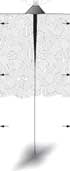 |
Shear-focusing
of pre-existing melt as an origin for small-volume
intraplate volcanism |
Greg A. Valentine
Department
of Geology, University at Buffalo, USA, gav4@buffalo.edu
This webpage is a summary of the following
papers:
Valentine
G.A., Hirano N., (2010), Mechanisms of low-flux intraplate
volcanic fields – Basin
and Range (North America) and Northwest Pacific Ocean, Geology, 38,
55-58, doi:10.1130/G30427.1
Valentine G.A., Perry F.V., (2007),
Tectonically controlled, time-predictable basaltic
volcanism from a lithospheric mantle source (central
Basin and Range Province, USA), Earth
and Planetary Science Letters, 261,
201-216, doi:10.1016/j.epsl.2007.06.029
Valentine G.A., Perry F.V., (2006),
Decreasing magmatic footprints of individual volcanoes
in a waning basaltic field, Geophysical Research
Letters, 33,
L14305, doi:10.1029/2006GL026743
Intraplate volcanic fields occur in
both continental and seafloor settings, and examples
can be found in all types of regional tectonic environments.
While deep-seated mantle plumes, upwelling asthenosphere,
and other traditional mechanisms make
sense for some of these intraplate volcanic systems,
there are many cases where these explanations really
do not work. This is especially true for volcanic fields
with low magma output rates (eruptive flux). Such systems
are characterized by small volcanoes (~0.005-2 km3)
that are commonly monogenetic, meaning that they have
only a single eruptive phase, which might last days
to perhaps a few decades, and then never erupt again,
excepting the low probability event where a completely
new volcano happens to intersect a previous one.
The volcanoes in these systems tend to be alkali basalts
that reflect small melt fractions in their mantle sources.
Volcanoes in low-flux fields typically do not follow
simple spatial-temporal trends, for example where ages
decrease in a certain direction. Rather, a new volcano
might erupt anywhere in the volcanic field, seemingly
independently of the location of the preceding event.
Some of these volcanic fields have very low eruptive
fluxes; in the Southwest Nevada Volcanic Field (SNVF,
western USA), the eruptive flux is currently about
0.5 km3/Ma (Valentine & Perry,
2007).
To put this in perspective, Kilauea volcano has a magma
flux approximately six orders of magnitude greater.
Although there are few well-documented low-flux volcanic
fields, many of them do have one thing in common–the
presence of some sort of regional tectonic deformation. Valentine & Perry (2007) suggested that small monogenetic volcanoes in the SNVF
are not the result of an active melting process in
the upper mantle, but instead represent focusing of
patches of preexisting partial melt. It is noteworthy
that in the SNVF, geochemical and isotopic data suggest
the magma sources reside in the lithospheric mantle,
which reaches depths of ~100 km locally. These sources
represent compositional heterogeneities such as local
enrichments in hydrous phases, which in turn depress
the solidus temperature such that at the ambient pressure
and temperature (~60-80 km depth) they have small melt
fractions. Regional deformation focuses melt within
these sources until the melt interconnectivity is sufficient
to develop a buoyant pressure head that can initiate
upward dike propagation. This deformation-driven melt
focusing is the mechanism explored by Holtzman & Kohlstedt (2007)
in the context of mid-ocean-ridge spreading centers.
In the conceptual model proposed by Valentine & Perry (2007),
there is a close relationship between volcano characteristics
on the surface, and their magma sources at depth. For
example, in the SNVF the eruptive fissure lengths are
related to the volumes of individual volcanoes, as
are lava effusion rates that are inferred from field
data. Each volcano is sourced by a separate patch of
partial melt, with its own geochemical signature, in
the upper mantle. The volume of melt available to ascend
and erupt is proportional to the volume of the partial
melt patch. The strike-length of dikes, and ultimately
eruptive fissures (the intersection of dikes with Earth’s
surface), that ascend from a partial melt patch is
related to the length scale or “magmatic footprint” of
the patch (Valentine & Perry,
2006).
Larger-volume patches produce longer dikes, which
are reflected on the surface as longer fissures and
larger eruptive volumes. Effusion rate is proportional
to dike width cubed, which is proportional to dike
length. Thus, longer dikes erupt larger volumes at
faster rates during individual monogenetic events.
Each volcano can be considered as a sample of a heterogeneity
in the upper mantle (Figure 1A). At the scale of the
volcanic field, the eruptive volume flux exhibits time-predictable
behavior, i.e., the repose interval is proportional
to the volume of the preceding eruption. This is consistent
with a close relationship between regional deformation
and volcanism.
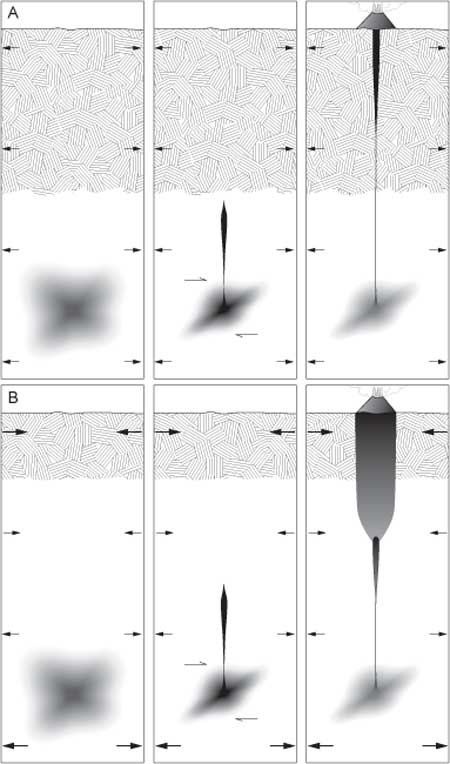
Figure 1: Cartoons showing cross
sections through the crust (line pattern) and upper
mantle (white) under regional deformation. Shaded area
in the mantle represents a hypothetical patch containing
partial melt. During regional deformation, the relatively
weak, partially molten patch takes up relatively more
deformation than the surroundings, and melt is focused
until it is sufficiently interconnected to trigger
ascending dikes. (A) In the Southwest Nevada Volcanic
Field, the lithosphere, including the magma sources
in the lithospheric mantle, is subject to horizontal
s3 with an orientation
that varies little with depth, facilitating direct
dike ascent to the surface. (B) In the NPO, flexure
causes extension perpendicular to the flexure axis
in the lower lithosphere, and perhaps partly in the
upper asthenosphere, which is not locally clearly defined,
where magmas are sourced, but ascending dikes stall
at mid-lithosphere levels because s3 has
a different orientation in the upper lithosphere. Stalled
magmas fractionate and eventually trigger new dikes
with the proper orientation to travel through the remaining
lithosphere and erupt on the sea floor.
Valentine & Perry (2007) use the SNVF as an example of the very-low-eruptive-flux
end-member of volcanic field behavior, where magmatism
can only occur in the presence of tectonic deformation
(tectonically controlled). They compare this with
the high flux end-member where the thermal structure
of the upper mantle and high rate of dike injection
into the crust is proposed to overwhelm regional tectonics
(magmatically controlled volcanism, e.g., the
mantle plume hypothesis). The spectrum of behavior
may control other factors such as the likelihood of
ascending dikes being “captured” by
pre-existing structures vs. making their own
pathways. Understanding this has the potential to contribute
to hazard assessment.
Valentine & Hirano (2010) extended this concept to explain recently discovered,
young fields of small-volume alkali basalt volcanoes
on the floor of the Northwest Pacific Ocean (NPO),
a setting that has long been thought to be volcanically
quiescent, as it contains the oldest ocean lithosphere
on the planet. Those small volcanoes form as the Pacific
lithosphere is flexed upward prior to downwarping where
it is subducted beneath Japan. Geochemical data suggest
that melts feeding NPO volcanism are related to a heterogeneous
upper mantle with locally depressed solidus temperatures. Valentine & Hirano (2010) compare SNVF and NPO volcanism for a broad suite of
characteristics and propose that in the latter case
the regional deformation associated with flexure is
responsible for focusing preexisting melts sufficiently
to trigger upward dike injection. In contrast to the
SNVF, where dikes essentially ascend uninterrupted
through the crust, in the NPO ascending dikes stall
at mid-lithosphere depths in response to rotation of
the principal stresses. There, at depth, s3 is
perpendicular to the flexure axis, while in the upper
lithosphere
s1 is perpendicular
to the axis (Figure 1). Stalling results in fractionation,
assimilation and incorporation of xenolithic material,
which partly overprints the source geochemistry.
This conceptual model for NPO volcanism
is proposed as an alternative to the “petit spot” interpretation
by Hirano et al. (2006), which assumes that
magmas rise passively through flexure-induced fractures
that penetrate the entire lithosphere. That model overlooks
the effect of vertical rotation of principal stresses
in flexure, as well as the difficulty of generating
open fractures extending to depths of ~80-90 km. Also,
perhaps unintentionally, the term “petit spot” seems
to imply upwelling (i.e., small hot spots
or microplumes) as well as implying that NPO volcanism
is a unique phenomenon, when in reality it is very
similar to volcanic fields on continents and likely
on the sea floor.
Shear focusing for mobilizing and collecting small
melt fractions, together with mantle that varies on
a scale of kilometers in composition and solidus temperature,
is a reasonable alternative to mechanisms that invoke
active melting, for low-eruptive-flux volcanic fields.
References
-
Hirano, N., Takahashi, E., Yamamoto,
J., Abe, N., Ingle, S.P., Kaneoka, I., Hirata,
T., Kimura, J.-I., Ishii, T., Ogawa, Y., Machida,
S., and Suyehiro, K., 2006, Volcanism in response
to plate flexure, Science, 313, 1426-1428 and online
supplemental material.
-
Holtzman, B.K., and Kohlstedt,
D.L., 2007, Stress-driven melt segregation and
strain partitioning in partially molten rocks:
effects of stress and strain, Journal
of Petrology,
48, 2379-2406.
-
-
-
last updated 1st
October, 2011 |