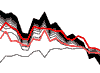 |
Recycled
crust controls contrasting source compositions
of Mesozoic and Cenozoic basalts in the North
China Craton |
Yongsheng
Liu1,2 Shan Gao2 Peter
B. Kelemen3 & Wenliang
Xu4
1State
Key Laboratory of Geological Processes & Mineral
Resources, Faculty of Earth Sciences, China University
of Geosciences, Wuhan 430074, China (yshliu@cug.edu.cn or yshliu@hotmail.com)
2State
Key Laboratory of Continental Dynamics, Department
of Geology, Northwest University, Xi’an 710069,
China
3Dept.
of Earth & Environmental Sciences, Columbia
University, NY 10964-8000, USA
4College
of Earth Sciences, Jilin University, Changchun 130061,
China
Click here to
download a PDF version of this webpage
Abstract
Mesozoic and Cenozoic basalts
of varying ages are widespread in the North China
Craton. Mesozoic basalts with ages > 110 Ma are
characterized by superchondritic Nb/Ta ratios (20.1 ± 0.3
(2σ))
and low Nb and Ta contents. Low Nb/Ta ratios (15.4 ± 0.3
(2σ)) and high Nb and Ta contents characterize
the < 110 Ma basalts. Both the > 110 Ma and < 110
Ma basalts have Fe/Mn ratios higher than basaltic melt
formed by partial melting of peridotite with the same
MgO and CaO contents. The > 110 Ma basalts have
island-arc-type trace-element patterns (i.e.,
depletion in Nb and Ta), whereas OIB-type trace-element
patterns (e.g., no depletion in Nb and Ta)
are characteristic of the < 110 Ma basalts. Based
on ΔFe/Mn values
for olivine, clinopyroxene, orthopyroxene and garnet,
high Fe/Mn ratios and negative correlations of Fe/Mn
with Yb (Y) of the < 110 Ma basalts suggest clinopyroxene/garnet-rich
mantle sources. The lower Fe/Mn ratios and positive
correlations of Fe/Mn with Y and Yb in the > 110
Ma basalts suggest orthopyroxene/garnet-rich mantle
sources.
Combining these data with Sr-Nd
isotopes, we present a conceptual model to explain
the contrasting geochemical features of the > 110 Ma and < 110
Ma basalts in a relatively consistent geodynamic framework.
Preferential melting of recycled ancient lower continental
crust during Mesozoic lithospheric thinning resulted
in:
-
peridotite-melt/fluid
reaction that formed the orthopyroxene/garnet-rich
mantle sources for the > 110
Ma basalts, and
-
peridotite + rutile-bearing
eclogite mixing that formed the clinopyroxene/garnet-rich
mantle sources for the < 110 Ma basalts.
Introduction
Mafic crustal materials, such as oceanic crust or
lower arc and continental crust, can be recycled back
into the convecting mantle, the former by subduction
and the latter by foundering or delamination (Gao
et al., 2004; Kay & Kay, 1991; Kelemen
et al., 2003; Lee et al., 2006; Lustrino,
2005; Rudnick, 1995; Sobolev et al.,
2007; Yuan et al., 2000). Then, because of
their lower melting points (at a given pressure) compared
to peridotite (Hirschmann & Stolper, 1996; Keshav
et al., 2004; Pertermann & Hirschmann,
2003b; Yaxley, 2000), they can melt preferentially
to produce a high-Si liquid (Rapp et al.,
1999; Yaxley & Green, 1998). As this melt
infiltrates peridotite, it converts olivine to pyroxene
to form garnet- and pyroxene-rich lenses/veins in the
peridotitic mantle (Liu et al.,
2005; Rapp et
al., 1999; Sobolev et al., 2005). Both
melt-peridotite reaction and foundering of eclogite
during subduction and/or delamination would produce
garnet pyroxenite reservoirs in the mantle and influence
the geochemistry of subsequent magmatism.
Case study of the North China Craton
Coupled with late Mesozoic and Cenozoic tectonothermal
reactivation, the ancient, cratonic mantle lithosphere
of the North China Craton (NCC) is believed to have
been removed from the base of the eastern block (Gao
et al., 2002; Griffin et al., 1998; Menzies
et al., 1993; Wu et al.,
2003; Xu, 2001). Mesozoic high-Mg# adakitic
lavas suggest that mafic lower continental crust had
been recycled into the convecting mantle during the
lithospheric thinning (Gao et al., 2004; Xu
et al., 2002).
Preferential partial melting of recycled mafic crustal
rocks in the mantle could lead to three types of mixing/reaction:
-
silicic melt-peridotite reaction by
equilibrium infiltration,
-
mechanical mixing of
peridotite and silicic melt by injection and freezing
of melts in cold lithospheric mantle, and
-
solid-state
mechanical mixing of peridotite and the eclogitic
residues after silicic melt extraction.
Mesozoic
and Cenozoic basalts of varying ages were studied
here to investigate these processes. The > 110
Ma and < 110 Ma basalts show contrasting trace
element patterns (Figure 1). The former were characterized
by island-arc-type trace element patterns, while
the later have ocean-island-basalt (OIB)-type trace-element
patterns.
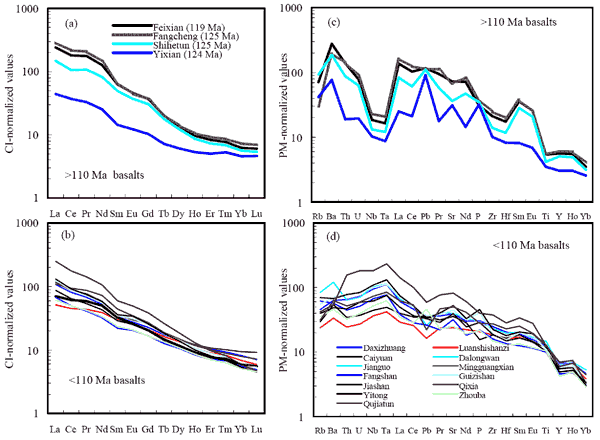
Figure 1 CI-normalized REE patterns
and PM-normalized trace-element patterns. Each line
represents a basalt locality. Chondrite (CI) values
from Taylor & McLennan (1985),
Primitive Mantle (PM) values from McDonough & Sun (1995). Click
here or on Figure for enlargment.
Contrasting Nb/Ta ratios of the > 110 Ma and < 110
Ma basalts
The > 110 Ma basalts are characterized
by superchondritic Nb/Ta ratios (20.1 ± 0.3
(2σ, n = 25)),
and low Nb (Ta) contents, while the < 110 Ma basalts
have lower Nb/Ta ratio (15.4 ± 0.3 (2σ,
n = 45)) and high Nb (Ta) contents (Figure 2). Rutile
strongly partitions Nb and Ta from melt (Δ(Nb,
Ta)rutile/melt >> 1),
and effectively fractionates Nb from Ta (ΔNb/ΔTarutile/melt
= 0.21 - 1.0; Foley et al., 2000; Green & Pearson,
1987; Klemme et al., 2005; Schmidt et
al., 2004; Stalder et al., 1998; Xiong
et al., 2005). The contrasting Nb/Ta ratios of
the > 110 Ma and < 110 Ma basalts could be attributed
to processes involving rutile.
The negative correlations between
MgO and Nb and Ta, expected for partial melting of
an ol + cpx + opx assemblage, indicate that both Nb
and Ta are highly incompatible during mantle melting
for the > 110 Ma basalts (Figure
2a). Therefore, their low Nb and Ta contents and high
Nb/Ta ratios suggest low-Nb and Ta mantle sources with
high Nb/Ta ratios. The positive correlations between
MgO and Nb and Ta for the < 110 Ma basalts indicate
that Nb and Ta are compatible during mantle melting
(Figure 2a). Their constant Nb/Ta ratios, regardless
of MgO contents, suggest that Nb/Ta ratios of the melts
were buffered by Nb (Ta)-rich Ti-Fe oxides (e.g.,
rutile and ilmenite; Figure 2b).
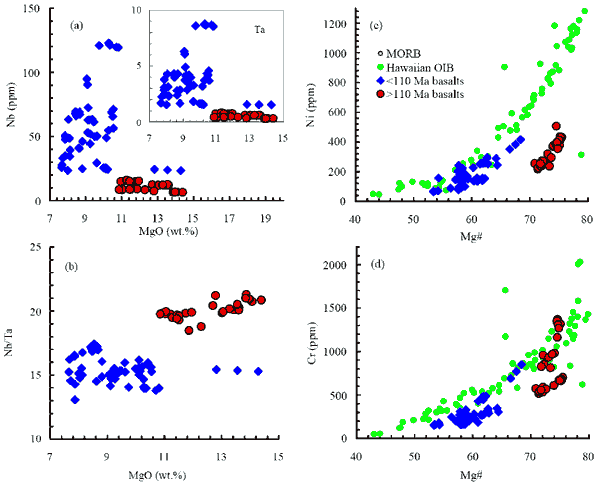
Figure 2 Plots of MgO-Nb (Ta) and
-Nb/Ta ratio and Mg#-Ni and -Cr. Mid-Ocean-Ridge
Basalts (MORB;
PetDB)
and Hawaiian OIB (Gaffney et al., 2004;
Mukhopadhyay et al., 2003; Norman and Garcia, 1999) are
also shown for comparison.
Peridotitic mantle
metasomatized by small-degree melts of rutile-bearing
eclogite would be characterized by high Nb/Ta ratios,
low Nb and Ta concentrations, and low HREE abundances.
In contrast, mechanical mixing of the complementary
rutile-bearing eclogitic residues with deeper mantle
peridotite would develop mantle garnet- and clinopyroxene-rich
reservoirs with high Nb and Ta contents but low Nb/Ta
ratios. In both cases, mixing or reaction with the peridotite would result in
dilution of TiO2 contents such that rutile would no longer be a primary phase
during partial melting of resulting hybrid sources. Subsequent re-melting of
the metasomatized peridotite could have given rise to the high Nb/Ta and low
Nb and Ta contents of the > 110 Ma basalts while re-melting of the peridotite-eclogite
residue mixtures could have given rise to the low Nb/Ta and high Nb and Ta contents
of the < 110 Ma basalts.
High Fe/Mn ratios caused by garnet-pyroxenite sources
A statistical review of previous experiments indicates
that, for less than 50% melting, high Fe/Mn ratios
(> 60)
in “primitive” basalts point to an eclogite/pyroxenite
source, while lower values suggest a peridotite source
(Figure 3a). Unlike Hawaiian basalts, most of the high
Fe/Mn Chinese basalts do not have high Ni, Cr and SiO2 contents (Figures 2c, d), and thus were not derived
from ol-free pyroxenitic sources formed by high-Si
melt-peridotite reaction (Sobolev et al.,
2005). This can be resolved if the pyroxenitic sources
were formed by mechanical mixing of rutile-bearing
eclogite and peridotite. Such mantle sources can explain
well the high Fe/Mn ratios, negative Fe/Mn-Yb (Y) correlations
of the < 110 Ma
basalts (Figure 3b). The good positive correlation between
Sc and Yb for the < 110 Ma basalts (except for those
from Caiyuan) is consistent with garnet-rich sources
(Figure 3d).
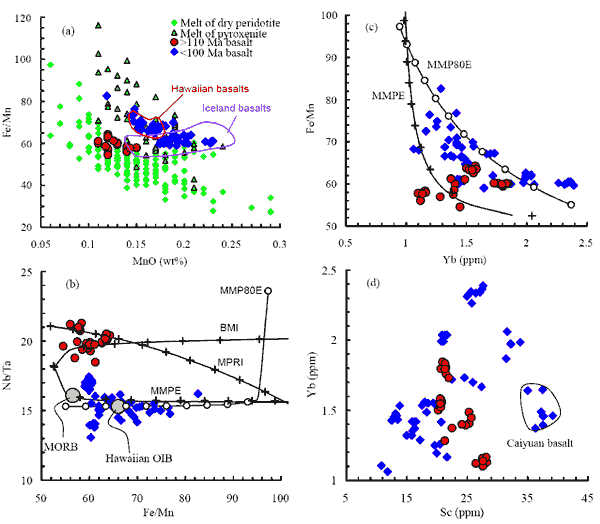
Figure 3 (a) Plots of Fe/Mn ratios
versus MnO contents of basaltic melts formed by partial
melting of pyroxenites (Keshav et al., 2004; Kogiso & Hirschmann,
2001; Kogiso et al., 2004; Pertermann & Hirschmann,
2003a) and dry peridotites (Baker & Stolper,
1994; Falloon & Danyushevsky, 2000; Hirose & Kushiro,
1993; Laporte et al., 2004; Pickering-Witter & Johnston,
2000; Schwab & Johnston, 2001; Walter, 1998; Wasylenki
et al., 2003). Products of melt-peridotite reaction
and melts of high Fe/Mn peridotitic material were not
compiled in (a). Hawaiian basalts (Huang et al., 2007;
Humayun et al., 2004) and Iceland basalts (Qin & Humayun,
2008; Thomas Find et al., 2006) were shown for comparison.
(b) Plots of Nb/Ta ratio vs. Fe/Mn ratio. (c, d) Plots
of Yb vs. Sc and Fe/Mn ratio. MPRI, BMI and MMPE in
(b, c) are partial melts of mixtures of peridotite
+ secondary pyroxenite formed by peridotite-melt reaction
by infiltration (MPRI) and injection (BMI), and mechanical
mixture of peridotite + eclogite (MMPE). Melting degrees
are 1%, 10% and 10% for MPRI, BMI and MMPE, respectively.
Each plus on the lines represents a 10% increment of
pyroxenite. MMP80E are partial melts of 20% peridotite
+ 80% eclogite. Each circle on the line represents
an increment of 10% partial melting. Fe/Mn ratio from
Humayun et al. (2004) and Nb/Ta ratio from Pfänder
et al. (2007) for Hawaiian OIB.
Fe/Mn ratios of the > 110
Ma basalts are still higher than MORB values (Figure
3b) and values predicted for melting of dry peridotite (Figure 3a). These observations
also suggest pyroxene/garnet-rich mantle sources. Although REE patterns and low
HREE contents of these > 110 Ma basalts demonstrate garnet signature (Figure
1), the negative correlation between Sc and Yb suggests that their mantle sources
are pyroxene-dominated (Figure 3d). Their positive correlations between Fe/Mn
ratio and HREE are inconsistent with abundant garnet in the source either (Figure
3c). These observations suggest that mantle sources for the > 110 Ma basalts
could be gar-bearing opx-rich pyroxenite or veined peridotite composed of lherzolite
and gar-bearing opx-rich pyroxenite.
Towards a unified model for the Mesozoic and Cenozoic
basalts
Those composite xenoliths found in the Cenozoic basalts
(Liu et al.,
2005) and Mesozoic high-Mg diorite
(Chen & Zhou, 2005) in the NCC could have
sampled the Mesozoic pyroxenite-veined peridotite formed
by melt-peridotite reactions. Although opx-rich pyroxenite
formed by the melt-rock reaction would not be significantly
enriched in highly incompatible elements, low degree
melting (~ 1%) of pyroxenite + peridotite mixture could
produce high Nb/Ta ratios and trace element patterns
of > 110 Ma basalts (Figures 3d and 4a). As an alternative,
we modeled melting of mixtures of “crystallized
eclogite melt” and peridotite. Injection of silicic
melts into cold peridotite, in the form of a dike or
grain boundary melt as well as melt-peridotite reaction,
can be approximated as simple melt-solid mixing in a
closed system. Because the eclogite-derived melt is enriched
in highly incompatible elements compared to the peridotite
wallrock, addition of a few percent melt can substantially
change the incompatible element and Sr-Nd isotopic compositions
of the mixture. Partial melts of such mixtures at a relatively
high melting degree (10%) show not only the island arc-type
trace element compositions (Figure 4b) and high Nb/Ta
ratios (Figure 3b), but also Sr-Nd isotopic compositions
of the > 110 Ma basalts (Figure 4d).
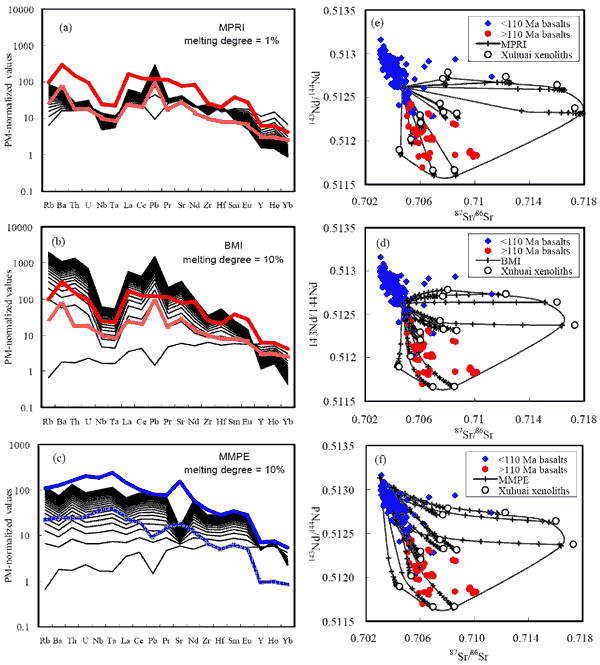
Figure 4 (a) Partial melts of mixture
formed by melt-peridotite reaction by infiltration
(MPRI). Silicic melt was assumed to be produced by
10% partial melting of recycled lower continental
crust. (b) Partial melts of mixture formed by peridotite-melt
reaction by injection (BMI). (c) Partial melts of
mechanical mixtures of peridotite and residual eclogite
(MMPE). Peridotite was assumed to be composed of
65% ol + 15% cpx + 15% opx + 5% sp, and converted
to pyroxenite (40% cpx + 30% opx + 30% gar) after
addition of eclogite melt. Partial melts were simply
calculated according to batch melting mode. Each
thin line represents a 10% increment of secondary
pyroxenite for MPRI and BMI, and eclogite for MMPE.
The thick lines are maximum and minimum values of the > 110
Ma basalts in (a, b) and < 110 Ma basalts in (c).
(c-f) Sr-Nd isotopic compositions of partial melts
of MPRI, BMI and MMPE. Model calculations were conducted
using the Xuhuai eclogite/garnet clinopyroxenite xenoliths
(Wang et al., 2005) for the composition of recycled
lower continental crust. Nd and Sr isotopic compositions
of the > 110 Ma mantle peridotites were represented
by harzburgite xenoliths with the most radiogenic Nd
from the 133 Ma Laiwu pyroxene-diorite (143Nd/144Nd=0.51263;
Xu et al., 2003) and the average 87Sr/86Sr
of continental lithospheric mantle (Pearson & Nowell,
2002). Nd and Sr isotopic compositions of the < 110
Ma mantle peridotites were represented by lherzolite
xenoliths from the 22.8 Ma Hannuoba basalt (143Nd/144Nd =
0.51318, 87Sr/86Sr = 0.70316;
Song & Frey, 1989). Sr and Nd isotopic compositions
of the basalts were collected from literatures. Each
plus on the lines represents a 10% increment of secondary
pyroxenite for MPRI and BMI, and eclogite for MMPE.
Cpx phenocrysts with reverse
zoning (i.e., high FeO, MnO, Na2O, and Al2O3 contents
in the core) in the Cenozoic basalts suggest presence of crustal pyroxenite in
their mantle sources (Wang et al., 2007). Melting of mechanical mixtures
of rutile-bearing eclogitic residues with mantle peridotite to the point of rutile
exhaustion would yield melts with features of the < 110 Ma basalts (e.g.,
low Nb/Ta ratios, high Fe/Mn ratios and OIB-type trace element patterns; Figures
3b and 4c). Our calculations suggest that 30-100% eclogite/garnet clinopyroxenite
is required to produce the OIB-type trace element compositions of the < 110
Ma basalts for 10% partial melting (Figure 4c). Such a high eclogite/garnet clinopyroxenite
proportion could be attributed to lower solidi for pyroxenite compared to peridotite.
At a given pressure, eclogite-garnet clinopyroxenite will be preferentially sampled
during partial melting, particularly at low melt fraction. Therefore, the net
proportion of pyroxenite in the melted part of the mixture could be significantly
higher than that in the whole mixture. The highly variable Sr-Nd isotopic compositions
of the < 110 Ma basalts can be produced by partial melting of such a mixture
using the Xuhuai eclogite/garnet clinopyroxenite xenoliths as the recycled continental
crust (Figure 4f).
To summarize, we suggest that the
differentiated Nb/Ta and high Fe/Mn ratios of the basalts
are fingerprints of the Mesozoic delamination and foundering
of deep lithosphere. The sources of the > 110 Ma
basalts were garnet-bearing opx-rich pyroxenite or
pyroxenite-veined peridotite formed by reaction and/or
mixing between peridotite and eclogite-derived felsic
melts, while the sources of the < 110 Ma basalts
were mechanical mixtures of peridotite and eclogitic
residues formed by the earlier stage of felsic melt
extraction.
Acknowledgments
We thank G.G. Gao, B.H. Zhang, C.L. Tong and J.Q.
Wang for their ICP-MS and XRF analysis work.
References
-
Baker M. B. and Stolper E. M. (1994) Determining
the composition of high-pressure mantle melts using
diamond aggregates. Geochim. Cosmochim. Acta 58,
2811-2827.
-
Chen L. H. and Zhou X. H. (2005) Subduction-related
metasomatism in the thinning lithosphere: Evidence
from a composite duniteorthopyroxenite xenolith entrained
in Mesozoic Laiwu high-Mg diorite, North China Craton. Geochem.
Geophys. Geosyst. 6(Q06008),
doi:10.1029/2005GC000938.
-
Falloon T. J. and Danyushevsky
L. V. (2000) Melting of refractory mantle at 1.5,
2 and 2.5 GPa under anhydrous and H2O-undersaturated
conditions: Implications for the petrogenesis of
high-Ca boninites and the influence of subduction
components on mantle melting. J.
Petrol. 41, 257-283.
-
Foley
S. F., Barth M. G., and Jenner G. A. (2000) Rutile/melt
partition coefficients for trace elements and an
assessment of the influence of rutile on the trace
element characteristics of subduction zone magmas. Geochim.
Cosmochim. Acta 64, 933-938.
-
Gaffney
A. M., Nelson B. K., and Blichert-Toft J. (2004)
Geochemical constraints on the role of oceanic
lithosphere in intra-volcano heterogeneity at West
Maui, Hawaii. J.
Petrol. 45, 1663–1687.
-
Gao
S., Rudnick R. L., Carlson R. W., McDonough W.
F., and Liu Y. S. (2002) Re-Os evidence for replacement
of ancient mantle lithosphere beneath the North
China craton. Earth Planet. Sci. Lett. 198,
307-322.
-
Gao S., Rudnick R. L., Yuan H. L., Liu X.
M., Liu Y. S., Xu W. L., Ling W. L., Ayers J.,
Wang X. C., and Wang Q. H. (2004) Recycling lower
continental crust in the North China craton. Nature 432,
892-897.
-
Green T. H. and Pearson N. J. (1987) An experimental
study of Nb and Ta partitioning between Ti-rich minerals
and silicate liquids at high pressure and temperature. Geochim.
Cosmochim. Acta 51, 55-62.
-
Griffin
W. L., Zhang A., O'Reilly S. Y., and Ryan C. G.
(1998) Phanerozoic evolution of the lithosphere
beneath the Sino- Korean Craton. In Mantle Dynamics
and Plate Interactions in East Asia, Vol.
Geodynamics Volume, 27 (ed. M. Flower, S.L. Chung,
C.H. Lo, and T.Y. Lee), pp. 107-126. American Geophysical
Union.
-
Hirose K. and Kushiro I. (1993) Partial melting
of dry peridotites at high pressures: Determination
of compositions of melts segregated from peridotite
using aggregates of diamond. Earth Planet. Sci.
Lett. 114,
477-489.
-
Hirschmann M. M. and Stolper
E. (1996) A possible role for garnet pyroxenite
in the origin of the "garnet
signature" in MORB. Contrib. Mineral. Petrol. 124,
185-208.
-
Huang S., Humayun M., and Frey F. A. (2007)
Iron/manganese ratio and manganese content in shield
lavas from Ko'olau Volcano, Hawai'i. Geochim.
Cosmochim. Acta 71,
4557-4569.
-
Humayun M., Qiu L., and Norman
M. D. (2004) Geochemical evidence for excess iron
in the mantle beneath Hawaii. Science 306,
91-94.
-
Kay R. W. and Kay S. M. (1991) Creation and
destruction of lower continental crust. Geol.
Rundsch. 80,
259-278.
-
Kelemen P. B., Hanghoj K., and Greene A.
R. (2003) One view of the geochemistry of subduction-related
magmatic arcs, with emphasis on primitive andesite
and lower crust. In The Crust, Vol. 3 (ed.
R.L. Rudnick), pp. 593-659. Elsevier.
-
Keshav S., Gudfinnsson
G. H., Sen G., and Fei Y.-w. (2004) High-pressure
melting experiments on garnet clinopyroxenite and
the alkalic to tholeiitic transition in ocean-island
basalts. Earth Planet. Sci. Lett. 223,
365-379.
-
Klemme S., Prowatke S., Hametner
K., and Günther
D. (2005) Partitioning of trace elements between
rutile and silicate melts: Implications for subduction
zones. Geochim.
Cosmochim. Acta 69, 2361–2371.
-
Kogiso
T. and Hirschmann M. M. (2001) Experimental study
of clinopyroxenite partial melting and the origin
of ultra-calcic melt inclusions. Contrib. Mineral.
Petrol. 142, 347-360.
-
Kogiso
T., Hirschmann M. M., and Pertermann M. (2004)
High-pressure partial melting of mafic lithologies
in the mantle. J. Petrol. 45,
2407–2422.
-
Laporte D., Toplis M. J., Seyler
M., and Devidal J. M. (2004) A new experimental technique
for extracting liquids from peridotite at very low
degrees of melting: application to partial melting
of depleted peridotite. Contrib.
Mineral. Petrol. 146, 463-484.
-
Lee
C. T. A., Cheng X., and Horodyskyj U. (2006) The
development and refinement of continental arcs
by primary basaltic magmatism, garnet pyroxenite
accumulation, basaltic recharge and delamination:
insights from the Sierra Nevada, California. Contrib. Mineral. Petrol. 151,
222-242.
-
-
Lustrino M. (2005) How the delamination and
detachment of lower crust can influence basaltic
magmatism. Earth
Sci. Rev. 72, 21-38.
-
McDonough
W. F. and Sun S. S. (1995) The composition of the
earth. Chem. Geol. 120,
223-253.
-
Menzies M. A., Fan W. M., and
Zhang M. (1993) Paleozoic and Cenozoic lithoprobes
and the loss of > 120
km of Archean lithosphere, Sino-Korean craton, China.
In Magmatic Processes and Plate Tectonics,
Vol. 76 (ed. H. M. Prichard, T. Alabaster, N. B.
W. Harris, and C. R. Neary), pp. 71-81. Geol Soc
Spec Pub.
-
Mukhopadhyay S., Lassiter J. C., Farley
K. A., and S.W. Bogue. (2003) Geochemistry of Kauai
shield-stage lavas: Implications for the chemical
evolution of the Hawaiian plume. Geochem. Geophys.
Geosyst. 4,
1009, doi:10.1029/2002GC000342.
-
Norman M. D. and Garcia
M. O. (1999) Primitive magmas and source characteristics
of the Hawaiian plume: petrology and geochemistry
of shield picrites. Earth Planet.
Sci. Lett. 168, 27-44.
-
Pearson
D. G. and Nowell G. M. (2002) The continental lithospheric
mantle: characteristics and significance as a mantle
reservoir Phil. Trans. R. Soc. Lond.
A 360, 2383-2410.
-
Pertermann
M. and Hirschmann M. M. (2003a) Anhydrous partial
melting experiment on MORB-like eclogites phase
relations, phase composition and mineral-melt partitioning
of major elements at 2-3 GPa. J. Petrol. 44,
2173-2202.
-
Pertermann M. and Hirschmann M. M. (2003b)
Partial melting experiments on a MORB-like pyroxenite
between 2 and 3 GPa: constraints on the presence
of pyroxenites in basalt source regions. J. Geophys.
Res. 108,
2125, 10.1029/2000JB000118.
-
Pfänder J. A., Münker
C., Stracke A., and Mezger K. (2007) Nb/Ta and
Zr/Hf in ocean island basalts -- Implications for
crust-mantle differentiation and the fate of Niobium. Earth
Planet. Sci. Lett. 254,
158-172.
-
Pickering-Witter J. and Johnston A. D. (2000)
The effects of variable bulk composition on the melting
systematics of fertile peridotitic assemblage. Contrib.
Mineral. Petrol. 140, 190-211.
-
Qin
L. and Humayun M. (2008) The Fe/Mn ratio in MORB
and OIB determined by ICP-MS. Geochim. Cosmochim.
Acta 72, 1660-1677.
-
Rapp R.
P., Shimizu N., Norman M. D., and Applegate G.
S. (1999) Reaction between slab-derived melts and
peridotite in the mantle wedge: experimental constraints
at 3.8 GPa. Chem. Geol. 160,
335-356.
-
Rudnick R. L. (1995) Making continental crust. Nature 378,
571-578.
-
Schmidt M. W., Dardon A., Chazot G., and
Vannucci R. (2004) The dependence of Nb and Ta rutile-melt
partitioning on melt composition and Nb/Ta fractionation
during subduction processes. Earth Planet. Sci.
Lett. 226,
415-432.
-
Schwab B. E. and Johnston A. D. (2001) Melting
Systematics of Modally Variable, Compositionally
Intermediate Peridotites and the Effects of Mineral
Fertility. J. Petrol. 42,
1789-1811.
-
Sobolev A. V., Hofmann A. W., Kuzmin D.
V., Yaxley G. M., Arndt N. T., Chung S.-L., Danyushevsky
L. V., Elliott T., Frey F. A., Garcia M. O., Gurenko
A. A., Kamenetsky V. S., Kerr A. C., Krivolutskaya
N. A., Matvienkov V. V., Nikogosian I. K., Rocholl
A., Sigurdsson I. A., Sushchevskaya N. M., and Teklay
M. (2007) The amount of recycled crust in sources
of mantle-derived melts. Science 316,
412-417.
-
Sobolev A. V., Hofmann A. W., Sobolev S.
V., and Nikogosian I. K. (2005) An olivine-free mantle
source of Hawaiian shield basalts. Nature 434,
590-597.
-
Song Y. and Frey F. A. (1989) Geochemistry
of peridotite xenoliths in basalt from Hannuoba,
Eastern China: Implications for subcontinental mantle
heterogeneity. Geochim.
Cosmochim. Acta 53, 97-113.
-
Stalder
R., Foley S. F., Brey G. P., and Horn I. (1998)
Mineral-aqueous fluid partitioning of trace elements
at 900-1200°C and 3.0-5.7 GPa: New experimental
data for garnet, clinopyroxene, and rutile, and
implications for mantle metasomatism. Geochim.
Cosmochim. Acta 62,
1781-1801.
-
Taylor S. R. and McLennan S. M. (1985) The
continental crust: Its composition and evolution.
Blackwell Scientific.
-
Thomas Find K., Kaj H., Folkmar
H., Jens F., Reinhard W., and Dieter G.-S. (2006)
Combined Trace Element and Pb-Nd-Sr-O Isotope Evidence
for Recycled Oceanic Crust (Upper and Lower) in the
Iceland Mantle Plume. J.
Petrol. 47, 1705-1749.
-
Walter
M. J. (1998) Melting of garnet peridotite and the
origin of komatiite and depleted lithosphere. J.
Petrol. 39, 29-60.
-
Wang Q.
H., Xu W. L., Wang D. Y., Lin J. Q., and Gao S.
(2005) Geochemical characteristics of eclogite
xenoliths in Mesozoic intrusive complex from Xu-Huai
area and its tectonic significance. Earth Sci.
J. China University Geosci. (in Chinese with English
abs.) 30,
414-420.
-
Wang W., Xu W., Wang D., Ji W., Yang D.,
and Pei F. (2007) Caiyuanzi Paleogene basalts and
deep-derived xenocrysts in eastern Liaoning, China:
constraints on nature and deep process of the Cenozoic
lithospheric mantle. J. Mineral. Petrol. 27,
63-70.
-
Wasylenki L., Baker M., Kent A., and Stolper
E. (2003) Near-solidus melting of the shallow upper
mantle: partial melting experiments on depleted peridotite. J.
Petrol. 44, 1163-1191.
-
Wu
F. Y., Walker R. J., Ren X. W., Sun D. Y., and
Zhou X. H. (2003) Osmium isotopic constraints on
the age of lithospheric mantle beneath northeastern
China. Chem.
Geol. 196, 107-129.
-
Xiong
X. L., Adam T. J., and Green T. H. (2005) Rutile
stability and rutile/melt HFSE partitioning during
partial melting of hydrous basalt: Implications
for TTG genesis. Chem. Geol. 218,
339-359.
-
Xu J. F., Shinjo R., Defant M. J., Wang Q.,
and Rapp R. P. (2002) Origin of Mesozoic adakitic
intrusive rocks in the Ningzhen area of east China:
Partial melting of delaminated lower continental
crust? Geology 30,
1111-1114.
-
Xu W. L., Wang D. Y., Wang Q. H., and Lin
J. Q. (2003) Petrology and geochemistry of two types
of mantle-derived xenoliths in Mesozoic diorite from
western Shandong province. Acta Petrol. Sin. 19,
623-636.
-
Xu Y. G. (2001) Thermo-tectonic destruction
of the Archaean lithospheric keel beneath the Sino-Korean
craton in China: Evidence, timing and mechanism. Phys.
Chem. Earth (A) 26, 747-757.
-
Yaxley
G. M. (2000) Experimental study of the phase and
melting relations of homogeneous basalt + peridotite
mixtures and implications for the petrogenesis of
flood basalts. Contrib. Mineral. Petrol. 139,
326-338.
-
Yaxley G. M. and Green D. H. (1998) Reactions
between eclogite and peridotite: mantle refertilisation
by subduction of oceanic crust. Schweiz. Mineral.
Petrogr. Mitt. 78, 243-255.
-
Yuan
X., Sobolev S. V., Kind R., Oncken O., Bock G.,
Asch G., Schurr B., Graeber F., Rudloff A., Hanka
W., Wylegalla K., Tibi R., Haberland C., Rietbrock
A., Giese P., Wigger P., Rower P., Zandt G., Beck
S., Wallace T., Pardo M., and Comte D. (2000) Subduction
and collision processes in the Central Andes constrained
by converted seismic phases. Nature 408,
958-961.
last updated 9th
May, 2008 |