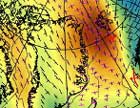 |
Intraplate stress & deformation: Changes in subduction zone linked to continental breakup |
Randell Stephenson1, Christian Schiffer2, Alexander L. Peace3, Søren B. Nielsen4 & Scott Jess5
1School of Geosciences, Geology and Geophysics, Meston Building, King’s College, University of Aberdeen, Aberdeen AB24 3UE, UK; r.stephenson@abdn.ac.uk
2Department of Earth Sciences, Uppsala University, Villavägen 16, 75236 Uppsala, Sweden; christian.schiffer@geo.uu.se
3School of Geography and Earth Sciences, McMaster University, 1280 Main Street West, Hamilton, Ontario, Canada L8S 4K1; peacea2@mcmaster.ca
4Department of Geoscience, Høegh-Guldbergs Gade 2, 8000 Aarhus C, Denmark; sbn@geo.au.dk
5Department of Geoscience, University of Calgary, 2500 University Dr. NW, Calgary, Alberta, Canada T2N 1N4; scott.jess@ucalgary.ca
This webpage is a summary of: Stephenson, R., C. Schiffer, A. Peace, S. Nielsen, and S. Jess (2020), Late Cretaceous-Cenozoic basin inversion and palaeostress fields in the North Atlantic-western Alpine-Tethys realm: Implications for intraplate tectonics, Earth-Science Reviews, in press.
A compilation of intraplate deformation structures, mainly as expressed as sedimentary basin inversion structures formed since the Late Cretaceous in the North Atlantic-western Alpine-Tethys realm, has been generalised and compared to palaeostress fields computed from geopotential (GP) energy gradients (e.g., Schiffer & Nielsen, 2016). Three key periods of tectonic transition and intraplate deformation were studied, the Late Cretaceous-Palaeocene, Eocene-Oligocene and Miocene. The results (Figure 1) are discussed in the context of a broad literature review with the aim of illuminating the geological record and deducing the causes and effects of intraplate tectonics at the plate- and global tectonic scales.
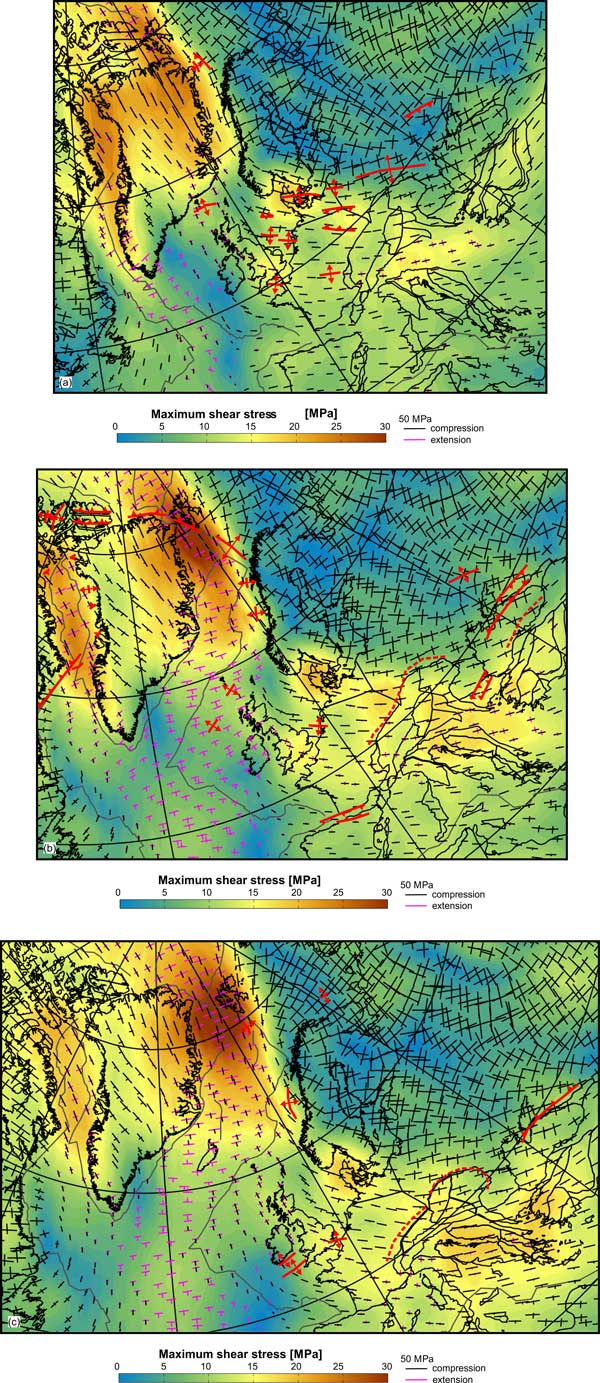
Figure 1: Generalised pattern of occurrence and orientation of basin inversion structures at 70 Ma (“Late Cretaceous- Palaeocene”), (b) 40 Ma (“Eocene-Oligocene”) and (c) 15 Ma (“Miocene”). Red schematic anticline and thrust symbols are superimposed on computed GP palaeostress results for 70 Ma, 40 Ma and 15 Ma, respectively, expressed as principal horizontal stresses (black lines representing compression and magenta extension) and the magnitude of the maximum shear stress (red-blue colour bar at the bottom). This is the difference between maximum (most extensional) and minimum (most compressional) of the principal horizontal stress components.
Conclusions build on pioneering work documenting European intraplate inversion done in the last decades (e.g., Ziegler, 1988; 1990; Kley, 2018) and considering its implications for tectonic driving mechanisms (e.g., Ziegler, 1987; Ziegler et al., 1995; Nielsen et al., 2007; Kley & Voight, 2008; Sandiford & Quigley, 2009). The main added value of this study is two-fold:
- the quantitative aspect of the present study, which incorporates models of GP palaeostress at times of intraplate deformation, and
- the stronger focus on Cenozoic intraplate deformation, not just in continental Europe but throughout the North Atlantic. This also benefitted from better constraints on the timing of deformation of key features such as the intraplate Eurekan (e.g., Stephenson et al., 2017) and Crimean-Caucasus (e.g., Sheremet et al., 2016) orogens.
The regional tectonic evolution of the study realm and its expression as intraplate deformation and palaeostress regimes for the three identified key periods of tectonic transition is briefly summarised as follows:
-
Late Cretaceous-Palaeocene:
-
Computed Late Cretaceous-Palaeocene principal horizontal stress directions are generally incompatible with the (smoothed) orientations of basin inversion structures (neglecting any role of inheritance or other locally derived perturbation) in west-central Europe. The widely-recognised implication is that an additional NW-SE orientated force derived from the Alpine-Tethys collisional plate boundary was involved in generating basin inversion at this time in west-central Europe.
-
Adria-Europe collision occurs (“Eo-Alpine” phase of Alpine Orogeny) and an unknown geodynamic process associated with the collisional/subductional plate boundary at this time produces the requisite “traumatic” intraplate compressional stresses linked to regionwide tectonic inversion in Central Europe.
-
The build-up and culmination of this “traumatic” event are interconnected with seafloor spreading kinematics in the central Atlantic Ocean, which record a period of very low or no Europe-Africa plate convergence in the latest Cretaceous-Palaeocene. This, in turn, leads to the onset of Laurasia break-up in the present-day North Atlantic Ocean with Greenland being detached from the Eurasian plate in the Palaeocene. This event terminates the renewal of traumatic stresses and leads to cessation of the Late Cretaceous-Palaeocene phase of intraplate deformation in west-central Europe.
-
The geologically sudden rupturing of Laurasia in the proto-North Atlantic can itself be considered a rapid plate boundary reconfiguration leading to a “traumatic” change in the stress fields of the contiguous plates. It promotes an acceleration of extension in the northern Labrador Sea-Baffin Bay leading to seafloor spreading in the latter.
- Eocene-Oligocene:
- There is a notable shift of locus of basin inversion from central-northern Europe in the Late Cretaceous-Palaeocene to the North Atlantic-Arctic realm and the periphery of the Alpine collision after the onset of North Atlantic break-up in the Eocene. In the former, this is accompanied by a significant change in the computed GP stress field around Greenland. Large extensional stresses in the Labrador Sea-Baffin Bay corridor decreased and initially weaker extension in the North Atlantic increased.
- Greenland acts as an independent plate and rotates, causing extensive basin inversion on its northern (Ellesmere Island-Svalbard) and western (Baffin Bay) margins. This includes formation of the intraplate Eurekan Orogen, which can be considered as a case of profound basin inversion (deforming and uplifting sedimentary strata deposited in this area after Palaeozoic orogenesis). The concurrent GP stress state is compatible with basin inversion in this realm at this time. This state of stress is a direct consequence of trauma in the Laurasian plate that led to North Atlantic break-up but is not itself overprinted by concurrent “traumatic stresses” as defined in this work.
- Northern Atlantic margins display local basin inversion promoted by the emerging North Atlantic ridge push, which is part of the evolving GP stress field in this area.
- Basin inversion still occurs immediately along the periphery of the Alpine-Tethys belt, most obviously linked with the nearby collision of the Eurasian plate with the Arabian plate (e.g., Crimea-Greater Caucasus intraplate orogen). It is mostly absent in west-central Europe, the traumatic stress field being generated at the Adria-Europe plate boundary having been relaxed and no longer renewed after the Palaeocene.
- Miocene:
- Seafloor spreading has ended in the Labrador Sea-Baffin Bay corridor and Greenland is now solidly part of the North American plate. All basin inversion processes surrounding the former “Greenland plate” have terminated.
- Basin inversion continues on the Norwegian margin and in the Barents Sea, as well as south of Ireland and Great Britain. It is likely linked to NE Atlantic ridge push and possibly developments related to the emerging Eurasia Basin of the Arctic Ocean.
- Miocene inversion occurs in southeastern Europe in the Black Sea and its margins but has not been recorded since, although there is active (transpressional) seismicity along the northern margin of the Black Sea.
The work allowed several general conclusions to be made regarding the geodynamics of intraplate deformation, as follows:
- Intraplate lithosphere stresses are those dominantly being generated by plate scale geopotential energy effects rather than collisional plate boundary effects and these can be considered to comprise the “background” intraplate stress field.
- Intraplate deformation, such as basin inversion, occurs – it goes without saying – whenever stress exceeds strength causing recordable permanent deformation at a suitable locus within the lithosphere. This can be dependent upon geologically rapid changes at plate boundaries (short compared to the lifespan of plate boundary zones themselves) that produce an additional “traumatic” component of intraplate stress.
- Intraplate deformation is promoted if/when the “traumatic” stresses constructively interfere with those derived from the background geopotential energy gradients and, further, the resulting causative, net stress field is favourably orientated with respect to pre-existing structures or other heterogeneities embedded within the lithosphere.
- “Traumatic” stresses are mainly an elastic response to the governing plate boundary processes. This requires that intraplate continental lithosphere is strong, with rigid-elastic properties, but that its elastic strength is finite and it can fail to produce permanent plastic deformation in the presence of a favourably orientated net stress field.
- Intraplate deformation, expressed as sedimentary basin inversion, occurs in a stress field related to lithosphere potential energy variations modified by tectonic forces produced at plate boundaries, means that it is a “top-down” (“plate” model rather than “plume” model) tectonic phenomenon.
References
-
Kley, J., 2018. Timing and spatial patterns of Cretaceous and Cenozoic inversion in the Southern Permian Basin. In: Kilhams, B., Kukla, P.A., Mazur, S., Mckie, T., Mijnlieff, H.F., Van Ojik, K. (eds), Mesozoic Resource Potential in the Southern Permian Basin. Geological Society, London, Special Publications, 469, 19-31. doi:10.1144/SP469.12
-
Kley, J., Voigt, T., 2008. Late Cretaceous intraplate thrusting in central Europe: effect of Africa-Iberia-Europe convergence, not Alpine collision. Geology, 36, 839-842.
-
Nielsen, S.B., Stephenson, R.A., Thomsen, E., 2007. Dynamics of Mid-Palaeocene North Atlantic rifting linked with European intra-plate deformations. Nature, 450, 1071-1074.
-
Sandiford, M., Quigley, M., 2009. TOPO-OZ: Insights into the various modes of intraplate deformation in the Australian continent. Tectonophysics, 474, 405-416.
-
Schiffer, C., Nielsen, S.B., 2016. Implications for anomalous mantle pressure and dynamic topography from lithospheric stress patterns in the North Atlantic Realm. Journal of Geodynamics, 98, 53-69. doi:10.1016/j.jog.2016.03.014
-
Sheremet, Ye., Sosson, M., Ratzov, G., Sydorenko, G., Voitsitskiy, Z., Yegorova, T., Gintov, O., Murovskaya, A., 2016. An offshore-onland transect across the north-eastern Black Sea basin (Crimean margin): Evidence of Palaeocene to Pliocene two-stage compression. Tectonophysics, 688, 84-100. doi:10.1016/j.tecto.2016.09.015
-
Stephenson, R., K. Piepjohn, C. Schiffer, W. von Gosen, G.N. Oakey, G. Anudu, 2017. Integrated crustal- geological cross-section of Ellesmere Island. In: Pease, V., Coakley, B. (eds). Circum-Arctic Lithosphere Evolution. Geological Society, London, Special Publications, 460, 7-17. doi.org/10.1144/SP460.12
-
Ziegler, P.A., 1987. Late Cretaceous and Cenozoic intra-plate compressional deformations in the Alpine foreland – geodynamic model. Tectonophysics, 137, 389-420.
-
Ziegler, P.A., 1988. Evolution of the Arctic-North Atlantic and western Tethys, American Association of Petroleum Geologists, Memoir, 43, 198 pp.
-
Ziegler, P.A., 1990. Geological Atlas of Western and Central Europe, 2nd Ed. Shell Internationale Petroleum Mij. B.V. and Geological Society of London Publishing House, Bath, England, 239 p.
-
Ziegler, P.A., Cloetingh, S., van Wees, J-D., 1995. Dynamics of intraplate compressional deformation: The Alpine foreland and other examples. Tectonophysics, 252, 7-59.
last updated 28th
June, 2020 |