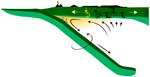 |
Mantle Dynamics Beneath the Pacific Northwest and the Generation of Voluminous Back-arc Volcanism |
Maureen D. Long1, Christy B. Till2,3, Kelsey A. Druken4,5, Richard W. Carlson5, Lara S. Wagner6, Matthew J. Fouch5, David E. James5, Timothy L. Grove2, Nicholas Schmerr5,7, Chris Kincaid4
1Department of Geology and Geophysics, Yale University
2Department of Earth, Atmospheric, and Planetary Sciences, Massachusetts Institute of Technology
3Now at United States Geological Survey, Menlo Park
4Graduate School of Oceanography, University of Rhode Island
5Department of Terrestrial Magnetism, Carnegie Institution of Washington
6Department of Geological Sciences, University of North Carolina at Chapel Hill
7Planetary Geodynamics Laboratory, NASA Goddard Space Flight Center
This webpage draws from the paper: Long, M.D.; Till, C.B.; Druken, . A.; Carlson, R.W.; Wagner, L. S.; Fouch, M.J.; James, D.E.; Grove, .L.; Schmerr, N.; Kincaid, ., Mantle dynamics beneath the Pacific Northwest and the generation of voluminous back-arc volcanism, Geochem. Geophys. Geosyst., 13, Q0AN01, 2012.
Summary
The Pacific Northwest (PNW) has a complex tectonic history and over the past ~17 Ma has played host to several major episodes of intraplate volcanism. These events include the Steens/Columbia River flood basalts (CRB) and the striking spatiotemporal trends of the Yellowstone/Snake River Plain (Y/SRP) and High Lava Plains (HLP). Several different models have been proposed to explain these features, which variously invoke the putative Yellowstone plume, rollback and steepening of the Cascadia slab, extensional processes in the lithosphere, or a combination of these. Here we integrate seismologic, geodynamic, geochemical, and petrologic results from the multidisciplinary HLP project and associated analyses of EarthScope USArray seismic data to propose a conceptual model for post-20 Ma mantle dynamics beneath the PNW and the relationships between mantle flow and surface tectonomagmatic activity. This model invokes rollback subduction as the main driver for mantle flow beneath the PNW beginning at ~20 Ma. A major pulse of upwelling due to slab rollback, upper plate extension, and consequent melting produced the Steens/CRB volcanism. Continuing trench migration enabled mantle upwelling and hot, shallow melting beneath the HLP. Additional buoyant mantle upwelling is required to explain Y/SRP volcanism, but subduction-related processes may well have played a primary role in controlling its timing and location, and this upwelling likely continues today in some form. This model makes predictions that are broadly consistent with seismic observations, geodynamic modeling experiments, and petrology and geochemistry.
Background
Despite the long history of work on the post-20 Ma tectonomagmatism of the PNW, a consensus about what processes have controlled the timing and location of volcanic activity has not been forthcoming. In particular, whether or not a mantle plume is required to explain the volcanism is debated [e.g., Humphreys et al., 2000; Christiansen et al., 2002; Hooper et al., 2007; Smith et al., 2009; Obrebski et al., 2010; James et al., 2011; Schmandt et al., 2012; Fouch, 2012]. Here we discuss results from the recent High Lava Plains Project, combining petrology, geodynamical modeling, and broadband seismology components. The region encompasses the subduction of the Juan de Fuca plate to the NE and the rollback of the trench at a rate of ~35 mm/yr, as well as events such as a phase of accelerated slab rollback that began at about 20 Ma [Atwater and Stock, 1998].
Volcanism
Miocene to present volcanic activity in the PNW can be divided into three episodes of compositionally distinct volcanism (Figure 1): distributed calc-alkaline volcanism associated with the subduction of the Farallon plate (~36-18 Ma), the flood basalt era (~16.6-10.5 Ma), and HLP/SRP volcanism (~12 Ma-present). Eruption of the flood basalts initiated along NS-trending dikes near Steens Mountain in SE Oregon and migrated N to the Chief Joseph and Monument dike swarms in N Oregon [Camp and Ross, 2004]. By ~12 Ma, silicic volcanism had begun a spatiotemporal migration both to the NW along the HLP [Jordan et al., 2004] and to the NE along the SRP [Pierce and Morgan, 1992]. The present-day termination of these silicic volcanic tracks can be found at Yellowstone in the YSRP, and between Newberry and Three Sisters volcanoes in the HLP. Basaltic volcanism has been widespread in both space and time in both regions over the past ~10 Ma.
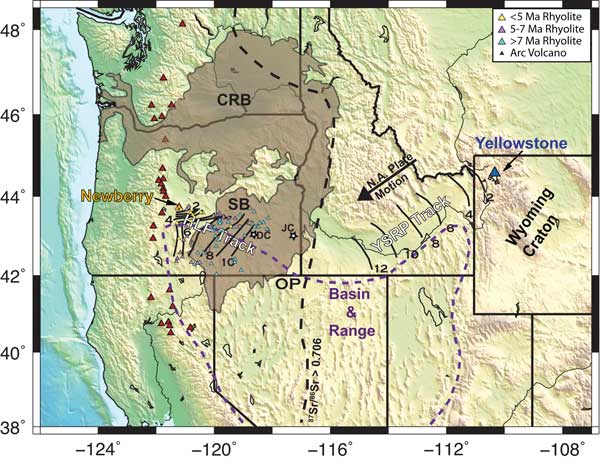
Figure 1: Map of the geologic and tectonic setting of the Pacific Northwest. Gray shaded region: the Steens flood basalts (SB) and Columbia River flood basalts (CRB). Red triangles: Cascades arc volcanoes. Young (<12 Ma) volcanism in the High Lava Plains (HLP) region is shown; blue, purple, and yellow triangles: HLP rhyolites. Colors indicate the age progression. White stars: Jordan Craters (JC) and Diamond Crater (DC). Black lines: age contours for progressive rhyolitic volcanism in the HLP and Snake River Plain (SRP) volcanic tracks. Dashed purple line: the approximate northern extent of Basin and Range extension. Dashed black line: 87Sr/86Sr = 0.706.
Thermobarometric calculations for young primitive basaltic lavas from across the HLP suggest their minimum depth of equilibration with the asthenospheric mantle occurred at temperatures of ~1180-1380°C at 37-59 km depth. The plagioclase and spinel lherzolite thermometer and barometer of Till et al. [2012] was used because of its suitability for modeling melting of variably depleted and metasomatized mantle. The shallowest depths of equilibration for samples across the HLP are immediately below the present-day Moho and occur at temperatures consistent with commonly assumed mantle potential temperatures and adiabatic gradients. The petrologic constraints point to a model where melting was driven by corner flow (i.e., upwelling in the asthenospheric mantle due to plate-driven flow processes), rather than from deep, anomalously hot mantle e.g., from a mantle plume. The comparison between primitive Quaternary HLP basalt equilibration conditions and geophysics implies that the Moho and the lithosphere-asthenosphere boundary lay at approximately the same depth for at least the last several million years.
Seismology
Seismic anisotropy suggests strong, uniform deformation in the upper mantle beneath the HLP. Delay times beneath the HLP are large (up to ~2.5 s) and fast directions are uniformly EW. This is evidence for uniform, EW mantle flow [Long et al., 2009]. To the E of the HLP, in W Montana and E Idaho, there is a transition from EW to NE-SW fast directions, roughly parallel to the absolute motion of the North American plate. Comparisons between laboratory geodynamic modeling and SKS splitting data found the rollback of the Cascadia slab likely results in focused mantle flow in the central part of the backarc parallel to the direction of rollback [Druken et al., 2011]. Our preferred model for upper mantle anisotropy beneath the PNW is that mantle flow beneath the HLP is largely controlled by slab rollback, while further to the E in the ESRP, the effect of rollback dies off, allowing a transition to North American Plate-driven flow. Complementary constraints on mantle dynamics can be obtained from transition zone (TZ) discontinuity topography. Beneath the HLP, there is evidence for locally lower temperatures associated with the penetration of the Juan de Fuca slab into the TZ [Eagar et al., 2010; Schmandt et al., 2012]. Along-strike variations suggest slab fragmentation at TZ depths. Schmandt et al. [2012] found evidence for higher temperatures in the TZ beneath Yellowstone from an upwards deflection by ~12-18 km of the 660 km discontinuity. They argued this is consistent with a plume-like upwelling.
Tomographic models of the PNW [e.g., Sigloch et al., 2008; Roth et al., 2008; Obrebski et al., 2010; Schmandt and Humphreys, 2010; James et al., 2011] reveal a well-imaged Juan de Fuca slab which manifests as a nearly continuous, higher-velocity anomaly down to the deep upper mantle (>300 km) with a southern edge extending along the direction of plate motion from the Mendocino triple junction across northernmost Nevada. There is apparent fragmentation of the slab in the upper mantle beneath Oregon [Obrebski et al., 2011], although this may be an imaging artifact [Roth et al., 2008]. A strong low-velocity anomaly underlies the entire eastern SRP to depths of ~150 km. A sub-horizontal branch of the old Farallon plate, orphaned from the descending plate by the northward migration of the triple junction, is imaged in the TZ directly beneath the SRP/Y track. Evidence for a continuous, low-velocity body beneath Yellowstone corresponding to a classical plume conduit is ambiguous, although there is a relatively weak region of low velocities that extend down to the TZ in a warped, sheet-like structure spatially connected with a prominent slab-gap anomaly in the TZ. Surface-wave tomography [e.g., Wagner et al., 2010] identifies relatively low S-wave velocities beneath the HLP and Newberry Volcano along with a low-velocity anomaly beneath the eastern SRP that deepens to at least ~175 km beneath Yellowstone.
Laboratory experiments
Idealized laboratory geodynamic experiments to study the combined effects of trench migration, slab steepening, and modest upper plate extension on the mantle flow field [Druken et al., 2011] reveal that the initiation of rollback and extension induces a major pulse of upwelling in the mantle wedge. Vertical velocities within 3 Ma of rollback and extension initiation are greatest beneath the center of the slab and less near the edge. Such a phase of rapid upwelling may represent the source of the melting associated with the Steens/CRB flood basalts. Temperature measurements along the “trench” after ~20 Ma of rollback and extension display a dramatic temperature increase toward the slab centerline, with equivalent scaled mantle anomalies that are similar to “hot spot” temperatures [Courtier et al., 2007]. The increase in temperature in the center of the slab migrates trench-ward with time, providing a mechanism for the time-progressive rhyolitic volcanism in the HLP trend.
Our preferred model
Our preferred model for PNW mantle dynamics is driven by four robust observations: 1) seismic anisotropy argues for well-organized contemporary mantle flow beneath the HLP in an E-W direction, likely controlled by slab rollback, 2) tomographic models argue for very low uppermost mantle velocities beneath both the HLP and the SRP, with a fragmented slab in the TZ, 3) geodynamic modeling suggests that the phase of rapid slab rollback beginning at ~20 Ma is associated with a large pulse of mantle upwelling that increased in magnitude to the north, with continued broad upwelling beneath the HLP enhanced by rollback-controlled mantle flow, and 4) the modern lithosphere beneath the HLP is thin, and petrologic models indicate that HLP basalts are produced by shallow decompression melting near the base of the seismically imaged crust.
Our model (Figure 2) proposes that the phase of rapid slab rollback and steepening that initiated at ~20 Ma enabled a large pulse of upwelling. This facilitated rapid thinning of the lithosphere as well as extensive mantle melting, representing the source of the flood basalts, with the surface expression of the flood basalts likely controlled by preexisting structures in the lithosphere. As the subduction system continued to evolve post-17 Ma, the upper mantle beneath the HLP experienced a reorganization of the flow field to the rollback-controlled mantle flow we infer today. Continued upwelling beneath the HLP, in combination with ongoing modest extension of the upper plate facilitated lithospheric thinning and the generation of small volumes of melt at shallow depth beneath the HLP. The westward migration of HLP volcanism is primarily a result of the plate-induced flow field, with the concentration of volcanism along the HLP likely controlled at least in part by lithospheric structures such as the Brothers Fault Zone.
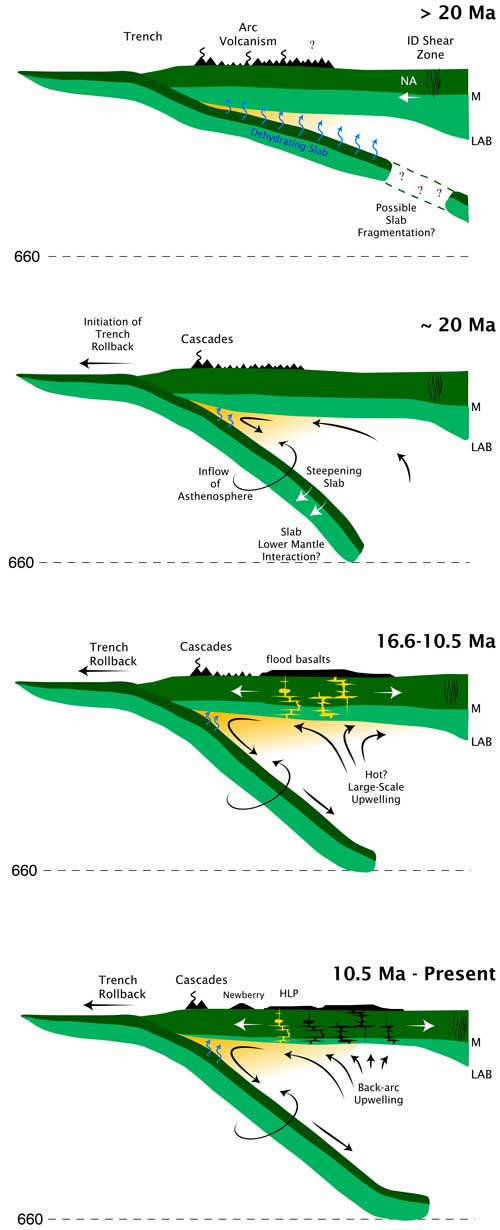
Figure 2: Schematic cartoon of the main model features for four times: pre-20 Ma, ~20 Ma (initiation of rapid rollback), ~16.5-10.5 Ma (flood basalt era), and 10.5 Ma-present (HLP/SRP era). Because of the uncertainty surrounding the origin of the upwelling responsible for Y/SRP volcanism, the cartoon focuses on flood basalt eruptions and subsequent HLP volcanism.
This model is consistent with petrologic, geodynamic, and seismologic observations beneath the HLP, but it does not provide an obvious explanation for the Y/SRP trend to the east. One possibility is the scenario discussed by James et al. [2011], which invokes mantle upwelling around the tip and edge of the sinking remnant slab driven by slab rollback. Alternatively, the deep mantle plume model has been advocated by many workers [e.g., Richards et al., 1989; Camp and Hanan, 2008; Obrebski et al., 2010].
While the work described here does not rule out a deep mantle plume origin for Yellowstone, a plausible alternative is that mantle dynamics beneath the PNW are largely controlled by subduction-related processes. The initiation of slab rollback at ~20 Ma (and possibly the fragmentation of the Farallon slab) facilitated upwelling of buoyant mantle that constitutes the mantle source of the SRP/Y magmas. The difference between the subduction-controlled buoyant mantle upwelling invoked here and the “plume” that is often invoked as the cause of SRP/Y volcanism may be at least partially a matter of semantics. If the definition of “plume” is broad enough to include an uppermost-mantle upwelling whose location and timing is controlled by slab fragmentation, then the SRP/Y volcanic trend may be called plume-related in the context of our model. Models for upwelling beneath the PNW in general and SRP/Y in particular must be consistent with known plate-driven processes, regardless of the possible presence of a separate, deep mantle plume.
References
-
Atwater, T.M., and J.M. Stock (1998), Pacific-North America plate tectonics of the Neogene southwestern United States: An update, Int. Geology Rev., 40, 375-402. [note: an animation of this plate reconstruction model can be found at http://emvc.geol.ucsb.edu/2_infopgs/ IP4WNACal/bNEPacWNoAmer.html]
-
Camp, V.E., and Hanan, B.B. (2008), A plume-triggered delamination origin for the Columbia River Basalt Group, Geosphere, 4, 480-495.
-
Camp, V.E., and M.E. Ross (2004), Mantle dynamics and genesis of mafic magmatism in the intermontane Pacific Northwest, J. Geophys. Res., 109, B08204, doi:10.1029/2003JB002838.
-
Christiansen, R.L., G.R. Foulger, and J.R. Evans (2002), Upper-mantle origin of the Yellowstone hotspot, Geol. Soc. Am. Bull., 114, 1245-1256.
-
Courtier, A.M., M.G. Jackson, J.F. Lawrence, Z. Wang, C.A. Lee, R. Halama, J.M. Warren, R. Workman, W. Xu, M.M. Hirschmann, A.M. Larson, S.R. Hart, C. Lithgow- Bertelloni, L. Stixrude, and W.Chen (2007), Correlation of seismic and petrologic thermometers suggests deep thermal anomalies beneath hotspots, Earth Planet. Sci. Lett., 264, 308–316.
-
Druken, K.A., M.D. Long, and C. Kincaid (2011), Patterns in seismic anisotropy driven by rollback subduction beneath the High Lava Plains, Geophys. Res. Lett., 38, L13310, doi:10.1029/2011GL047541.
-
Eagar, K.C., M.J. Fouch, and D.E. James (2010), Receiver function imaging of upper mantle complexity beneath the Pacific Northwest, United States, Earth Planet. Sci. Lett., 297, 141-153.
-
Eagar, K.C., M.J. Fouch, D.E. James, and R.W. Carlson (2011), Crustal structure beneath the High Lava Plains of eastern Oregon and surrounding regions from receiver function analysis, J. Geophys. Res., 116, B02313, doi:10.1029/2010JB007795.
-
Fouch, M.J. (2012), The Yellowstone hotspot: Plume or not?, Geology, 40, 479-480.
-
Hanson-Hedgecock, S., L.S. Wagner, M.J. Fouch, and D.E. James (2012), Constraints on the causes of mid-Miocene volcanism in the Pacific Northwest US from ambient noise tomography. Geophys. Res. Lett., 39, L05301, doi:10.1029/2012GL051108.
-
Hooper, P.R., V.E. Camp, S.P. Reidel, and M.E. Ross (2007) The origin of the Columbia River flood basalt province: Plume versus nonplume models, In: Foulger, G. R., Jurdy, D. M. (Eds.), Plates, plumes, and planetary processes, Geol. Soc. Am. Spec. Pap., 430, 635-668.
-
Humphreys, E.D., K.G. Deuker, D.L. Schutt, and R.B. Smith (2000), Beneath Yellowstone: Evaluating plume and nonplume models using teleseismic images of the upper mantle, GSA Today, 10, 1-7.
-
James, D.E., M.J. Fouch, R.W. Carlson, and J.B. Roth (2011), Slab fragmentation, edge flow and the origin of the Yellowstone hotspot track, Earth Planet. Sci. Lett., 311, 124-135.
-
Jordan, B.T., A.L. Grunder, R.A. Duncan, and A.L. Deino (2004), Geochronology of age-progressive volcanism of the Oregon High Lava Plains: implications for the plume interpretation of Yellowstone, J. Geophys. Res., 109, B10202, doi:10.1029/2003JB002776.
-
Long, M.D., H. Gao, A. Klaus, L.S. Wagner, M.J. Fouch, D.E. James, and E. Humphreys (2009), Shear wave splitting and the pattern of mantle flow beneath eastern Oregon, Earth Planet. Sci. Lett., 288, 359-369.
-
Obrebski, M., R.M. Allen, F. Pollitz, and S.-H. Hung (2011), Lithosphere-asthenosphere interaction beneath the western United States from the joint inversion of body-wave traveltimes and surface-wave phase velocities. Geophys. J. Int., 185, 1003-1021.
-
Obrebski, M., R.M. Allen, M. Xue, and S. Hung (2010), Slab-plume interaction beneath the Pacific Northwest, Geophys. Res. Lett., 37, L14305, doi:10.1029/2010GL043489.
-
Pierce, K.L., and L.A. Morgan (1992), The track of the Yellowstone hot spot: Volcanism, faulting and uplift, Geol. Soc. Am. Mem., 179, 1-53.
-
Richards, M., R.A. Duncan, and V.E. Courtillot (1989), Flood basalts and hot-spot tracks: plume heads and tails, Science, 246, 103-107.
-
Roth, J.B., M.J. Fouch, D.E. James, and R.W. Carlson (2008), Three-dimensional seismic velocity structure of the northwestern United States, Geophys. Res. Lett., 35, L15304, doi:10.1029/2008GL03466.
-
Schmandt, B., K. Dueker, E. Humphreys, and S. Hansen (2012), Hot mantle upwelling across the 660 beneath Yellowstone, Earth Planet. Sci. Lett., 331-332, 224-236.
-
Schmandt, B., and E. Humphreys (2010), Complex subduction and small-scale convection revealed by body-wave tomography of the western United States upper mantle, Earth Planet. Sci. Lett., 297, 435-445.
-
Sigloch, K., N. McQuarrie, and G. Nolet (2008), Two-stage subduction history under North America inferred from finite-frequency tomography, Nat. Geosci., 1, 458-462.
-
Smith, R. B., M. Jordan, B. Steinberger, C. M. Puskas, J. Farrell, G. P. Waite, S. Husen, W.-L. Chang, and R. O’Connell (2009), Geodynamics of the Yellowstone hotspot and mantle plume: Seismic and GPS imaging, kinematics, and mantle flow, J. Volcanol. Geotherm. Res., 188, 26-56.
-
Till, C.B., T.L. Grove, and M.J. Krawczynski (2012), A melting model for variably depleted and enriched lherzolite in the plagioclase and spinel stability fields, J. Geophys. Res., 117, B06206, doi:10.1029/2011JB009044.
-
Wagner, L.S., D.W. Forsyth, M.J. Fouch, and D.E. James (2010), Detailed three-dimensional shear wave velocity structure of the northwestern United States from Rayleigh wave tomography, Earth Planet. Sci. Lett., 299, 273-284.
last updated 15th January, 2013 |