|
Instability Migration (Delamination) and the Geological Record |
Tim Stern
Institute of Geophysics, Victoria University of Wellington, Wellington, New Zealand, tim.stern@vuw.ac.nz
Based on the paper: Stern, T., G. Houseman, M. Salmon, L. Evans, Instability of a lithospheric step beneath western Northy Island, New Zealand, Geology, 41, 423-426, 2013.
This paper uses the dimensionless variable approach to model a mantle instability using a finite deformation program developed by Greg Houseman and Lynn Evans. We apply this approach to an example where there is good geological evidence for a migrating surface uplift and subsidence associated with a putative mantle instability. We argue that the instability was initiated by a transtensional transform that ran through the central North Island of New Zealand in the Miocene.
Geological history: Importance in understanding instability development
The concept of delamination mantle instabilities and their role in orogenic belts has undergone a renaissance in the past 15 years or so. The initial idea largely grew from papers by Bird (1979) and Houseman et al. (1981), but in the past decade seismic methods have developed sufficiently to confirm that mantle “drips” beneath the lithosphere do exist in nature. While there have been many theoretical papers on modeling mantle instabilities, what is in short supply is clear evidence from the geological record for their occurrence. One possible reason is that many of the areas that have experienced mantle instability are elevated (e.g., the western USA) and have been stripped of their marine sedimentary record, or never had such a record in the first place.
The central North Island of New Zealand is well placed for such a study for several reasons:
- It was, and still is, mantled by a very large section of Miocene–Recent marine sediments;
- It has been subjected to both compression and extension in a back–arc setting for ~ 25 Ma; and
- The western North Island has been the focus of 40 years of oil exploration, which has yielded excellent seismic and well data.
In a country dominated by subduction like New Zealand, it is always tempting to seek an explanation for observations via subduction dynamics and classical plate tectonics. Three key observations alerted us, however, that something was different:
- The sudden uplift of the central North Island at about 5 Ma that extended over a dome-like area ~ 300 km in diameter (Figure 1);
- The sharp east-west boundary between the volcanoes of Mt. Ruapehu and Taranaki–the Taranaki-Ruapehu (TR) line discussed in Stern et al. (2013), across which there is rapid change in geophysical properties of the lithosphere; and
- The systematic north-to-south migration of basin depocentres from 12 Ma to the present through the western North Island (Stern et al., 2013).
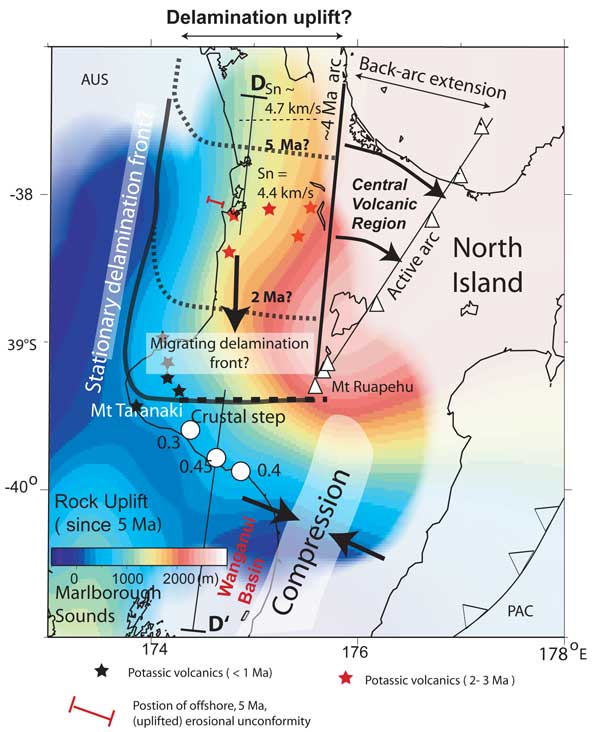
Figure 1: Map showing proposed area of migrating delamination for the western North Island of New Zealand. The delamination is proposed to have been initiated by a transtensional transform along the western boundary of the Central Volcanic Region, which would have rapidly juxtaposed thin- against thicker lithosphere (12- 0 Ma). The colours represent rock uplift since 5 Ma that can be dated by offshore unconformities seen in oil industry seismic data (Pulford & Stern, 2004). Present day uplift is shown by white circles (rates in mm/y) along coast southeast of Mt. Taranaki and subsidence is expressed by the drowning of the Marlborough Sounds in the last 1 Ma. Note southward migration of potassic volcanism. The TR line runs between Mt. Taranaki and Mt. Ruapehu beneath which there is an active zone of seismicity to a depth of 45 km. The Moho also shows a step increase in depth of 7-10 km with thin crust to the north (Salmon et al., 2011).
A decade ago we carried out a detailed analysis of the geophysical properties across the TR line and found that the Moho had a vertical step in it. Beneath the step unusual seismicity occurs to a depth of ~ 40-45 km i.e. in upper mantle (Salmon et al., 2011). To the north of the step, on the thin-crust side, receiver functions show a flat and extremely strong conversion at a depth of 25 km. This is an unusually shallow Moho for an area where the elevation is ~ 300 m on average and suggests upper mantle buoyancy. Thus the TR line presents a major and, because of a persistent seismicity cloud around the Moho step, an unstable boundary between thick crust/lithosphere to the south and thin crust/lithosphere to the north.
Linked to the TR line is the 300-km-long progression of sedimentary depocenters from 12 Ma to present down through the western North Island. These depocenters pass through the TR line and define the path of a geological “wave” with progressive subsidence and sedimentary onlap to the south coupled with uplift and erosion to the north. The progression is systematic with accelerated movement in the past 3 Ma (Stern et al., 2013). Note that the north-south azimuth of this migration is almost at right angles to the estimated plate-dip direction over the same period. Thus, although the TR line and sedimentary basin migration is no doubt indirectly related to the subduction history of the Pacific plate beneath the North Island of New Zealand, the direct processes appear to result from restructuring within the crust and mantle of the overriding Australian plate.
Numerical experiments on step-driven mantle Instabilities
We explored possible mantle instability models that could explain both the phenomena of the TR line and the migration of the sedimentary depocenters. Other constraints on our instability model were that it had to remove almost 100% of the mantle lithosphere, attenuate the crust, and that it needs to predict a migrating and coupled zone of uplift-subsidence. We propose an edge-instability was created by the rapid juxtaposition of lithospheres of different thickness along a transtensional, transform fault. It turns out that such an instability rapidly morphs into a migrating instability with the requisite thinning of both crust and mantle at its trailing edge (Stern et al., 2013). We use the finite element code basil (Houseman et al., 2008; Neil & Houseman, 1999) where the analysis is done with a series of dimensionless variables. The advantage of this scheme is the key variables are reduced to about 3 dimensionless ratios:
- crust-to-mantle viscosity (η’)
- crust-to-mantle lithosphere thickness (m’)
- dimensionless time given by: t’ = t(ΔρgL/2ηm)
where Δρ = the density contrast between mantle lithosphere and asthenosphere, t is regular time, L = the thickness of lithosphere, and ηm is the reference mantle density. Another dimensionless variable that had some influence on whether or not the instabilty will start is the ratio of the height of the initial step to the lithospheric thickness, d’ = d/L, where d = step height.
We also found the instability migrated faster and further when we had a low-viscosity lower-crustal layer (a “delamination effect”). So this led to another dimensionless ratio of η’’ = ηlc/ηm, where ηlc = viscosity of the lower crust.
Our results are summarized in Figure 2. Here there are a series of curves for different dimensionless time intervals. This is a two-dimensional approach to what is clearly a three-dimensional effect. We are currently working on the three-dimensional problem.
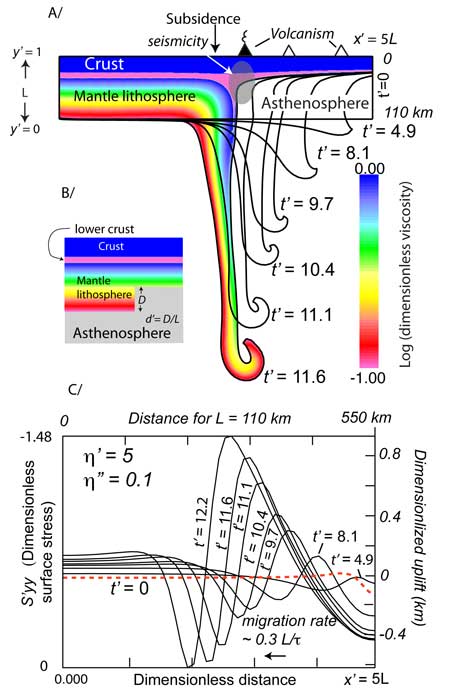
Figure 2: Panel A: Finite element model of an evolving step-instability in dimensionless time t’. In the North Island setting the right hand end is to the North. t’ would be millions of years if L= 110 km and the upper mantle viscosity is 5 x 1020 Pa s. The instability effectively peels back and travels ~ 300 km over 10 Ma (~ 30 mm/a). Panel B: The initial condition step to initiate the instability. Panel C: The predicted uplift and subsidence of the solid surface through time. η’ = 5 and η’’ =0.1 refer to the viscosity contrast of the upper crust to the upper mantle, and the lower crust to upper mantle respectively. More details are given by Stern et al. (2013).
References
-
Bird, P., Continental delamination and the Colorado Plateau. J. Geophys. Res., 84, 7561–7571, (1979).
-
Houseman, G., McKenzie, D. & Molnar, P., Convective instability of a thickened boundary layer and its relevance for the thermal evolution of continental convergent belts. J. Geophys. Res., 86, 6115-6132, (1981).
-
Houseman, G.A., Barr T.D., Evans, L.A, Basil: stress and deformation in a viscous material (Ch 3.8, pp 77-85) in Microdynamics Simulation, ed. Bons, P.D., Koehn, D., Jessell, M.W., Lecture Notes in Earth Sciences, 106, Springer. ISBN: 978-3-540-25522-255, (2008).
-
Levander, A. et al., Continuing Colorado Plateau uplift by delamination style convective lithospheric downwelling. Nature, 472, 461-465, doi:10.1038/nature10001, (2011).
-
Neil, E.A. & Houseman, G.A., Rayleigh-Taylor instability of the upper mantle and its role in intraplate orogeny. Geophys. J. Int., 138, 89-107, (1999).
-
Salmon, M., Stern, T. & Savage, M. A major step in the continental Moho and its geodynamic consequences: the Taranaki-Ruapehu line, New Zealand. Geophys. J. Int., 186, 22-44, doi:10.1111/j.1365-246X.2011.05035.x, (2011).
-
Stern, T., G. Houseman, M. Salmon, L. Evans, Instability of a lithospheric step beneath western North Island, New Zealand, Geology, 41, 423-426, (2013).
-
Pulford, A. & Stern, T.A. Pliocene exhumation and landscape evolution of central North Island, New Zealand: the role of the upper mantle. J. Geophys. Res., 109, doi10.1029/2003jf000046, (2004).
last updated 14th April, 2014 |