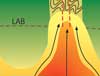 |
Ancient
lithosphere blobs beneath the oceans:
spelling out the geochemists’ alphabet
and understanding ocean basin lithosphere |
|
Suzanne
O’Reillya, Ming
Zhanga, William
Griffina & Graham Begga,b
aGEMOC
National Key Centre, Department of Earth and Planetary
Sciences, Macquare University, NSW 2109, Australia
sue.oreilly@mq.edu.au, mzhang1951@live.com.au, bill.griffin@mq.edu.au
bMinerals
Targeting International PL, 17 Prowse Street, West
Perth, WA 6005, Australia
graham@mineralstargeting.com
Also see: www.gemoc.mq.edu.au/Annualreport/annrep2008/Reshigh08.html
This webpage is a summary of: O'Reilly,
S.Y., Zhang, M., Griffin, W.L., Begg, G. and Hronsky,
J. 2009. Ultradeep continental roots and their
oceanic remnants: a solution to the geochemical "mantle
reservoir" problem? Lithos, 112,
S2, 1043-1054. 
The nature of continental Archean lithospheric
mantle
Archean subcontinental lithospheric
mantle (SCLM) is distinctive in its highly depleted
composition, commonly strong stratification,
and the presence of rock types (e.g.,
depleted, low-Fe harzburgite) absent in younger
SCLM (Griffin & O’Reilly,
2007; Griffin et al., 2009).
Primary (unmetasomatised) Archean lithosphere is
very low in basaltic components such as Al, Ca,
Fe, consists dominantly of dunite and Ca-poor harzburgite
and has high seismic wave velocities, mainly due
to the high proportion of Mg-rich olivine (Fo92-94).
Archean lithosphere is significantly less dense
than asthenosphere at any depth, and this buoyancy
means that it cannot be gravitationally delaminated;
it needs mechanical disaggregation (e.g.,
rifting) and/or metasomatic reworking to be disrupted.
Oceanic Archean mantle revealed in tomographic
models
Figure 1 shows tomographic slices
through the oceanic lithosphere and upper mantle
of the Atlantic Ocean Basin at 0-100 km, 100-175
km and 175-250 km. We use a high-resolution global
tomography model derived from SH body-wave travel
times based on the approach of Grand (2002)
and fully described by Begg
et al. (2009).
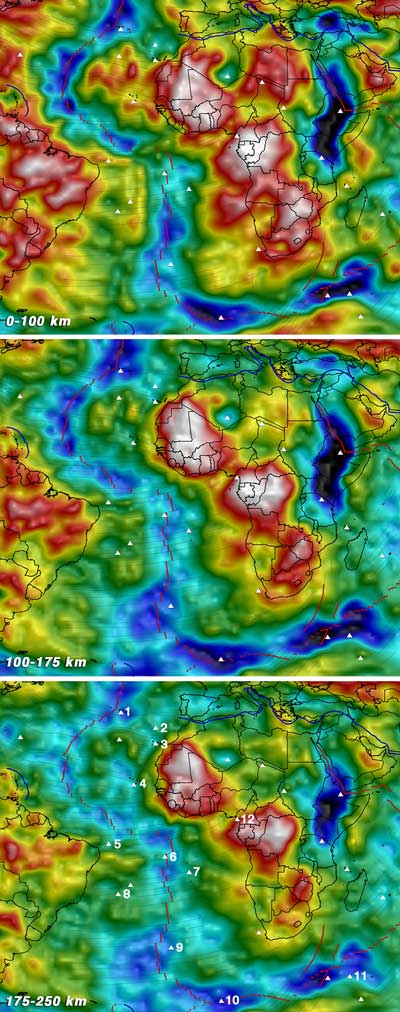
Figure 1. Tomographic model
images at three depth slices for the Atlantic
Ocean Basin (see text, Begg
et al., 2009 and
O’Reilly
et al, 2009 for details of
the model and colour scales). Note that “hot” (red-white)
colours indicate higher velocities and cool colours
lower velocities. Relevant OIB Provinces are:
1, Azores; 2, Madeira; 3, Canary Islands; 4,
Cape Verde; 5, Fernando de Noronha; 6, Ascension
Islands; 7, St. Helena; 8, Trindade; 9, Tristan
da Cunha (Walvis Ridge at ~130 Ma; Richardson
et al., 1984); 10, Bouvet; 11, Crozet Archipelago
(Afanasy – Nikitin Rise in the Indian Ocean
at Late Cretaceous, ~115-80 Ma; Mahoney et al.,
1996); 12, Cameroon Line.
In the 0-100 km section, high-velocity regions
are obvious. Some are apparently continuous with
continental regions (especially off southwestern
Africa and southeastern South America) and some
occur as discrete “blobs” within the
ocean basin, from the continental margins to the
mid-ocean ridge. In the layer from 100-175 km,
these fast domains persist, and some also show
velocity contrasts in the 175-250 km layer.
A traditional interpretation for high-velocity
regions at the margins of ocean basins is the effect
of cooling of the oceanic lithosphere with time
and distance from the ridge. However, this cannot
be the explanation for the discrete blobs that
lie within the ocean basin, both away from the
original rift margins and near the present-day
ridge, with some extending to depths of 250 km.
We suggest that these high-velocity volumes represent
remnants of depleted (buoyant), ancient continental
lithosphere, fragmented and stranded during the
rifting process at the opening of the ocean basin.
The high-velocity domains extending out from the
coastlines are not uniformly distributed along
the basin edge. The most marked high-velocity regions,
off SE South America and northwest and southwest
Africa, appear to be continuous with their respective
continental deep structure as seen in the tomographic
models. The global magnetic-anomaly map (Korhonen
et al., 2007; Figure 2) shows that these
regions have a complex magnetic signature that
is consistent with extended continental crust,
and distinct from that of oceanic lithosphere,
which is characterised by the regular magnetic
striping produced at spreading centres.
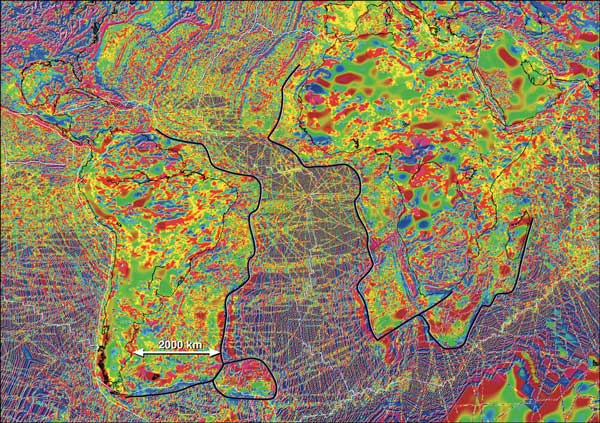
Figure 2. Modified extract
from the global magnetic anomaly map (Korhonen
et al., 2007, Magnetic Anomaly Map of the World
Scale: 1:50,000,000, 1st edition, Commission
for the Geological Map of the World, Paris, France)
showing the Atlantic Ocean Basin and Atlantic
coasts of South Africa and South America. Black
lines outline the regions with crustal rather
than oceanic magnetic characteristics. Click
here or on Figure for enlargement.
Old Re-Os ages for mantle sulfides
in some depleted mantle rock types beneath rift
zones and oceanic areas (see references in O’Reilly
et al.,
2009 and Coltorti
et al.,
2010)
suggest that these high-velocity blobs (inferred
to have high Mg# and low density) represent relict
Archean to Proterozoic SCLM (now refertilised to
varying degrees, during episodes of mantle fluid
inflitration reflecting larger-scale tectonic events)
that was mechanically disrupted and thinned during
the formation of the oceanic lithosphere. This
interpretation implies that ocean basins do not
form by clean breaks at now-observed continental
boundaries, but that significant volumes of buoyant
old mantle are embedded within the newly generated
oceanic lithosphere. The opening of ocean basins
may be largely by listric faulting mechanisms,
leaving significant wedges of continental lithosphere
at rifted margins, and stranding domains of ancient
lithosphere in the upper part of the new oceanic
crust-mantle system, where they would remain as
buoyant blobs.
If the higher-velocity coherent blobs observed
at depths up to >150 km in the upper mantle
of the Atlantic Ocean do represent remnant Archean
mantle roots, this has important implications for
the nature of global convection. Models involving
large-scale horizontal movement would be difficult
to reconcile with these observations. Instead,
convection may be dominantly in the form of upwelling
vertical conduits with shallow horizontal flow
(Figure 3). The locus of these conduits may be
controlled by the geometry of the margins and the
coherence of the buoyant lithospheric blobs.
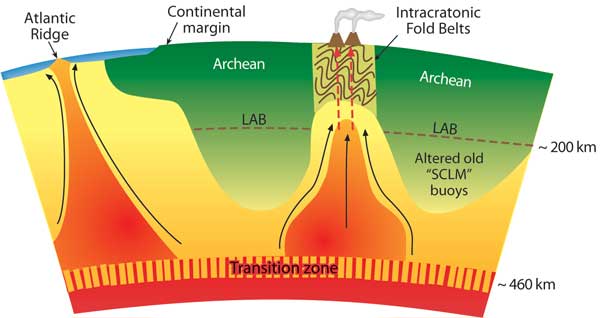
Figure 3. Cartoon indicating
how high-Vs (low-density), vertically coherent
regions extending to up to > 250
km could control convection pathways (modified
from O’Reilly
et al., 2009).
Ocean island basalt goechemical signatures
The
localised persistence of ancient SCLM beneath oceans
also provides a logical explanation for the “alphabet
soup” of mantle sources
created by geochemists to describe the isotopic
signatures of basalts (EM1, EM2, HIMU, DMM; Hofmann,
1997 and references therein; Figure 4). These
components are generally attributed to different
geochemical reservoirs within the convecting
mantle. However, all of these geochemical fingerprints
are found in lithospheric material and have been
well characterised in mantle xenolith studies
(e.g., Leeman, 1982; Wilson & Downes,
1991; Zhang et al.,
2001; Thompson
et al., 2005). If lithospheric volumes persist
to deep mantle levels (>150 km) in ocean basins,
then interaction with upwelling mantle plumes
can “contaminate” magmas and fluids
(Figure 3), imposing a range of isotopic and
trace-element signatures. A detailed examination
of the ocean-island database from the Atlantic
shows a strong correlation between “continental” signatures
(EM1, EM2, etc.) and the presence of high-velocity
blobs in the seismic tomography.
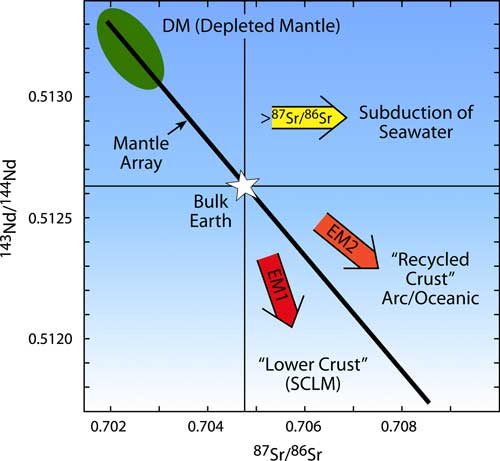
Figure 4. Isotopic components commonly observed
in basaltic magmas and their fields in Nd and
Sr isotopic space (Hofmann, 1997, and references
therein).
This model removes the requirement for hidden
source regions embedded within the convecting mantle.
Magma interaction with deep ancient SCLM roots
also provides a simple explanation for observations
such as Archean Re-depletion model ages in oceanic
basalts.
References
-
Begg,
G.C., Griffin, W.L., Natapov, L.M., O’Reilly,
S.Y., Grand, S.P., O’Neill, C.J., Hronsky,
J.M.A., Poudjom Djomani, Y., Swain, C.J., Deen,
T. and Bowden, P. 2009. The lithospheric architecture
of Africa: Seismic tomography, mantle petrology
and tectonic evolution. Geosphere, 5,
23-50.
-
Coltorti,
M., Bonadiman, C., O’Reilly,
S.Y., Griffin W.L. and Pearson, N.J. 2010.
Buoyant ancient continental mantle embedded
in oceanic lithosphere (Sal Island, Cape Verde
Archipelago). Lithos,
120, 1-2, 223-233.
-
Grand, S. 2002. Mantle shear-wave tomography
and the fate of subducted slabs. Philisophical
Transactions Royal Society of London,
A360, 2475-2491.
-
-
-
Hofmann, A.W. 1997. Mantle geochemistry: the
message from oceanic volcanism. Nature,
385, 219-229.
-
Korhonen, J.V., Farihead,
J.D., Hamoudi, M., Hemant, K., Lesur, V., Mandea,
M., Maus, S., Purucker, M., Ravat, D., Sazonova,
T. and Thébault,
E. 2007. Magnetic Anomaly Map of the World. Commission
for the Geological Map of the World.
-
Leeman, W.P. 1982. Tectonic and magmatic significance
of strontium isotopic variations in Cenozoic
volcanic rocks from the western United States. Geological
Society of America Bulletin, 93, 487-503.
-
O'Reilly,
S.Y., Zhang, M., Griffin, W.L., Begg, G.
and Hronsky, J. 2009. Ultradeep continental
roots and their oceanic remnants: a solution
to the geochemical "mantle reservoir" problem? Lithos,
112, S2, 1043-1054. 
-
Thompson, R.N., Ottley, C.J., Smith, P.M., Pearson,
D.G., Dickin, A.P., Morrison, M.A., Leat, P. T.
and Gibson, S.A. 2005. Source of the Quaternary
alkalic basalts, picrites and basanites of the
Potrillo Volcanic Field, New Mexico, USA lithosphere
or convecting mantle? Journal of Petrology, 46,
1603-1643.
-
Wilson, M. and Downes, H. 1991. Tertiary-Quaternary
Extension-Related Alkaline Magmatism in Western
and Central Europe. Journal of Petrology,
32, 811-849.
-
Zhang, M., Stephenson, P.J., O'Reilly, S.Y.,
McCulloch, M.T. and Norman, M. 2001. Petrogenesis
and geodynamic implications of late Cenozoic
basalts in North Queensland, Australia: trace-element
and Sr-Nd-Pb isotope evidence. Journal of Petrology,
42, 685-719.
last updated
26th November, 2010 |