James M.D. Day & David R. Hilton
Scripps Institution of Oceanography, University of California San Diego, La Jolla, CA 92093-0244, USA; jmdday@ucsd.edu ; drhilton@ucsd.edu
This webpage is a summary of: Day J.M.D., Barry P.H., Hilton D.R., Pearson D.G., Burgess R., Taylor L.A. (2015) The helium flux from the continents and ubiquity of low-3He/4He recycled crust and lithosphere. Geochimica et Cosmochimica Acta, 153, 116-133.
Helium isotope ratios (3He/4He) are sensitive tracers of mantle heterogeneity, indicating preservation of relatively undegassed reservoirs in Earth’s mantle since planetary accretion (e.g., Kurz et al., 1982). Two important but poorly characterized reservoirs for He are subducted oceanic crust and lithosphere and cratonic continental lithosphere. These reservoirs may play a direct role in the genesis of some intraplate lavas (e.g., Hofmann & White, 1982; McKenzie & O’Nions, 1983), and in He isotope mantle evolution (Gonnermann & Mukhopadhyay, 2009). It has been proposed that these reservoirs might also host high-3He/4He domains (e.g., Anderson, 2000; Albarède, 2008). If correct, they may have a significant impact on the noble gas compositions of volcanic rocks (Moreira & Kurz, 2001; Gautheron & Moreira, 2002; Day et al., 2005).
Helium isotope compositions for both cratonic and non-cratonic peridotite xenoliths can be explained through pervasive open-system behaviour of He in cratonic, mobile belt and tectonically-active regions (Gautheron & Moreira, 2002; Barry et al., 2015; Day et al., 2015). Using He isotope data for cratonic peridotites, and assuming that:
-
significant portions (>50 %) of the Archaean and Proterozoic continental lithospheric mantle (CLM) are stable and unaffected by melt or fluid infiltration on geological timescales (>0.1 Ga), and
-
U and Th contents vary between cratonic lithosphere and non-cratonic lithosphere, a 3He flux of 0.25-2.2 atoms/s/cm 2 is derived for the CLM ( Day et al., 2015).
These estimates differ by a factor of ten from non-cratonic lithospheric mantle (Gautheron & Moreira, 2002) and are closer to the observed 3He flux from the continents (<1 atoms/s/cm2) (Sano, 1986).
Pyroxenite and eclogite materials from the continental regions are widely interpreted to be the metamorphic/metasomatic equivalents of recycled oceanic crustal protoliths, and all are characterized by relatively low-3He/4He (0.03-5.6 RA) (Day et al., 2015). Together with oceanic peridotites (Moreira & Kurz, 2001), and the CLM, these materials represent mantle reservoirs with low time-integrated 3He/(U+Th). Pyroxenite and eclogite are also typically characterized by higher Fe/Mg, more radiogenic Os-Pb isotope compositions, and more variable δ18O values (~3 to 7‰), compared with peridotitic mantle. The low-3He/4He values of these reservoirs and their distinctive compositions make them probable end-members to explain the compositions of some low-3He/4He OIB, and provide an explanation for the low-3He/4He measured in most HIMU lavas. For example, it has been argued that He and O isotope compositions of Canary Island lavas as well as lavas from other HIMU islands are correlated, with this relationship representing mixing between at least four different mantle components (Day & Hilton, 2011):
- Recycled oceanic lithosphere that has suffered high-temperature hydrothermal alteration to generate low-δ18O and 3He/4He signatures with ~4 to 6 RA.
- Recycled oceanic crust, with extremely low-3He/4He (<0.1 RA), and a range of δ18O, broadly ranging from mantle-like to high-δ18O values.
- A high-3He/4He component with ≥11 RA and mantle-likeδ18O.
- A depleted mid-ocean-ridge-basalt mantle (DMM) component with mantle δ18Oolivine (5.2 ± 0.3 ‰) and 3He/4He of 8 ± 1 RA.
These end-member compositions also allow assessment of the approximate concentration of He in recycled oceanic crust, making the following assumptions: 3He/4He for DMM is 8 ± 1 RA with an He concentration of 1 × 10-5 cm3STP g-1 (Moreira & Kurz, 2001), and 3He/4He for ancient pyroxenites is equal to 0.05-1.2 with a ~10% contribution of 3He/4He from recycled oceanic crust. These calculations yield an estimated He concentration in recycled oceanic crust of ~2 to 10 × 10-7 cm3STP g-1. Helium isotope data for pyroxenites and eclogites, therefore, support models for the generation of HIMU 3He/4He from the partial melting of recycled oceanic crustal protoliths (e.g., Hanyu & Kaneoka, 1997; Hilton et al., 2000; Moreira & Kurz, 2001; Parai et al., 2009; Day & Hilton, 2011).
A basic tenet of partial melting models is that the concentrations of elements in different protoliths involved in melting are fundamental to generating the resulting composition of magmas. For example, a He-rich source (e.g., DMM, high-3He/4He mantle) can ‘overprint’ pre-existing mantle He-isotope heterogeneity, if He contents are sufficiently low in the host protoliths (e.g., recycled oceanic crust and lithosphere). Alternatively, at high temperatures, diffusion of He will lead to isotopic equilibration of He such that only completely isolated, or volumetrically large mantle reservoirs will preserve unique 3He/4He signatures. Transformation of recycled oceanic crustal protoliths to pyroxenite and eclogite at high temperatures will therefore inevitably result in them inheriting the 3He/4He signature of their ambient surrounding mantle. Thus, at locations where a strong recycled crust or lithospheric component is implicated in OIB mantle sources (HIMU; EM), the helium isotope ratio will be dominantly controlled by He derived from DMM, and possibly from a high-3He/4He component. Conversely, where OIB are associated with a high plume flux, or high degrees of partial melting and tholeiitic magmatism (e.g., Hawai’i, Iceland, Reunion, Galapagos, Siberian LIP), there may be greater interaction between DMM and a high-3He/4He mantle reservoir as enriched flavours (EM, HIMU) become more diluted as a consequence of higher degrees of partial melting.
CLM and recycled oceanic crust and lithosphere protoliths are not reservoirs for high-3He/4He and so alternative, volumetrically significant, He-rich reservoirs, such as less-degassed (lower?) mantle, are required to explain high-3He/4He signatures measured in some intraplate lavas.
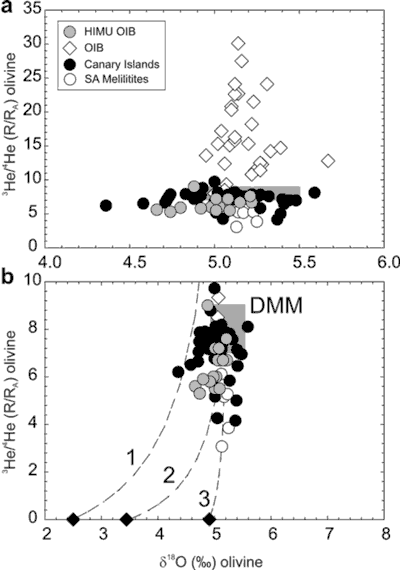
Figure 1: (a) 3He/4He versus 18O/16O variations in olivine phenocrysts from OIB and South African melilitites (SA melilitites) with HIMU-type Pb isotope compositions. Samples of OIB are divided into those with HIMU (here defined as 206Pb/204Pb >20), HIMU-type Canary Island, and OIB without HIMU (e.g., EM1, EM2, FOZO) affinity. Note the low-3He/4He of most HIMU and HIMU-type OIB and the larger range in δ18Oolivine for these samples compared with ‘OIB’. (b) Expanded view showing models of interaction between MORB (8 RA, δ18Oolivine = 5.3‰; models 2 and 3) or high-3He/4He peridotite (>15 RA, δ18Oolivine = 5.3‰; model 1) mantle melts mixing with pyroxenite and eclogite components with low-δ18O and low-3He/4He (<0.1 RA) and assuming 80% degassing of the peridotite mantle source. Uncertainties are generally smaller than symbols. See Day et al. (2015) for further details.
References
-
Anderson D.L. 2000. The statistics and distribution of helium in the mantle. International Geology Reviews, 42, 289-311.
-
Albarède F., 2008. Rogue mantle helium and neon. Science, 319, 943-945.
-
Barry P.H., Hilton D.R., Day J.M.D., Pernet-Fisher J.F., Howarth G.H., Agashev A.M., Pokhilenko N.P., Pokhilenko L.N., Taylor L.A. (2015) Volatile flux modification of cratonic lithosphere during the Permo-Triassic Siberian flood basalt event. Lithos, 216-217, 73-80.
-
-
Day J.M.D., Hilton D.R. (2011) Origin of 3He/4He ratios in HIMU-type basalts constrained from Canary Island lavas. Earth and Planetary Science Letters, 305, 226-234.
-
Day J.M.D., Hilton D.R., Pearson D.G., Macpherson C.G., Kjarsgaard B.A., Janney P.E. (2005) Absence of a high time-integrated 3He/(U+Th) source in the mantle beneath continents. Geology, 33, 733-736.
-
Gautheron C., Moreira M., 2002. Helium signature of the subcontinental lithospheric mantle. Earth and Planetary Science Letters, 199, 39-47.
-
Gonnermann H.M., Mukhopadhyay S., 2009. Preserving noble gases in a convecting mantle. Nature, 459, 560-563.
-
Hanyu T., Kaneoka I., 1997. The uniform and low 3He/4He ratios of HIMU basalts as evidence for their origin as recycled materials. Nature, 390, 273-276.
-
Hilton D.R., Macpherson C.G., Elliott T.R., 2000. Helium isotope ratios in mafic phenocrysts and geothermal fluids from La Palma, the Canary Islands (Spain): Implications for HIMU mantle sources. Geochimica et Cosmochimica Acta, 64, 2119-2132.
-
Hofmann A.W., White W.M., 1982. Mantle plumes from ancient oceanic crust. Earth and Planetary Science Letters, 57, 421-436.
-
Kurz M.D., Jenkins W.J., Hart S.R., 1982. Helium isotopic systematics of oceanic islands and mantle heterogeneity. Nature, 297, 43-47.
-
McKenzie D., O’Nions R.K., 1983. Mantle reservoirs and oceanic basalts. Nature, 301, 229-331.
-
Moreira M., Kurz M.D., 2001. Subducted oceanic lithosphere and the origin of the ‘high μ’ basalt helium isotopic signature. Earth and Planetary Science Letters, 189, 49-57.
-
Parai R., Mukhopadhyay S., Lassiter J.C., 2009. New constraints on the HIMU mantle from neon and helium isotopic compositions of basalts from the Cook-Austral Islands. Earth and Planetary Science Letters, 277, 253-261.
-
Sano Y., 1986. Helium flux from the solid Earth. Geochemical Journal, 20, 227–232.
last updated 28th
August, 2015 |