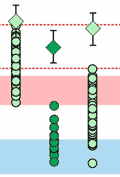 |
Magmatism in the North Atlantic Igneous Province; mantle temperatures, rifting and geodynamics |
Malcolm J. Hole1
& James H. Natland2
1Department of Geology and Geophysics, University of Aberdeen, Aberdeen AB243UE, UK; m.j.hole@abdn.ac.uk
2RSMAS/MGS University of Miami, Miami FL 33149 USA; jnatland@msn.com
This webpage is a summary of Hole, M. J., and J. H. Natland (2019), Magmatism in the North Atlantic Igneous Province; mantle temperatures, rifting and geodynamics, Earth-Science Reviews, in press.
A question of fundamental importance to all models of melt extraction from the mantle, including the origin of "hot spot" magmatism and Large Igneous Provinces, is the relative roles of source composition, source temperature, and volatiles. Studying magmas and their component minerals can inform us about magmatic temperatures and, by inference, the temperature structure of the mantle. These are key considerations in our fundamental view of the dynamics of the mantle (Figure 1).
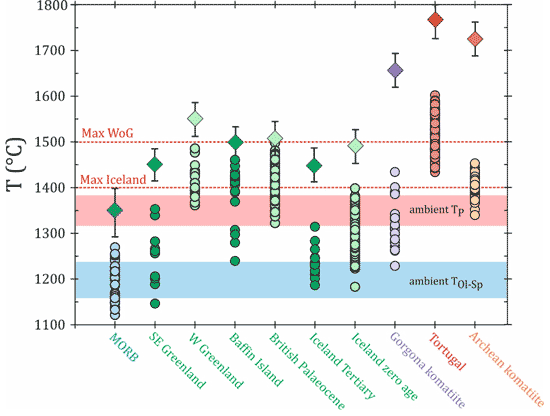
Figure 1. Summary of global maximum petrological estimates of TP (diamonds ±40°C) and ranges of olivine-spinel equilibrium crystallization temperatures (TOl-Sp) for magnesian olivine (dots with ranges for individual samples). The lower light-blue shaded region represents the range of TOl- for olivine which crystallized from near-primary magmas formed at ambient TP~1350±40°C (upper pink-shaded region). The horizontal pecked lines represent the maximum estimated TP for Iceland and West of Greenland (WoG; Disko Island, Baffin Island) from Hole & Natland (2019). Data sources for TOl-Sp; MORB, Gorgona komatiite and Archean komatiite, Coogan et al. (2014); British Palaeocene, Baffin Island, West Greenland (Disko Island), Coogan et al. (2014), Spice et al. (2016); Iceland, Matthews et al. (2016), Spice et al. (2016); Tortugal Trela et al. (2017). Petrological estimates from Herzberg and Asimow (2008; 2015), Hole (2015), Hole and Millett (2016) and Trela et al. (2017).
A plethora of estimates of mantle potential temperature (TP) for the North Atlantic Igneous Province (NAIP) exist in the literature. We critically appraised the methodology used to derive these TP estimates and re-evaluated the results. Methods for making temperature estimates fall into two broad categories; 1. melt-mineral equilibria; and 2. petrological methods using estimates of a model primary magma composition. These may be summarized as follows.
- Melt-mineral equilibria.
- Equilibrium between olivine and melt yields good estimates of crystallization temperature of olivine. However, converting crystallization temperature to TP is problematical because it requires knowledge of the extent and pressure of melting. Consequently, olivine-melt equilibria allow only relative temperatures to be estimated.
- Olivine–spinel–melt temperatures (TOl-Sp or "aluminium in olivine"; Coogan et al., 2014) require equilibrium to be have been attained between olivine and coexisting Cr-spinel, a situation that is difficult to ascertain. Independent estimates of spinel crystallization temperatures using the SPINMELT-2.0 routine of Nikolaev et al. (2018) suggest that published TOl-Sp may be up to 100°C too high.
- Petrological methods for estimating primary magma compositions.
- Published temperature estimates involving calculating model primary magmas using olivine addition to pillow-lava glasses are unreliable because host glasses formed along the liquid+olivine+plagioclase cotectic and not just the olivine liquidus. This results in significant over-estimates of TP for model primary magmas.
- Petrological models tend to over-estimate TP in picrites containing appreciable accumulative olivine. Importantly, magma-chamber processes can generate picritic lavas containing only magnesian olivine, but picrites alone do not require high mantle temperatures.
- Applying the primary magma model PRIMELT3 (Herzberg & Asimow, 2015) to selected aphyric lavas from West Greenland, which do not have accumulated olivine, suggest a maximum TP ~1500°C. Petrological models for Icelandic glasses suggest a maximum TP ~1450°C (Figure 1) which may be consistent with olivine melt and olivine-spinel equilibration temperatures but only in certain volcanic centres. This may be compared with the generally accepted TP of MORB source of 1350±40°C, giving maximum temperature anomalies of ~100°C for Iceland and 150°C for West Greenland picrites.
- Petrological modelling tools such as PRIMELT3 assume melting of dry peridotite. Melting of damp peridotite (~200 ppm H2O) reduces TP by about 50°C. Melting in the presence of CO2 has a similar effect.
Isotopic data demonstrate that the NAIP mantle was lithologically and chemically heterogeneous and had a hybrid pyroxenite-peridotite lithology, the pyroxenite component being derived from recycling of subducted slabs. However, there is no necessity for the subducted slabs to have been recycled to the core-mantle boundary. Pyroxenite could have been derived from Caledonian-aged slabs that also hosted helium with high 3He/4He within the shallow mantle, which was inherited by Palaeocene or young melts. The pyroxenite component was more readily fusible than the peridotite component under the same P-T conditions, allowing variations in melt productivity throughout the province. Melting of lithologically variable mantle is consistent with observed radiogenic isotope variability in Icelandic basalts and related trace-element variations throughout the NAIP.
Current models for magmatism in Iceland are partly constrained by the thickness of new crust that can be generated by decompression melting of peridotite or a mixed lithological source (e.g., Shorttle et al., 2013; 2014; Matthews et al., 2016; Lambart, 2017). Darbyshire et al. (2000) suggest crustal thicknesses of ~20 km for the northern half of the Northern Volcanic Zone (NVZ) and thicker crust (24-42 km) elsewhere in central and east Iceland. The thickest crust (42 km) is found in central Iceland (Foulger et al., 2003).
Shorttle et al. (2014) and Lambart (2017) argued that for a mixed pyroxenite–peridotite lithology at ~1480°C passive plate spreading alone could generate only the ~20 km of crust beneath coastal areas of Iceland and the thick crust under central Iceland was strongly affected by enhanced melt production caused by plume-driven upwelling. Nevertheless, melt production can only be accounted for by either decompression melting or release of pre-existing liquid, and any model, plume or otherwise, needs to account for the volume produced specifically in these terms.
A viable explanation for the observations is that the thick crust under central Iceland is formed by ~20 km of new, igneous crust emplaced on top of pre-existing crust of the same thickness. Both Foulger (2006) and Torsvik et al. (2015) concluded that continental crust beneath southeast Iceland comprises an extension of the ~350-km-long and 70-km-wide Jan Mayen Microplate Complex. If this is correct, any model for crustal generation in Iceland’s rift zones need only account for a maximum of 20 km of magmatic productivity and does not require short-wavelength, extreme variability in plume buoyancy flux to explain larger crustal thicknesses.
Local extensional tectonism resulting from plate boundary forces represents the most important control on the distribution of magmatism in the NAIP, with each period of magmatism being related to its own, local rifting event. Prior to continental break-up, continental insulation elevated local mantle temperatures, and extension above this internally heated mantle drove melting. Because, unlike the mantle plume model, internal heating does not provide a continual flux of heat, magmatism at any one location waned as melt was generated and the mantle subsequently cooled at each location.
References
-
Coogan, L.A., Saunders, A.D., Wilson, R.N., 2014. Aluminium-in-olivine thermometry of primitive basalts: Evidence of an anomalously hot mantle source for large igneous provinces. Chem. Geol. 368, 1-10.
-
Courtillot, V., Davaille, A., Besse, J.,Stock, J., 2003. Three distinct types of hotspots in the Earth’s mantle. Earth Planet. Sci. Lett. 205, 295-308
-
Darbyshire, F.A., Priestley, K.F., White, R.S., Stefansson, R., Gunmundsson, G.B., Jakobsdottir, S.S., 2000. Crustal structure of central and northern Iceland from analysis of teleseismic receiver functions. Geophys. J. Int. 143, 163-184.
-
Foulger, G.R., 2006. Older crust underlies Iceland. Geophys J. Int. 165, 672-676.
-
Foulger, G., 2012. Are ‘hot spots’ hot? J. Geodynamics 58, 1-28.
-
Foulger, G. R., Z. Du, and B. R. Julian (2003), Icelandic-type crust, Geophys. J. Int., 155, 567-590.
-
Herzberg, C., Asimow, P.D., 2008. Petrology of some oceanic island basalts: PRIMELT2.XLS software for primary magma calculation. Geochem. Geophys. Geosys. 9. Doi: 10.1029/2008GC002057.
-
Herzberg, C., Asimow, P.D., 2015. PRIMELT3 MEGA.XLSM software for primary magma calculation: Peridotite primary magma MgO contents from the liquidus to the solidus. Geochem. Geophys. Geosys. 16, 563-578.
-
Hole M.J., 2015. The generation of continental flood basalt from decompression melting of internally heated mantle. Geology, 43, 311-314.
-
Hole, M.J., Millett, J.M., 2016. Controls of mantle potential temperature and lithospheric thickness on magmatism in the North Atlantic Igneous Province. J. Petrol. 57, 417-436.
-
Lambart, S. 2017. No direct contribution of recycled crust in Icelandic basalts. Geochem. Persp. Lett. 4, 7-12. doi: 10.7185/geochemlet.1728
-
Matthews, S., Shorttle, O., Maclennan, J., 2016. The temperature of the Icelandic mantle from olivine-spinel aluminum exchange thermometry. Geochem. Geophys. Geosys. 17, 4725-4752. doi:10.1002/2016GC006497.
-
Nikolaev, G.G. Ariskin, A.A. Barmina, G.S. 2018. SPINMELT-2.0: Simulation of Spinel–Melt Equilibrium in Basaltic Systems under Pressures up to 15 Kbar: II. Description of the Program Package, the Topology of the Cr-spinel–Melt Model System, and Petrological Implications. Geochem. Inter. 56, 125-135. DOI: 10.1134/S001670291802005
-
Shorttle, O., Maclennan, J., Lambart, S., 2014. Quantifying lithological variability in the mantle. Earth Planet. Sci. Lett. 395, 24-40.
-
Shorttle, O., Maclennan, J., Piotrowski, A.M., 2013. Geochemical provincialism in the Iceland plume. Geochim. Cosmochim. Acta. 122, 363-397. DOI: 10.1016/j.gca.2013.08.032
-
Spice, H.E., Fitton, J.G., Kirstein, L.A., 2016. Temperature fluctuation of the Iceland mantle plume through time. Geochem. Geophys. Geosys. 17, 234-254.
-
Torsvik, T.H., Amundsen, H.E.F., Trønnes, R.G., Doubrovine, P.V., Gaina, K., Kusznir, N.J., Steinberger, B., Corfu, F., Ashwal, L.D., Griffin, W.L., Werner, S.C., Jamtveit, B., 2015. Continental crust beneath southeast Iceland. Proc. Nat. Acad. Sci. USA 112, E1818-1827.
-
Trela, J., Gazel, E., Sobolev, A.V., Moore, L., Bizimis, M., Jicha, B., Batanova, G., 2017. The hottest lavas of the Phanerozoic and the survival of deep Archean reservoirs. Nature Geoci. 10, 451-457. DOI: 10.1038/NGEO2954
last updated 1st December, 2019 |