2002) did not use
glasses, but instead determined whole-rock X-ray fluorescence
(XRF) analyses of quenched margins as the basis for
backtrack estimates. The XRF analyses of the margins
have variable MgO contents, from ~14-21%, at odds with
the glass analyses of earlier studies on rocks of identical
petrography. As whole rock rather than glass compositions,
these certainly contain variable amounts of olivine
phenocrysts, and the analyses should not be used for
backtrack calculations.
To summarize, as forsteritic as some
of the olivine in these rocks is, it only circumstantially
occurs in mixed rocks that may have had a number of
magma stems antecedent to the host rocks, and which
themselves were substantially differentiated (on a
low-pressure cotectic) when olivine incorporation occurred.
The crystallization and mixing histories thus are very
like those of Hawaiian and Juan Fernandez picrite (Helz,
1987; Clague et al., 1995; Natland,
2003). Dikes or other intrusions of differentiated
magma intersected crystal sludges – stagnant
masses of some other magma at depth; these were charged
with olivine phenocrysts and xenocrysts. The intruding
dikes also scavenged some olivine and spinel from wall
rock. Portions of all these materials were necessary
to construct each final picrite. The pre-cotectic liquid
line of descent of any given olivine is unknown, there
may be more than one line of descent represented in
mixed lava, and the lines of descent cannot be reconstructed
using the crystallization history of the picrites.
Further Difficulties with Reconstructed Melt Inclusions
The reconstruction procedure of Yaxley
et al. (2002)
forces the compositions of the melt inclusions usually
to higher but sometimes lower FeO than the simple experimentally
re-equilibrated melt inclusions happened to have. The
experimental melt inclusions have a restricted range
of MgO contents, from 10.1-11.4%, as befits melts quenched
at a single temperature of 1275°C, and the group
of them is not olivine-controlled (Figure 2). This
is in accord with quenching of experimental charges
equilibrated at a single temperature. Additional reconstruction
calculations stretch out the range of MgO contents,
to 9.7-20.2%, and in particular force many inclusions
to have high estimated original MgO contents (Figure
2A). This also provides a very crude and partial, but
I would say only apparent, degree of olivine-control
to the range of melt-inclusion compositions (light
blue fields in Figure 2), seemingly justifying the
conclusion that some parental liquid stems must have
had very high MgO contents.
However, three assumptions are involved. The first
is that re-equilibration at 1275°C was at too low a
temperature simply because the FeO content seems
too low when compared with whole-rock compositions;
the second is that low-FeO inclusions must automatically
have experienced Fe loss to surrounding olivine; the
third is that an ancient continental lithospheric mantle
source of unknown diversity, but which clearly provided
a continental lithospheric geochemical signal to the
picrites (Yaxley et al., 2002), provided no
low-FeO magma stems to the hybrid state of these rocks,
as they more obviously did to the West Greenland samples
studied by Larsen & Pedersen (2002). All
three assumptions can be questioned, but all point
in the same direction. The surest way ultimately to
infer a high-temperature picritic composition is to
add iron that was not measured back in to the original
inclusion compositions, and then “correct” the
MgO contents accordingly.
The suitability of melt inclusions
for backtrack calculations in general can be questioned
for two reasons. First, some inclusions may have been
captured from boundary layers adjacent to growing olivine
crystals and from which some elements excluded from
the growing olivine might have become concentrated
(Natland, 2003).
The width of a boundary layer depends on cooling rate
(Gaetani & Watson, 2000) and heightened
concentrations of a variety of trace and minor elements
might have occurred. Interpretations of, for example,
the extent and type of enrichment of mantle sources
(e.g., Yaxley et al., 2002) thus
might be compromised.
Second, backtrack calculations may
be compromised by high diffusion rates of FeO and MgO
across olivine and into inclusions after mixing into
a foreign magma (Gaetani & Watson, 2000).
In numerical simulations, inclusion compositions in
olivine tholeiite from the Reykjanes peninsula, Iceland,
were modified in a matter of months. That is they were
modified at rates comparable to residence times in
magma chambers and conduits, to become similar to those
of host liquids, with somewhat lower MgO and distinctly
higher FeO contents, depending on how differentiated
the new host liquid happened to be. Thus mixing of
primitive magmas into differentiated cotectic liquids
tended to make inclusion compositions more iron
rich than they were initially, adding to the potential
to calculate parental crystallization temperatures
that are too high. Furthermore, the final liquid compositions
are more sensitive to changing temperature than olivine
compositions; indeed, the effect on olivine compositions
was minimal. Mixing throws melt inclusions out of equilibrium
with host olivine, an effect that is particularly marked
in the data of Larsen & Pedersen (2002).
Attempts by Gaetani & Watson (2000) to
simulate addition of olivine back into melt inclusion
compositions and then to follow the cooling history
of the olivine failed to produce liquid lines of descent
agreeing with the olivine addition path. They state “This
mismatch demonstrates the inherent potential for uncertainty
in melt inclusion correction calculations due to diffusive
exchange between an inclusion and its host olivine
during the later stages of cooling” (p.
35).
If these problems are ignored, the
melt inclusions in olivine of composition Fo90-92 of Larsen & Pedersen (2002)
with iron as measured give approximate maximum backtracked
parental MgO contents of as little as 10-12% (lower
curving arrows in Figure 2A). Sigurdsson et al. (2000)
obtained similar values for inclusions in Iceland spinel
without adjusting FeO contents. This is about the MgO
content calculated for the most primitive MORB, which
the suite otherwise resembles (e.g., it has
low TiO2 contents and nearly matches MORB
cotectic differentiation). This backtrack estimate
only can be attempted assuming that the inclusions
were not also crystallizing plagioclase or clinopyroxene
at any point, which is unlikely based on the cotectic
trend of host glasses and the presence of similar glasses
at Iceland. One inclusion has CaO higher (open triangle
in Figure 2B), and both SiO2 and Na2O
lower, than the others, suggesting that it may have
included calcic plagioclase before heating to 1275°C.
Compositionally similar low-FeO inclusions at Iceland
occur not just in olivine as magnesian as Fo92,
but also in Cr-spinel, clinopyroxene and plagioclase
in the same rocks (e.g.,
Gurenko & Chaussidon, 1991; Sigurdsson
et al., 2000). They cannot all be corrected for
iron loss in the same way as inclusions in olivine,
thus a simple olivine-control correction is inadequate.
The minerals therefore crystallized from liquids represented
by their associated melt inclusions with their current
compositions at temperatures of about 1300°C, based
on experimental comparisons (see also Foulger
et al.,
2005, who estimated similar temperatures
using the glass geothermometer of Beattie,
1993). Thus many West Greenland and Icelandic melt
inclusions have low FeO and also low MgO; this gives
the low crystallization temperatures at glass MgNo
= 67-78. The Padloping Island picrites are similar
in most respects of composition and mineralogy, but
do not have low-FeO melt inclusions. Instead, on all
variation diagrams, almost all the Padloping inclusions
are similar to host glasses (but with MgO systematically
elevated by experiment); they may not have been inclusions
at all, but cross sections of open skeletal embayments
in the olivine.
Olivine in picrite thus might have
many origins, including some crystals that might be
from ancient dunite cumulates or fragments of refractory
upper mantle residues either of a modern or an ancient
partial melting event. Olivine as magnesian as Fo92-93 especially
could be xenocrystic, since this is evidently the
predominant global composition of olivine in ultramafic
xenoliths derived from Archean mantle, including Ubekendt
Ejland, West Greenland (Bernstein
et al., 2006, 2007), which is within the West
Greenland picrite province (Figure 1). Such olivine
is interpreted to be in residual ultramafic rock left
from an Archaean partial melting event. Mafic liquid
in equilibrium with this olivine during the ancient
partial melting event could have precipitated magmatic
olivine just as magnesian. That olivine may then and
there have precipitated in dunitic veins, pods and
tabular magma bodies between melt sources and the Archaean
crust, and eons later been extracted by dike rocks
of differentiated Paleogene magmas. Whether this is
likely or unlikely is difficult to say. However, could
it really be a coincidence that such magnesian olivine
just happens to occur in the lithosphere where “plume
head” picrite
erupts? At the minimum, simple existence
of Fo92-93 olivine in West Greenland and
Baffin Bay picrites cannot be absolute proof that host
basalt had a high-temperature precursor along any thread
of a common liquid line of descent in Paleogene times.
Some other means of assessment is needed.
Spinel
Cr-spinel is a mineral of great petrological sensitivity,
a ready indicator of aspects of magma composition and
oxidation state (Allan et al., 1988; Poustovetov & Roeder, 2000).
Magmatic spinel is almost always euhedral and is most
often enclosed in olivine, which it thus appears to
precede in crystallization sequence. This can be tricky
to judge, however, since olivine growth may trigger
Cr saturation in the melt immediately next to it (c.f., Natland,
2003). The two minerals thus crystallize mainly along
a two-phase cotectic, but during the very earliest
crystallization history of any primitive basalt. We
tend to think of olivine as being the first mineral
to crystallize from basalt, but this is not technically
correct. Dunite, ostensibly the cumulate produced during
this stage, is never a “monomineralic” rock.
Poustovetov & Roeder (2000)
developed a model from which the composition of chromite
can be predicted from melt (glass) compositions. Their
program is available as an Excel spreadsheet, Melt-Chromite.
Spinel composition involves coupled substitutions between
Fe2+ and Al, and between Mg and Cr. Ti also
partitions into spinel. In the structural formula for
spinel, proportions of these five elements as well
as minor elements that substitute for them allow Fe3+ to
be calculated from charge balance. For the crystallization
model, however, the ratio of Fe2+/(Fe2++
Fe3+) in the
glass must be assumed. For initial calculations and
comparisons, I have taken this ratio to be 0.9, a common
value for MORB (Christie et al., 1986). I
thus compare predicted spinel compositions for basalt
glasses and either natural or experimental melt inclusions
of samples from West Greenland and Padloping Island
(Figure 3). The natural spinel compositions are in
the left panels (Figure 3A, C, and E); predicted compositions
are in the right three panels (Figure 3B, D, and F).
The diagrams for CrNo (Cr/[Cr+Al]) and Fe3No
(Fe3+/[Fe3++Al+
Cr + V]) versus MgNo (Mg/[Fe2+ + Mg]) are
conventional faces for the spinel prism. TiO2 (rather
than TiNo) is plotted semilogrithmically against MgNo
in the lower two panels.
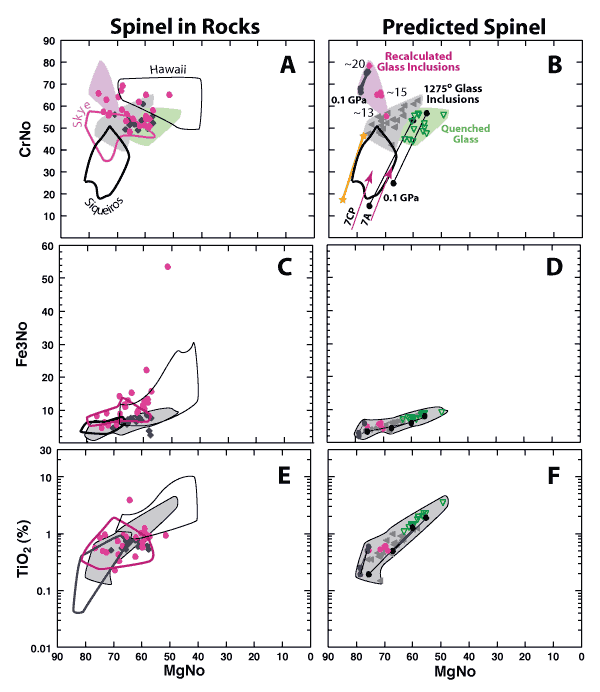
Figure 3: Comparison
of natural spinel in West Greenland and Padloping
Island picrites (left panels, A, C, and E)
with spinel predicted to be in natural glasses
and glass inclusions (right panels, B, D, and
F) by the technique of Poustovetov & Roeder
(2000). Data sources are as in A, as are symbols
in left panels. Symbols in right panels are
given by labels to shaded fields which are
replicated without labels or symbols in A.
Data fields labeled in A by place are for picrites
and one plagioclase-phyric basalt from Siqueiros
fracture zone (Natland, 1989); Hawaii – Kilauea
and Puna Ridge (Clague et al., 1995); and Skye,
Scotland (J. Allan & J. Natland, unpublished
data). Gray fields in D and F bound full data
fields, rather than the distinct shaded fields
in B, and are repeated in C and E. Lines link
predicted selected spinel compositions calculated
at 1 bar and 0.1 GPa. These data points are
for natural glasses (black) and selected recalculated
and bulk rock parental inclusions with MgO
contents range from 13-20, as indicated near
the light purple data field in B (blue; Yaxley
et al., 2002). |
In Figure 3B, predicted spinel compositions
are given for quenched glasses, experimental glass
inclusions at 1275°C of Yaxley et al. (2002),
and various recalculated or estimated parental compositions
to a maximum MgO content of 20.2% (Yaxley et al.,
2002; Putirka, 2008). The data fields, respectively
green, gray, and lavender, are repeated in Figure 3A.
In the lower panels, I show just a single all encompassing
field (gray within a black line), which I replicate
in the right panels, Figure 3, C and E. For several
compositions, calculations at 1 bar and 10000 bars
(10 kilobars or 0.1 GPa) are linked by tie lines. The
predictions have the following features:
- Pressure increase produces strong variations
in CrNo (Figure 3B) with MgNo for glass compositions
with relatively low MgO contents;
- Pressure variations produce weak variations in
CrNo if glass has high MgO contents (Figure 3B);
- High glass MgO contents (13-20%) produce high
CrNo (Figure 3B);
- Cotectic crystallization causes CrNo to increase
somewhat, and MgNo to decrease (inclined light
green field in Figure 3B);
- Some spinel crystallized from glass with Fe2+/(Fe2++
Fe3+) < 0.9 (data points above gray
field in Figure 3C); adjusting this downward from
0.9 in glass used in the model calculations would
increase MgNo (by increasing melt MgNo at constant
total iron) and CrNo in predicted spinel compositions.
The general result is that high glass
MgO-contents (>15%) produce a restricted predicted
field (lavender) on Figure 3B, one fairly insensitive
to pressure, with spinel compositions having both high
MgNo and high CrNo. Most of this field does not overlap
natural spinel from West Greenland and Padloping Island,
nor the boundaries of three other data fields provided
for comparison (Hawaii, Skye, and Siqueiros Fracture
Zone in the eastern Pacific). Predicted individual
spinel compositions within the lavender field of Figure
3B, also have lower Fe3No and TiO2 (purple
and blue data points linked by lines in Figure 3, D
and F) than natural spinel. Nearly horizontal trends
of spinel in Figure 3, A and E, result from mixing
between spinel-bearing high-MgO and low-MgO (differentiated)
magmas.
Poustovetov & Roeder (2000) suggested
that compositional variability in glasses, especially
in Al2O3 contents, could explain the variability of
MORB spinel, and especially invoked the role of plagioclase
fractionation in producing such variability. They thought
differences in glass Al2O3 content of as little as
2%, by weight (with Al2O3 varying from 15-17%) would
be sufficient to explain variability in CrNo. Since
many primitive MORB have abundant plagioclase phenocrysts,
this conclusion might be justified. However, the samples
from Siqueiros Fracture Zone are picritic, with no
plagioclase phenocrysts, thus the variability of spinel
CrNo there seems likely to indicate the effect of pressure.
All spinel at West Greenland and Padloping Island,
however, has fairly restricted and high CrNo, suggesting
that it all crystallized at shallow levels in the crust.
Spinel thus fairly positively indicates
that the compositions of the basaltic glass from which
it precipitated around Baffin Bay had MgO contents
no higher than ~15%. No natural spinel anywhere
matches compositions for any estimated parental basaltic
liquid with MgO content >18%,
as predicted for 26 out of 29 island locations by Putirka (2008).
Even the estimated parental glass for Siqueiros Fracture
Zone of Putirka (2008) seems too high
in MgO contents (13.2%) to explain the spinel variability
in picritic rocks there (Figure 3B). The entire spinel
field in Figure 3B is to the right of the estimated
parental spinel compositions, and crystallized from
melt with lower MgO content.
Summary
Figure 4 summarizes estimated predicted
temperatures of crystallization at atmospheric pressure
for compositions of glass margins and inclusions in
picritic samples from West Greenland. The temperature
algorithm is that of Beattie (1993), which
is available in the program Melt-Chromite of Poustovetov & Roeder (2000).
This gives temperature estimates a bit lower than the
scheme of Ford et al. (1993). For example,
the average temperature of inclusions heated experimentally
to 1275°C by Yaxley et al. (2002) is about
25° less than experimental with the Beattie procedure.
But all those compositions cluster tightly in both
T and MgO content on this diagram, as they should.
In contrast, five compositions recalculated from these
with higher MgO content project linearly to excessively
high T from compositions of natural glasses and the
homogenized inclusions. The maximum limit for MgO content
and temperature based on spinel is given by the dashed
outer boundary of the light blue field. The solid
line is a more realistic limit for spinel crystallization
at most places, including Samoa (Natland,
2008), Iceland and Padloping
Island, with only two West Greenland spinel compositions
falling outside it.
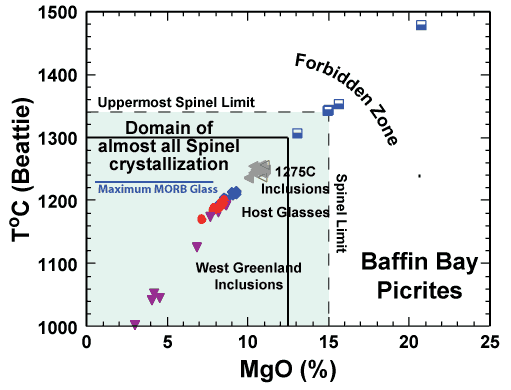
Figure 4: MgO content
of natural glasses and inclusions from West
Greenland and Padloping Island (data symbols
and sources as in Figure 2) versus estimated
temperature (ToC) of crystallization (Beattie,
1993), calculated from Melt-Chromite (Poustovetov
& Roeder, 2000). From Figure 3, the upper limits
of T and MgO content, representing the most magnesian
spinel composition, are given by the outer boundary
of the light blue shaded field (Spinel Limit). Also
from Figure 3, any more spinel in individual samples
crystallized from hybrid and/or more differentiated
liquids at <1300°C and 12.5% MgO (dashed line),
including a majority at temperatures and MgO contents
represented by host glasses. |
Spinel populations in given samples,
like those of olivine, appear to reflect a diversity
of parental compositions in their makeup (lateral spread
in MgNo at relatively fixed CrNo in Figure 3A), some
crystallizing from more magnesian melt strains, or
more differentiated liquids, or both, than others.
Host basalt is always an amalgam of many parental strains,
and is also influenced by mixing with differentiated
liquid. As a result, spinel compositions predicted
from glass margins will always plot further to the
right in Figure 3A than many natural spinel compositions
in the same samples. One can force a host composition
to some higher MgO content by adding olivine back into
it, but this diagram indicates how artificial that
procedure can be. Furthermore, such addition does not
produce a realistic antecedent composition from which
all olivine and spinel co-precipitated along a common
liquid line of descent, as Putirka (2008),
for example, assumed in his calculation of a parent
to Siqueiros picrite. The rocks say that this does
not occur, and that at best only some parental magma
strains anywhere had higher MgO contents than about
12.5%. In most rocks, the contributing melt strains,
and certainly the average of them, had MgO content
less than this.
Once again, the sample distribution
obeys the Central Limit Theorem (Anderson,
2003). A consequence is that we should not “correct” a
mean composition to what we consider to be an upper
statistical limit. Perhaps also the upper limit has
a plausible explanation, such as that the mantle source
is lithologically heterogeneous (Natland,
1989, 2007; Sobolev
et al., 2005) or that the more magnesian inclusion
compositions are a consequence of
pressure (Falloon
et al.,
2007a, b; Presnall & Gudfinnsson, 2008).
Then, even the statistical limit should not be subject
to correction. Thus a few strains in some places (e.g.,
Hawaii; Clague et al., 1995), but not all
(e.g., Samoa; Natland,
2008), might
have been olivine controlled and had 13-16% MgO contents,
but no higher, and they would have been no hotter if
erupted individually than about 1340°C (Figure
4). So say spinel compositions.
Discussion and Conclusion
One reviewer of an early draft of
this webpage advised me to keep the technique of olivine
addition to basaltic melt compositions as a means of
evaluating parental MgO content, eruptive temperature
and potential temperature. “Don’t
throw the baby out with the bath water,” he
advised. West Greenland and Padloping Island adjacent
to Baffin Bay seemed to offer the most likely challenge
to my view that picrites are complicated rocks; here,
if anywhere, the procedure was bound to work. However,
this region turns out to be no different than any other,
and the rocks are hybrids that are indeed too complicated
to see how the procedure can be valid even here. Some
other procedures, outlined above, are even less appropriate.
I have carefully searched the bath water, but find
no baby there to throw.
To recapitulate, the Paleogene picrites
of west Greenland and Baffin Bay were no hotter on
eruption than picrite at Iceland, and eruptive temperatures
at Iceland itself are little, at the very most about
100°, hotter than
a normal spreading ridge (Foulger
et al.,
2005; Presnall & Gudfinnsson, 2008).
This probably rules out a plume from the core-mantle
boundary by criteria of Putirka (2008), and may only
require variations in pressure or mantle heterogeneity,
rather than differences in temperature at a common
source depth, to explain. Although other places need
to be evaluated individually, this must be approached
through a careful examination of crystallization histories
of likely parental and near-parental compositions.
To date, I have not found any rock from Samoa, Hawaii,
Juan Fernandez, West Greenland, Skye or Iceland in
which even any individual parental liquid magma strain
exceeded 16% MgO content, and the average MgO content
of hybrid and amalgamated host liquid was less than
that. The search for olivine-controlled glasses should
continue. If it is successful in future, it may modify
the present picture, but we have not reached this stage
yet.
The high Fo content of some Baffin
and West Greenland olivine, reaching Fo92 in
five samples and as high as Fo92.9 in one
of them, places unusual and restrictive conditions
on magma genesis. Fo92-93 is more magnesian
than average olivine in residual abyssal peridotite
from transform faults, even those near presumed hot
spots on ridge axes (Dick, 1989). Fo92-93
is more magnesian than olivine found in Samoan residual
harzburgite xenoliths, from which clinopyroxene has
been exhausted by partial melting (Wright,
1987; Dieu, 1995). In fact, Fo92-93
olivine is only found in the most refractory continental
peridotite xenoliths from which almost all orthopyroxene has
been extracted by melting and what is left in the melt
source is mainly dunite (Bernstein et al.,
2006, 2007). In experimental analogs to peridotite
considered with respect to partial melting, this is
equivalent to the invariant point enstatite-out, when
additional melt extraction ceases even as temperature
increases (e.g., Presnall, 1969).
In nature, Fo92-93 thus is likely the most
magnesian olivine in any peridotite in the lithosphere,
ancient or modern, but among peridotite xenoliths it only occurs,
so far as we know, in Archaean (not Proterozoic or
younger) mantle rocks so refractory that they may have
been involved in a widespread or global magma ocean
(Bernstein et al., 2007). The extent of partial
melting was likely 40% or more. Orthopyroxene exhaustion
in mantle sources thus is the minimum partial melting
condition required to produce a basaltic or picritic
partial melt capable of crystallizing >Fo92 olivine.
This is an extraordinary condition to impose on a
modern mantle plume, yet here it is postulated at a
place where Fo92-93 olivine happens to be prevalent
in the particular ancient lithospheric mantle through
which basaltic magma had to pass (Bernstein et
al., 2006). Backtracking liquid compositions to
the extent that they should be in equilibrium with
Fo92-93 thus implies that the Baffin plume head was
as hot as anything that has existed on the surface
of the Earth through geological time, that it ignored
the upper mantle through which its magmas had to ascend,
entraining none of it, and yet that it produced olivine
phenocrysts with the same composition as in only that
type of mantle. Furthermore that olivine now survives
only in variably enriched differentiated basalt so
little different from transitional to enriched abyssal
tholeiite or Icelandic basalt that even picritic calculated
parental compositions are only about 21% partial melts
(Putirka, 2007) rather than the 40% partial
melts entailed by the petrological condition. This
seems unlikely.
Finally, the high values of 3He/4He (R/Ra) in gases
taken from olivine in several West Greenland samples
(Graham et al., 1998) must be viewed with
caution. Bulk-rock compositions were not determined.
Rock descriptions in Graham et al. (1998)
indicate that olivine in some of the samples was as
magnesian as Fo92, although whether this was a particular
host to the high-3He/4He cannot be known. Even
if such radiogenic helium is present in less forsteritic
olivine, spread of “mantle” helium to highly
differentiated liquids in flanking rift systems, there
to work its way into olivine as iron rich as Fo75,
must be allowed (Natland, 2003). In this case,
the rocks have low concentrations of helium in general,
which is consistent with their olivine being picked
up when shallow degassed differentiates in dikes traversed
crystal-rich sludges or mantle wall rock. It also fits
with the evidence for shallow crystallization of spinel
in other rocks. The isotopic signal of helium thus
could well be dominated even by a minor concentration
of gases cracked out of ancient olivine-rich materials en
route. Thus if helium is present in very forsteritic
olivine in a picrite, then the source of that olivine,
especially if it has kink bands and subgrains, must
be carefully evaluated. The olivine is almost certainly
xenocrystic.
Olivine is a natural time capsule
for “old” helium
with high 3He/4He, and in cumulates
such as dunite, which are often deformed, helium isotopes
could retain original values without the influence
of U and Th, excluded from olivine, in the same facies,
for eons. One might simply claim that such helium arises
with plume magmas (e.g., Graham et al.,
1998). However, this follows from the assumptions that
the mantle is homogenous, and that all olivine in a
sample lies along a common liquid line of descent.
But when refractory Archaean mantle is traversed by
later basalt, one has to wonder whether a plume attribution
for this particular olivine, thence helium isotopes,
is justified.
Acknowledgments
For services rendered and thoughts expressed, not
necessarily in agreement with the above, I thank Trevor
Falloon, Dean Presnall and Gillian Foulger.
References
-
Allan, J.F., Sack, R.O., and Batiza, R., 1988. Cr-rich
spinels as petrogenetic indicators: MORB-type lavas
from the Lamont seamount chain, eastern Pacific, American
Mineralogist, 73, 741-753.
-
-
Beattie, P., 1993. Olivine-melt
and orthopyroxene-melt equilibria, Cont. Min.
Pet., 115,
103-111.
-
Bernstein, S., Hanghoj, K., Kelemen, P.B.,
and Brooks, C.K., 2006. Ultra-depleted, shallow
cratonic mantle beneath West Greenland: Dunitic
xenoliths from Ubekendt Ejland, Cont. Min.
Pet., 152,
335-347, doi:10.1016/S0012-821X(97)00175-I.
-
Bernstein,
S., Kelemen, P.B., and Hanghoj, K., 2007. Consistent
olivine Mg# in cratonic mantle reflects Archean
mantle melting to the exhaustion of orthopyroxene. Geology, 35,
4570462: doi:10.1130/G23336A.I.
-
Christie, D.M., Carmichael,
and Langmuir, C.H., 1986. Oxidation states of mid-ocean
ridge basalt glasses. Earth
Planet. Sci. Lett., 79,
397-411.
-
Clague, D.A., Moore, J.G., Dixon,
J.E., and Friesen, W.B., 1995. Petrology of submarine
lavas from Kilauea’s
Puna Ridge, Hawaii, J. Petrol., 36,
299-349.
-
Clarke, D.B., 1970. Tertiary basalts of Baffin
Bay: possible primary basalt from the mantle, Cont.
Min. Pet., 25, 203-224.
-
Clarke,
D.B., and O’Hara, M.J., 1979. Nickel
and the existence of high-MgO liquids in nature. Earth
Planet. Sci. Lett., 44, 153-158.
-
Dick,
H.J.B. 1989. Abyssal peridotites, very slow spreading
ridges, and ocean ridge magmatism. In Saunders,
A.D., Norry, M.J. (eds.), Magmatism in the ocean basins, Geological
Society Special Publlication 42, 71-105.
-
Dieu, J., 1995. On the formation of cumulates,
characteristics of oceanic lithosphere, and the
process of carbonatite metasomatism; a study of
ultramafic xenoliths from South Pacific Islands.
University of California, San Diego (Ph.D. Dissertation),
393 pp.
-
Falloon, T.J., Danyushevsky, L.V., Ariskin,
A., Green, D.H., and Ford, C.E., 2007a, The application
of olivine geothermometry to infer crystallization
temperatures of parental liquids: implications
for the temperatures of MORB magmas, Chem. Geol., 241,
207-233.
-
Falloon, T.H., Green, D.H. and Danyushevsky,
L.V., 2007b. Crystallization temperatures of tholeiitic
parental liquids: Implications for the existence
of thermally driven mantle plumes. In Foulger, G.R.,
and Jurdy, D.M. (eds.), Plates, Plumes, and Planetary
Processes.
Geological Society of America Special Paper 430,
235-252.
-
Fitton, J. G., Saunders, A.D.,
Norry, M.J., Hardarson, B.S., and Taylor, R.N.,
1997. Thermal and chemical structure of the Iceland
plume, Earth
Planet. Sci. Lett., 153,
197-208.
-
Foulger, G.R., J.H. Natland and D.L. Anderson,
2005. Genesis of the Iceland melt anomaly by plate
tectonic processes, in: Plates, Plumes,
and Paradigms, G.R.
Foulger, J.H. Natland, D.C. Presnall and D.L. Anderson
(Eds.), Geol. Soc. Am. Special Paper 388, 595-625.
-
Ford, C.E., Russell,
D.G., Craven, J.A., and Fisk, M.R.. 1983. Olivine-liquid
equilibria: Temperature, pressure, and composition
dependenc of the cystal/liquid cation protition
coefficients for Mg, Fe2+, Ca and Mn, J. Petrol., 24,
254-265.
-
Francis, D., 1985. The Baffin Bay lavas
and the value of picrites as analogues of primary
magmas, Cont.
Min. Pet., 89, 144-154.
-
Gaetani,
G.A., and Watson, E.B., 2000. Open system behavior
of olivine-hosted melt inclusions, Earth
Planet. Sci. Lett., 183,
27-41.
-
Graham, D.W., Larsen, L.M., Hanan, B.B.,
Storey, M., Pedersen, A.K., and Lupton, J.E., 1998.
Helium isotope composition of the early Iceland
mantle plume inferred from the Tertiary picriees
of West Greenland, Earth
Planet. Sci. Lett., 160,
241-255.
-
Gurenko, A.A., and Chaussidon, M., 1995.
Enriched and depleted primitive melts included
in olivine from Icelandic tholeiites: Origin by
continuous melting of a single mantle column, Geochim.
et Cosmochim. Acta, 59,
2905-2907, doi:10.1016/0016-7073(95)00184-0.
-
Helz,
R.T., 1987. Diverse olivine types in lava of the
1959 eruption of Kilauea volcano and their bearing
on eruption dynamics. In:Decker, R.W., Wright, T.L., & Stauffer,
P.H. (eds), Volcanism in Hawaii, U.S. Geological
Survey Professional Paper 1350. Washington:
U.S. Government Printing Office, 691-722.
-
Herzberg,
C. and O’Hara, M.J., 2002, Plume-associated
magmas of Phanerozoic age, J. Petrol., 43,
1857-1883.
-
Klein, E., and Langmuir, C.H., 1987. Global
correlations of ocean ridge basalt chemistry with
axial depth and crustal thickness, J. Geophys.
Res., 92,
8089-8115.
-
Larsen, L.M., and Pedersen, A.K, 2000.
Processes in high-Mg, high-T magmas: Evidence from
olivine, chromite and glass in Paleogene picrites
from West Greenland, J.
Petrol., 41, 1071-1098.
-
Larsen, L.M., Pedersen, A.K.,
Sundvoll, B., and Frei, R., 2003. Alkali
picrites formed by melting of old metasomatized
lithospheric mantle: Manitdlat Member, Vaigat Formation,
Paleocene of West Greenland. J. Petrol., 44,
3-38.
-
Natland,
J.H., 1989. Partial melting of a lithologically
heterogeneous mantle: Inferences from crystallization
histories of magnesian abyssal tholeiites from the
Siqueiros Fracture Zone. In: Saunders, A.D.
and Norry, M. (eds.), Magmatism in the Ocean
Basins, Geol. Soc. London, Spec. Publ., 42,
41-77.
-
Natland,
J.H., 2003. Capture of mantle helium by growing olivine
phenocrysts in picritic basalts from the Juan Fernandez
Islands, SE Pacific, J. Petrol. 44,
421-456.
-
Natland, J.H., 2007. ΔNb and
the role of magma mixing at the East Pacific Rise
and Iceland. In Foulger,
G.R., and Jurdy, D.M., eds., Plates, Plumes
and Planetary Processes, Geological Society
of America Special Paper 430: 413-449, doi: 10.1130/2007.20430(21).
-
-
O’Hara,
M.J., 1968. The bearing of phase equilibria studies
on the origin and evolution of igneous rocks, Earth-Science
Reviews, 4, 6-133.
-
Perfit,
M.R., Fornari, D.J., Ridley, W.I., Kirk, P.D.,
Casey, J., Kastens, K.A., Reynolds, J.R., Edwards,
M., Desonie, D., Shuster, R., and Paradis, S.,
1996. Recent volcanism in the Siqueiros ransform
fault: picritic basalts and implications for MORB
genesis, Earth
Planet. Sci. Lett., 141,
91-108.
-
Poustovetov, A., and Roeder, P.L., 2000.
The distribution of Cr between basaltic melt and
chromian spinel as an oxygen geobarometer, Canadian
Mineralogist, 39,
309-317.
-
Presnall, D.C., 1969. The geometrical analysis
of partial fusion. American Journal of Science, 267,
1178-1194.
-
Presnall, D. C., and Gudfinnsson,
G.H., 2008. Origin of oceanic lithosphere, J.
Petrol., 49,
615-632, doi:10.1093/petrology/egm052
-
Putirka, K.,
2008. Excess temperatures at ocean islands: Implications
for mantle layering and convection, Geology, 36,
283-286, doi: 10.1130/G24615A.
-
Putirka, K., Perfit,
M., Ryerson, F.J., and Jackson, M.G., 2007. Ambient
and excess mantle temperatures, olivine thermometry
and active vs. passive upwelling, Chem.
Geol., 241, 177-206, doi:
10.1016/j.chemgeo.2007.01.014.
-
Robillard, I., Francis,
D., and Ludden, J.N., 1992. The relationship between
E- and N-type magmas in the Baffin Bay lavas. Cont.
Min. Pet., 112,
230-241.
-
Sigurdsson, I.A., Steinthorson, S., and
Gronvold, K., 2000. Calcium-rich melt inclusions
in Cr-spinels from Borgarhraun, northern Iceland, Earth
Planet. Sci. Lett., 183,15-26,
doi:10.1016/S-0012-821X (00)00269-7.
-
Sobolev, ÅV.,
Hofmann, A.W., Sobolev, S.V., and Nikogosian, I.K.,
2005. An olivine-gree mantle source of Hawaiian
shield basalts, Nature, 434,
590597.
-
Yaxley, G.M., Kamenetsky, V.S.,
Kamenetsky, M., Norman, M.D., and Francis, D.,
2002. Origins of compositional heterogeneity in
olivine-hosted melt inclusions from the Baffin
Island picrites, Cont.
Min. Pet., 148,
426-442.
-
Wright, E., 1987. Mineralogical studies of
Samoan ultramafic xenoliths: implications for upper
mantle processes, In Fryer, P., Batiza, R., and
Boehlert, G.W. (eds.), Seamounts,
Islands, and Atolls, Geophysical Monograph,
43, Washington (American Geophysical Union), 221-234.
last updated 20th
August, 2008 |