 |

|
The
Hoggar swell and volcanism, Tuareg shield,
Central Sahara: Intraplate reactivation of
Precambrian structures as a result of Alpine
convergence
|
|
|
Introduction
Hoggar (or Ahaggar) volcanism, in
the Central Sahara, is Eocene to Quaternary in age
(35 to nearly 0 Ma; Aït-Hamou et al.,
2000 and references therein). Hoggar is located within
the African plate far from the recent East African
rift system. Associated with a swell 1,000 km in diameter
(Figure 1), Hoggar Cainozoic volcanism is classically
considered to be a mantle plume product (Sleep,
1990) even though no thermal anomaly has been observed
(Lesquer et al., 1989). When considering
available geological, geophysical and petrological
data, such a model is hardly supported. When integrating
the data with the structure of the Precambrian basement
and the present geodynamic environment, an alternative
model comes to mind. This comprises a source at the
lithosphere/asthenosphere interface melted by adiabatic
pressure release in response to stress applied by
the Africa-Europe collision on pre-existing shear
zones and fractures, within a semi-rigid block (metacraton).
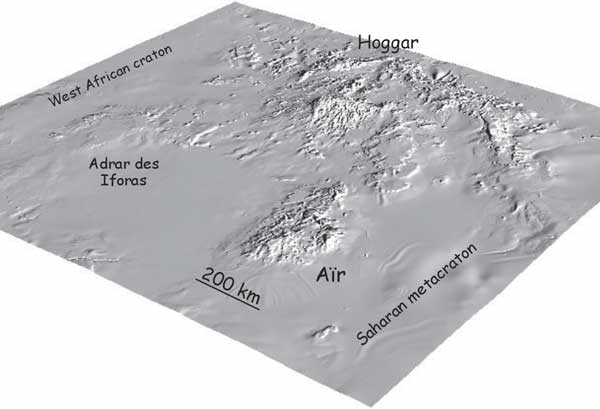
Figure 1: The Hoggar swell from
GLOBE data, NOAA; processing by Ph. Trefois, MRAC.
Click on image to enlarge.
|
The
Precambrian basement
Hoggar forms the main part of the Tuareg
shield, which is principally made
of Archaean/Palaeoproterozoic and Neoproterozoic terranes.
These amalgamated during the late Neoproterozoic Pan-African
orogeny (Black et al., 1994) as a result of
the convergence of the West African craton (WAC) and
the Saharan (meta)craton (Figure 2).
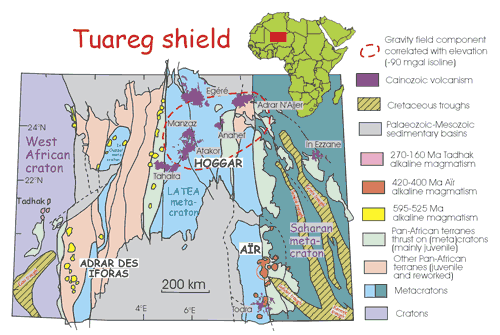
Figure 2: The Tuareg shield. Distinction
of major types of terranes (after Black et al., 1994;
Liégeois et al., 2003). Localities of alkaline
magmatism: post-collisional (595-525 Ma; Ba et al.,
1985; Liégeois & Black, 1987; Liégeois
et al., 1998); Devonian in Aïr (Moreau et al.,
1994); Permian-Jurassic in Tadhak (Liégeois et
al., 1991) and Cainozoic volcanism (drawn from a satellite
photograph – Orthorectified Landsat Thematic Mapper
Mosaics as compressed color imagery in MrSIDTM file
format from Lizardtech). Gravity anomaly from Lesquer
et al. (1988), Cretaceous troughs from Genik (1993).
Click on image to enlarge.
A first stage comprised the accretion
of oceanic island arcs on these cratons and on microcratons
during the period 900-680 Ma. Relics of these terranes,
including ophiolites and eclogites, are preserved as
thrust sheets on more rigid bodies (Liégeois
et al., 2003 and references therein).
The second stage was the regional northerly
tectonic escape of the Tuareg terranes due to oblique
collision with the WAC. It is characterised by spectacular
N-S shear zones. During that stage, the metacratonization
of the LATEA (Central Hoggar) microcontinent occurred,
i.e. the squeezing of this rigid body, which was torn
into several moving blocks. For a description of the
metacraton concept, see Abdelsalam et al.,
2002. This induced sliding along N-S mega-shear zones
and the intrusion of granitoid batholiths (615-580 Ma)
with geochemical signatures indicative of the lower
crust (Sri= 0.710; epsilonNd=
-20). Linear lithospheric delamination beneath these
mega-shear zones may occur under such circumstances,
allowing a drastic increase in heat flow and melting
of the crust. Post-collisional and anorogenic high-level
alkaline plutons are aligned on the same mega-shear
zones, particularly along (meta)craton margins (Figure
2; Liégeois et al., 1998; Azzouni-Sekkal
et al., 2003). |
Hoggar during the
Palaeozoic and Mesozoic
At the beginning of the Phanerozoic, the Tuareg shield
was entirely eroded and covered by Ordovician sandstones
whose source region was to the south. The shield did
not constitute an obstacle for the sedimentation flux
(Beuf et al., 1969).
The presence of rare Cretaceous continental remnants
lying directly on top of Hoggar Precambrian indicates
that the shield was already uplifted and slightly
re-eroded before the Cretaceous. The palaeo-currents
in Cretaceous sediments around the shield indicate
that Hoggar was bypassed by the sediment flux from
the north (Faure, 1985). Hoggar, as well
as the Eglab and Tibesti massifs, was an island during
the Cretaceous, being only covered by continental
lakes (Fabre, 1976). This suggests that the
swell already existed during the Cretaceous. The current
presence of Cretaceous deposits at an altitude of
2,000-3,000 meters (Rognon; Fabre, 1976)
indicate the importance of uplift during the Cainozoic,
however. This Cretaceous uplift could be linked to
the development of a series of Cretaceous elongate
basins in West Africa (Figure 2; Genik, 1993;
Dautria & Lesquer, 1989).
Cainozoic volcanism
& plutonism
Recent volcanic activity began during the late Eocene
(35-30 Ma; Aït-Hamou et al., 2000) and
lasted probably till the upper Palaeolithic or even
Neolithic (Lelubre, 1952). It occurred in
several districts (Figure 2): Tahalra (1,800 km2),
Atakor (2,150 km2), Manzaz (1,500 km2),
Egéré (2,800 km2), Anahef
(400 km2) and Adrar N'Ajjer (2,500 km2),
all in Central Hoggar, and also in Teria (100 km2)
to the NE, In Ezzane (800 km2) to the east
and Todra (1,050 km2) to the south of Aïr.
This volcanism forms high relief, often over 2,000
m in altitude, culminating in the Atakor (Mt. Tahat,
which is 2,918 m high). The Hoggar swell reaches an
altitude of 350-400 m on its margin and 1,000-1,500
in its centre. (The town of Tamanrasset is at an altitude
of 1420 m). Some Precambrian basement inliers have
been observed in Atakor at an altitude of up to 2,600
m.
|
Three
main stages can be identified for Hoggar volcanism:
-
The oldest stage is Upper Eocene
to Oligocene and is present only in the Anahef district.
It corresponds to tholeiitic basalts of fissural
origin (thickness of 600 m; 35-30 Ma; Aït-Hamou
et al., 2000), intruded by a dozen subvolcanic
ring complexes (c. 29 Ma for the Achkal complex;
Maza et al., 1995) made of gabbros, diorites,
monzonite, alkali-feldspar syenites and nepheline
syenites. The largest is the Tellerteba ring complex,
which is 8 x 5 km in size. These complexes are covered
by alkaline rhyolites whose age (c. 24 Ma) suggests
uplift of 0.4 mm/year (Maza et al., 1995).
The deeper levels currently exposed in the Anahef
district are then linked to contemporaneous uplift
of Hoggar.
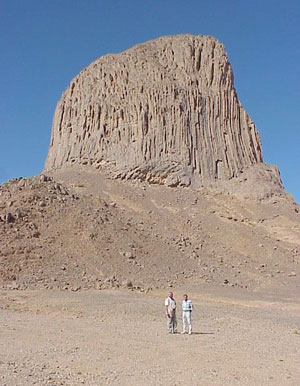 |
-
The second stage is the
most voluminous and is well represented in
the Atakor district (Girod, 1971).
It occurs between 20 and 12 Ma and between
7 and 4 Ma (Rognon et al., 1983)
and comprises 80% basalts and 18% trachytes
and phonolites (Figure 3).
-
The latest stage is late
Pliocene to late Quaternary (3 Ma to upper
Palaeolithic). In Atakor, this volcanism only
comprises basanites and nephelinites, which
follow valleys and can cover upper Palaeolithic
terraces. In the Tahalra district, and also
probably in the lesser-known Manzaz, Egéré
and Adrar N'Ajjer districts, most of the volcanic
activity occurred between 3.5 and 2.5 Ma (Aït-Hamou,
2000). It comprises 95% alkali basalts (basanitic
and hawaiitic flows). Some volcanoes are more
recent, and some cover Neolithic terraces.
Figure 3. The Iharen phonolitic plug, near
Tamanrasset.
|
The In Ezzane district is almost unknown,
due to its remote location (Figure 4). The In Teria
district comprises melilitic rocks rich in xenoliths
(Bossière & Megartsi, 1982; Dautria
et al., 1992). In Aïr (Niger), the Todra district
(Black et al., 1967) began with about 30 trachytic
and phonolitic volcanoes, many erupting very viscous
lavas and trachytic tuffs. The Todra volcano itself
lies at an altitude of 1,780 m. This stage was followed
by the formation of about 130 basaltic volcanoes (Figure
5). They are generally regular cones and only one flow
following valleys was generated. Black et al.
(1967) estimated that some volcanoes cannot be more
than a few centuries old. They remarked also that these
volcanoes are located either on NW-SE oriented faults
or on annular faults linked to Devonian ring complexes
(Figure 6). The NW-SE faults belong to conjugate brittle
faults that developed at the end of the Pan-African
orogeny, due to the WAC indentation (Ball,
1980).
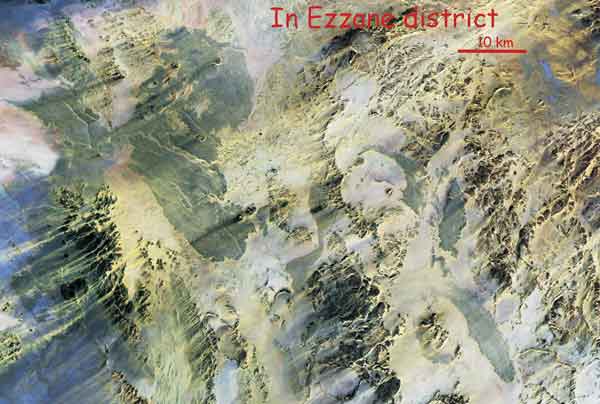
Figure 4. Satellite
photograph of the In Ezzane district (Orthorectified
Landsat Thematic Mapper Mosaics as compressed color
imagery in MrSIDTM file format from Lizardtech). The
lavas appear blue. The circular structures have not
yet been studied. Click on image to enlarge.
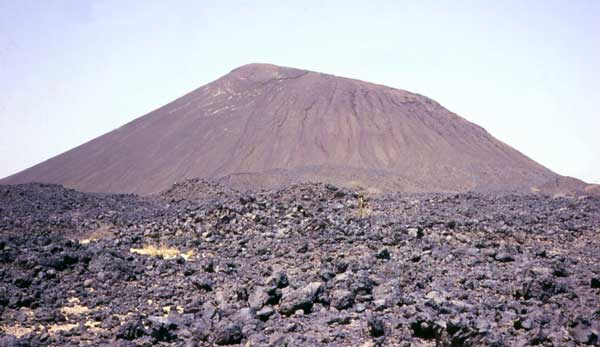
Figure 5. Example
of a small Aïr basaltic volcano, which generated
one flow. |
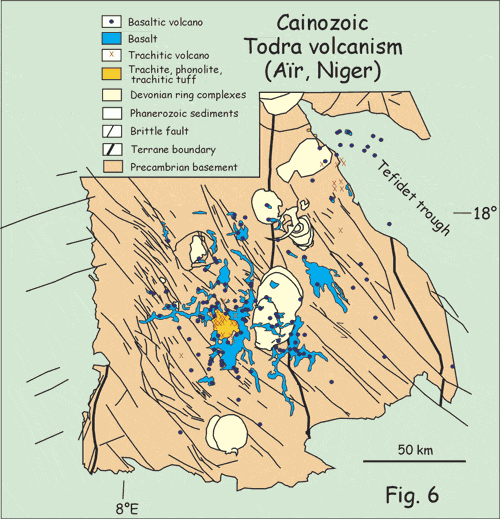
Figure 6. Map of southern Aïr showing volcanoes
and lava flows and their link with the terrane boundaries,
the late Pan-African brittle faults and the Devonian
ring faults (from Black et al., 1967; 1985; Liégeois
et al., 1994).
|
Geophysical
data
Crough (1981) proposed that
Central Hoggar is underlain by anomalously light mantle,
on the basis of free air gravity data. He interpreted
this as indicating a magmatic body at a depth of <
60 km below the surface. Lesquer et al. (1988)
analysed the shape of the anomaly (Figure 2), which
appears to be correlated with the basement elevation,
and suggested that the body lies between 20 and 70 km
depth and has a maximum thickness of 30 km. This association
between the gravity anomaly and the Cainozoic volcanism
led to the suggestion that a mantle plume underlies
Hoggar (Aït-Hamou & Dautria, 1994;
Aït Hamou et al., 2000). However, heat
flow measured inside the Hoggar swell is normal (50
mW/m2) and there is no evidence for a thermally
perturbed lithosphere (Lesquer et al., 1989).
On the contrary, a decrease in heat flow is observed
with basement elevation, which is inconsistent with
a thermal uplift. Only a minor thermal structure is
possible below the centre of Atakor, where heat flow
of 63 mW/m2 has been measured (Lesquer
et al., 1989). The latter suggests that small high-temperature
mantle bodies intrude the crust beneath the recent volcanic
areas (Ayadi et al., 2000).
The low-density zone proposed on the
basis of gravity data thus cannot be interpreted as
an upwelling of the lithosphere/asthenosphere boundary.
In contrast, a large thermal anomaly occurs to the north,
below the Saharan basins (Lesquer et al., 1990;
Lesquer & Vasseur, 1992). A low P-wave
velocity structure has been detected beneath Central
Hoggar, extending from the surface down to 300 km (Ayadi
et al., 2000). The velocity contrast with adjacent
areas is modest, however, and the anomaly is strongest
beneath Atakor and Tahalra where it reaches -5% between
74 and 114 km depth. Considering both the seismic and
heat flow data, Lesquer & Vasseur (1992)
proposed that the mantle beneath Central Hoggar is intermediate
between cratonic mantle and activated mantle. If this
anomaly is related to a hot body, the latter must have
been emplaced before 60 Ma in order to have cooled off
(Lesquer et al., 1988). |
The
data from the xenolith included within the alkali basalts
Following the work of Girod et
al. (1981) and Dautria et al. (1987),
it may be concluded that amphibole-rich xenoliths from
Hoggar alkali basalts indicate a mantle metasomatism
event during or just before (max. 40 Ma) the generation
of Hoggar magma between 1,000°C and 1,100°C
and 15 to 18 kbar. Xenoliths and lavas have low 87Sr/86Sr
isotopic ratios – between 0.70306 and 0.70344.
Based on the xenoliths, the source is enriched by a
factor of 7–9 chondritic in LREE and 2 in HREE.
The degrees of partial melting vary from 1.2 to 2.1%
for nephelinitic melts and 3.8% to 4.4% for basanitic
melts, both leaving a garnetiferous residuum. There
is a positive correlation between the degree of deformation
of the xenoliths and the abundance of "metasomatic"
minerals (principally amphibole), which indicates that
the fluids were preferentially injected along strained
zones. The wide regional distribution and abundance
of such amphibole-rich rocks in the Hoggar upper mantle
indicate that it is highly veined and hydrous along
the shear zones. Such mantle metasomatization could
be the cause of the present uplift of the Hoggar. In
addition, the Hoggar basement uplift could be seen as
an isostatic response to upper mantle density reduction
controlled by magmatic events and associated metasomatism.
The
isotopic data
Pb, Sr and Nd isotopic compositions
of Hoggar volcanic rocks lie within the EM1 and HIMU
components (Allègre et al., 1981; Dupuy
et al., 1993; Aït-Hamou et al., 2000),
the influence of the latter seeming to increase with
time. The main alkaline basalts have 87Sr/86Sr
varying from 0.7030 to 0.7037, epsilonNd
from +3 to +7, 206Pb/204Pb from
19.3 to 20.5 and 207Pb/204Pb from
15.59 to 15.70, while the older (c.35 Ma) Taharaq tholeiitic
basalts have 87Sr/86Sr varying
from 0.7035 to 0.7045, epsilonNd from -2.5
to +3, 206Pb/204Pb from 18.3 to
19.5 and 207Pb/204Pb from 15.54
to 15.60. The EM1 pole has been attributed to the Pan-African
lithosphere and the HIMU component (with some DM) to
a plume, itself recycling subducted Proterozoic oceanic
lithosphere (Aït-Hamou & Dautria,
2000). |
The
geodynamics of the African plate during late Mesozoic
and Cainozoic
During the Cretaceous, the African
plate was subjected to an important rifting event. This
led to the equatorial Atlantic opening and to the generation
of intraplate rifts, including the Bénué
rift (Guiraud & Bosworth, 1997, and references
therein). In the Hoggar area, Cretaceous rifts developed
to the SE, within the Saharan metacraton (Figure 2).
They show sediment thicknesses of (Genik, 1993):
- Kermit trough – 10 km of Cretaceous (K) and
5 km of Cainozoic (C)
- Ténéré trough – 5
km of K and 2 km of C
- Tefidet trough – 3 km of K and 1 km of
C
- Grein trough – 1.5 km of K, 1.5 km of
Palaeogene and 2 km of Miocene/Holocene.
This indicates contemporaneity of the
Hoggar swell and the development of nearby troughs.
During the Cretaceous and the Cainozoic, the African
plate moved towards Europe, changing direction from
NE to NW. The main tectonic events occurred at 112,
85, 65, 37, 20, 8 and 1 Ma at the plate margin, with
effects within the African plate (Guiraud &
Bosworth, 1997 and references therein). At 35 Ma
Hoggar was located at 3°W and 18°N (using theGeomar-ODSN
reconstruction facility - http://www.odsn.de/),
i.e. 1,000 km to the SW, near the current position of
Timbuktu. |
An
alternative to the plume hypothesis
The constraints on Hoggar volcanism are:
-
If the negative gravity anomaly
associated with the Hoggar swell is linked to a
formerly hot body, the latter must be older than
60 Ma, to have had enough time to cool. It could
be much older, however.
-
Recent Hoggar volcanism is associated
with a swell but not a thermal anomaly. A current
large thermal anomaly is located to the north, below
the Saharan basins, while the African plate has
moved to the north-east. This thermal anomaly thus
cannot be the present signature of a former Hoggar
plume.
-
Slightly higher heat flow has
been measured very locally in the Atakor, suggestive
of local intrusion of asthenospheric mantle into
the Hoggar crust.
-
As a whole, Central Hoggar has
a structure intermediate between a craton and a
mobile belt, which corresponds to the concept of
a metacraton (a partially destabilized craton).
Coupled seismic and heat flow data indicate also
that the mantle beneath Central Hoggar is intermediate
between cratonic mantle and active mantle.
-
In Hoggar, strong xenolith deformation
is observed, indicating the importance of modal
mantle metasomatism. Fluids are preferentially injected
along strained zones.
-
The source of the Hoggar basalts
is REE enriched. Their Sr-Nd-Pb isotopic ratios
indicate an enriched mantle source, particularly
at the beginning of the event. The involvment of
old radiogenic lithosphere is very weak.
-
The movement of Africa towards
the north induced several tectonic phases (T) the
latest of which appears to have been followed by
volcanism (V) in Hoggar at T= 37 Ma, V= 35-30 Ma;
T= 20 Ma, V= 20-12 Ma; T=8 Ma, V= 7 – 2 Ma;
and T= 1 Ma, V= most recent.
It may be added that the location of
Hoggar, and more widely, Tuareg volcanism is linked
to the rheological characteristics of the Pan-African
terranes. It is located along shear zones within or
at the boundaries of metacratons (i.e., semi-rigid blocks;
Figure 2). It is also influenced by the late Pan-African
brittle conjugate fault system (Figure 6). We could
suggest the application of what has been demonstrated
recently for the Bicol volcanic arc (Pasquarè
& Tibaldi, 2003) where magma rose at depth
along NW-striking transcurrent faults, but where in
the uppermost crust magma rose along NE-striking fractures,
parallel to the direction of greatest principal stress.
Indeed, in the Hoggar-Tuareg shield, the general locations
of the volcanic districts are related to the main shear
zones while the precise locations of individual volcanoes
are determined either by NE-SW or the NW-SE fractures.
We know that the African plate moved, following the
period considered, either to the NE (84-37 Ma; 21-8
Ma) or the NW (37-21 Ma; 8-0 Ma). More detailed study
is needed to determine the details of this correlation.
On the other hand, no trace of a plume is observed between
the present location of Central Hoggar and its former
position 35 Ma ago, c. 1,000 km to the WSW, near the
current position of Timbuktu.
Hoggar volcanism may thus be the consequence
of intraplate rejuvenation of Pan-African structures
linked to the Africa-Europe collision. Based on the
model proposed for the Pan-African (late Neoproterozoic)
granitoids that intruded in same areas, I suggest that
linear lithospheric delamination occurred along the
Pan-African mega-shear zones located inside metacratons
or at their margins (Figure 7). This event was more
limited in intensity than at the end of the Pan-African
orogeny. It allowed the rise of enriched material from
the lithosphere/asthenosphere boundary (Black &
Liégeois, 1993; Anderson,
1995) that can melt by adiabatic pressure release
but this hot material was not abundant enough to melt
the old lithosphere nor to generate a thermal anomaly,
except very locally. (Minor involvement could be envisaged
for the early tholeiitic basalts, generated by a larger
degree of partial melting and characterized by a more
enriched isotopic signature.) The role of the relatively
shallow light body (20-70 km) is not yet clear as its
age is not known. It is older than 60 Ma, and could
be much older. Together with the mantle metasomatism
linked to Hoggar volcanism and with the stress transmitted
from plate boundaries, it could have contributed to
the uplift and generation of the Hoggar swell. We must
not forget, however, that the uplift observed in Aïr
(Figure 1) is also important (the basement is elevated
to up to 1,900 m) and is located far from this anomaly.
It is, in contrast, located on the western margin of
the Saharan metacraton, which is marked by one of the
most important Pan-African shear zones in the Tuareg
shield.
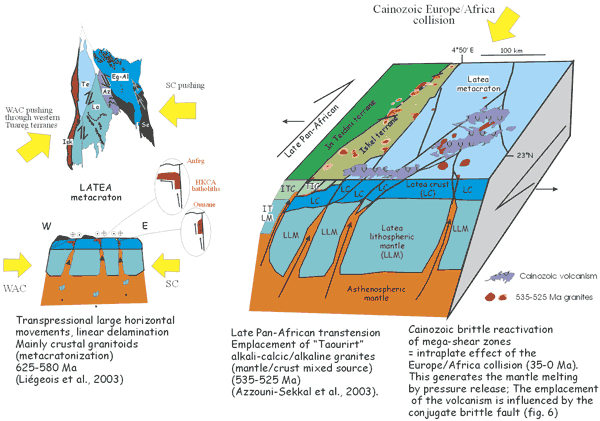
Figure 7. Proposed alternative model
for Hoggar. During the post-collisional Pan-African
period, the LATEA craton was dissected by mega-shear
zones (metacratonization). Major linear delamination
induced asthenospheric upwelling able to melt the lower
crust. this gave rise to mainly crustal granitoids (620-580
Ma; Liégeois et al., 2003 and references therein).
At the end of the convergence, a similar event occurred
but with less intensity, and gave rise to high-level
alkali-calcic plutons of mixed mantle/crust origin (535-525
Ma; Azzouni-Sekkal et al., 2003 and references therein).
This model proposed a similar scenario for the recent
volcanism, that it is the intraplate consequence of
Africa-Europe convergence. An even lower intensity event
could cause the mainly mantle origin of Hoggar volcanism.
See text for more details. Click on image to enlarge.
|
-
Abdelsalam, M., Liégeois,
J.P. and Stern, R.J., 2001. The Saharan metacraton.
Journal African Earth Sciences, 34,
119-136.
-
Aït-Hamou, F. and Dautria,
J.M., 1994. Le magmatisme cénozoïque
du Hoggar: une synthèse des données
disponibles. Mise au point sur l'hypothèse
d'un point chaud. Bulletin Service Géologique
Algérie, 5, 49-68.
-
Aït-Hamou, F., Dautria,
J.M., Cantagrel, J.M., Dostal, J. and Briqueu, L.,
2000. Nouvelles données géochronologiques
et isotopiques sur le volcanisme cénozoïque
de l'Ahaggar (Sahara algérien): des arguments
en faveur d'un panache. Comptes Rendus Académie
Sciences Paris, 330, 829-836.
-
Allègre, C.J., Dupré,
B., Lambert, B. and Richard, P., 1981. The subcontinental
versus the suboceanic debate. Lead-neodymium-strontium
isotopes in primary basalts in a shield area: the
Ahaggar volcanic suite. Earth Planetary Science
Letters, 52, 85-92.
-
-
Ayadi, A., Dorbath, C., Lesquer,
A. and Bezzeghoud, M., 2000. Crustal and upper mantle
velocity structure of the Hoggar swell (Central
Sahara, Algeria). Physics Earth Planetary Interiors,
118, 111-123.
-
Azzouni-Sekkal, A., Liégeois,
J.P., Bechiri-Benmerzoug, F., Belaidi-Zinet, S.
and Bonin, B., 2003. The "Taourirt" magmatic
province, a marker of the closing stages of the
Pan-African orogeny in the Tuareg Shield: review
of the available data and Sr-Nd isotope evidence.
Journal African Earth Sciences, in press.
-
Ba, H., Black, R., Benziane,
B., Diombana, D., Hascoet-Fender, J., Bonin, B.,
Fabre, J. and Liégeois, J.P., 1985. La province
des complexes annulaires alcalins sursaturés
de l'Adrar des Iforas, Mali. Journal African
Earth Sciences, 3, 123-142.
-
Ball, E., 1980. An example of
very consistent brittle deformation over a wide
intracontinental area: the late Pan-African fracture
system of the Tuareg and Nigerian shield. Tectonophysics,
61, 363-379.
-
Beuf, S., Biju-Duval, B., de
Charpal, O., Rognon, P., Gariel, O., Bennacef, A.,
1971. Les grès du Paléozoïque
inférieur au Sahara. Publication IFP, Collection
"Science et techniques du pétrole",
Paris, 464 pp.
-
Black, R., Jaujou, M. and Pellaton,
C., 1967. Notice explicative sur la carte géologique
de l'Aïr, à l'échelle du 1/500000ième.
Direction des Mines et de la Géologie, République
du Niger, Editions du BRGM, 57 pp.
-
Black, R., Latouche, L., Liégeois,
J.P., Caby, R. and Bertrand, J.M., 1994. Pan-African
displaced terranes in the Tuareg shield (central
Sahara). Geology, 22,
641-644.
-
Black, R., Lameyre, J. and Bonin,
B., 1985. The structural setting of alkaline complexes.
Journal African Earth Sciences, 3,
5-16.
-
Black, R. and Liégeois,
J.P., 1993. Cratons, mobile belts, alkaline rocks
and continental lithospheric mantle: the Pan-African
testimony. Journal Geological Society of London,
150, 89-98.
-
Bossière, G. and Megartsi,
M., 1982. Pétrologie des nodules de pyroxénites
associés à la rushayite d'In Téria
(NE d'Illizi, ex Fort Polignac), Algérie.
Bulletin Minéralogie, 105,
89-98.
-
Crough, S.T., 1981. Free-air
gravity over the Hoggar massif, northwest Africa:
evidence for the alteration of the lithosphere.
Tectonophysics, 77, 189-202.
-
Dautria, J.M., Dupuy, C., Takherist,
D. and Dostal, J., 1992. Carbonate metasomatism
in the lithospheric mantle: peridotitic xenoliths
from a melilitic district in the Sahara basin. Contributions
Mineralogy Petrology, 111,
37-52.
-
Dautria, J.M. and Lesquer, A.,
1989. An example of the relationship between rift
and dome: recent geodynamic evolution of the Hoggar
swell and of its nearby regions (Central Sahara,
Southern Algeria and Eastern Niger). Tectonophysics,
163, 45-61.
-
Dautria, J.M., Liotard, J.M.,
Cabanes, N., Girod, M. and Briqueu, L., 1987. Amphibole-rich
xenoliths and host alkali basalts: petrogenetic
constraints ans implications on the recent evolution
of the upper mantle beneath Ahaggar (Central Sahara,
southern Algeria). Contributions Mineralogy
Petrology, 95, 133-144.
-
Dupuy, C., Chikhaoui, M. and
Dostal, J., 1993. Trace element and isotopic geochemistry
of Cenozoic alkali basaltic lavas from the Atakor
(Central Sahara). Geochemical Journal,
27, 131-145.
-
Fabre, J., 1976. Introduction
à la géologie du Sahara algérien.
I. La couverture phanérozoïque.
S.N.E.D., Alger, 422 pp.
-
Faure, H., 1985. Aspects
généraux du Tertiaire et du Quaternaire
de l'Afrique. CIFEG, Paris.
-
Genik, G.J., 1993. Petroleum
geology of Cretaceous-Tertiary rift basins in Niger,
Chad and Central African Republic. American
Association Petroleum Geologists Bulletin,
77, 1405-1434.
-
Girod, M., 1971. Le massif
volcanique de l'Atakor (Ahaggar, Sahara algérien).
Mémoire CRZA, série 12, Editions CNRS,
Paris.
-
Girod, M., Dautria, J.M. and
de Giovanni, R., 1981. A first insight into the
constitution of the upper mantle under the Hoggar
area (southern Algeria): the lherzolite xenoliths
in the alkali basalts. Contributions Mineralogy
Petrology, 77, 66-73.
-
Guiraud, R. and Bosworth, W.,
1997. Senonian basin inversion and rejuvenation
of rifting in Africa and Arabia: synthesis and implications
to plate-scale tectonics. Tectonophysics,
282, 39-82.
-
Lesquer, A., Bourmatte, A., and
Dautria, J.M., 1988. Deep structure of the Hoggar
domal uplift (Central Sahara, south Algeria) from
gravity, thermal and petrological data. Tectonophysics,
152, 71-87.
-
Lesquer, A., Bourmatte, A., Ly,
S. and Dautria, J.M., 1989. First heat flow determination
from the Central Sahara: relationship with the Pan-African
belt and Hoggar domal uplift. Journal African
Earth Sciences, 9, 41-48.
-
Lesquer, A., Takherist, D., Dautria,
J.M. and Hadiouche, O., 1990. Geophysical and petrological
evidence for the presence of an anomalous upper
mantle beneath the Sahara basins (Algeria). Earth
Planetary Science Letters, 96,
407-418.
-
Lesquer, A. and Vasseur, G.,
1992. Heat-flow constraints on the West African
lithosphere structure. Geophysical Research
Letters, 19, 561-564.
-
Liégeois, J.P. and Black,
R., 1987. Alkaline magmatism subsequent to collision
in the Pan-African belt of the Adrar des Iforas
(Mali). In: Alkaline Igneous Rocks (J.G.
Fitton and B.J.G Upton, eds), Geological Society
Special Publication, London, 30,
381-401.
-
Liégeois, J.P., Black,
R., Navez, J. and Latouche, L., 1994. Early and
late Pan-African orogenies in the Aïr assembly
of terranes (Tuareg shield, Niger). Precambrian
Research, 67, 59-88.
-
Liégeois, J.P., Latouche,
L., Boughrara, M., Navez, J. and Guiraud, M., 2003.
The LATEA metacraton (Central Hoggar, Tuareg shield,
Algeria): behaviour of an old passive margin during
the Pan-African orogeny. Journal African Earth
Sciences, in press.
-
Liégeois, J.P., Navez,
J., Hertogen, J. and Black, R., 1998. Contrasting
origin of post-collisional high-K calc-alkaline
and shoshonitic versus alkaline and peralkaline
granitoids. Lithos, 45,
1-28.
-
Liégeois, J.P., Sauvage,
J.F. and Black, R., 1991. The Permo-Jurassic alkaline
Province of Tadhak, Mali: geology, geochronology
and tectonic significance. Lithos, 27,
95-105.
-
Maza, M., Dautria, J.M., Briqueu,
L. and Cantagrel, J.M., 1995. Massif annulaire de
l'Achkal: un témoin d'un magmatisme alcalin
d'âge oligocène supérieur au
Hoggar centro-oriental. Bulletin Service Géologique
Algérie, 6, 61-77.
-
Moreau, C., Demaiffe, D., Bellion,
Y. and Boullier, A.M., 1994. A tectonic model for
the location of Paleozoic ring-complexes in Aïr
(Niger, West Africa). Tectonophysics, 234:
129-146.
-
Pasquaré, F.A. and Tibaldi,
A., 2003. Do transcurrent faults guide volcanic
growth? The case of NW Bicol volcanic arc, Luzon,
Philippines. Terra Nova, 15,
204-212.
-
Rognon, P., Gourinard, Y., Bandet,
Y., Koeniguer, J.C. and Delteil-Desneux F., 1983.
Précisions chronologiques sur l'évolution
volcano-tectonique et géomorphologiques de
l'Atakor (Hoggar): apports des données radiométriques
(K/Ar) et paléobotaniques (bois fossiles).
Bulletin Société Géologique
France, XXV, 973-980.
-
Sleep, N.H., 1990. Hot spots
and mantle plumes: Some phenomenology. Journal
Geophysical Research, 95,
6715–6736.
last updated 28th May,
2006 |
|
|