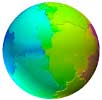 |
Breaking Earth's shell into a global plate network |
C.A. Tang1,2, A.A.G. Webb3, W.B. Moore4,5, Y.Y. Wang6, T.H. Ma1,6, T.T. Chen7
1State Key Laboratory of Coastal & Offshore Engineering, Dalian University of Technology, Dalian 116024, China; tca@mail.neu.edu.cn ; aagwebb@hku.hk ; tianhuima@dlut.edu.cn
2State Key Laboratory of Geological Processes and Mineral Resources, China University of Geosciences (Wuhan) 430074, China
3Division of Earth and Planetary Science and Laboratory for Space Research, University of Hong Kong, Pokfulam Road, Hong Kong 999077, China.
4Department of Atmospheric and Planetary Sciences, Hampton University, Hampton, Virginia 23668, USA; william.moore@hamptonu.edu
5National Institute of Aerospace, Hampton, Virginia 23666, USA.
6Deep Underground Engineering Research Center, Dalian University of Technology, Dalian 116024, China; yongyiwang017@qq.com
7School of Resources and Civil Engineering, Northeastern University, Shenyang 110819, China; chentiantian0304@163.com
This webpage is a summary of our open-access paper: Tang, C.A., Webb, A.A.G., Moore, W.B. et al. Breaking Earth’s shell into a global plate network. Nature Communications, 11, 3621 (2020).
Background
We propose a new concept for how plate tectonics initiated on Earth. A cold, early lithosphere may have experienced thermal expansion through the tensile failure limit, thereby rapidly developing a global rift network and setting the stage for subduction tectonics. As this idea bears a striking resemblance to long-discredited concepts such as the expanding Earth hypothesis, a deeper discussion is warranted, as follows.
First, it is noteworthy that, although the continental drift concept is over 100 years old and widespread acceptance of plate tectonics is over 50 years old, we yet lack a consensus understanding of how and why plate tectonics developed (e.g., Hansen, 2007; Moore & Webb, 2013; Bercovici & Ricard, 2014; Rey et al., 2014; Gerya et al., 2015). Nonetheless, there does appear to be a growing consensus that plate tectonics was preceded by some form of single-plate lithosphere, most likely a “hot” stagnant lid (using the model terminology of Lenardic, 2018). If so, then the initiation of plate tectonics involved overcoming the strength of this lid. Proposed physical mechanisms for this include: (a) mantle convective forcing, which may be focused along lithospheric weak zones that progressively developed over 100s of millions of years prior to failure (e.g., Moore & Webb, 2013; Bercovici & Ricard, 2014); (b) gravitational instabilities formed via juxtaposition of differently weighted lithosphere (e.g., Rey et al., 2014; Gerya et al., 2015); and (c) an exogenic impactor trigger (e.g., Hansen, 2007). Geodynamic modeling of these concepts has focused on continuum mechanics approximations of the processes responsible for rock failure. Thus far, these approximations have not been successfully connected to rock failure properties derived from laboratory experiments but rather have relied on matching the characteristics of Earth’s plate scale dynamics.
A new approach
Our new work offers two signal departures from other current work–a strikingly different idea for how the lid ruptures (thermal expansion), and the application of solid mechanics modeling to illustrate this idea. This depends directly on standard rock mechanics physical properties. We introduce both these new approaches below.
For thermal expansion to be sufficient to overcome the lithosphere’s tensile strength, the pre-expansion lithosphere must be cold, with a large temperature difference between the mantle and the surface. One model for generation of the early lithosphere permits this: heat-pipe cooling (Moore & Webb, 2013). In this cooling mode, heat-loss through the lithosphere is dominated by volcanic advection. Volcanic advection brings hot material from the mantle to the surface, whereupon the heat is lost to space and the previously hot magma is deposited on the surface as cold material. Continual volume transfer from depth to the surface requires subsidence to keep pace with the volcanism, so the cold surface materials sink rapidly, cooling the lithosphere. Because Earth as a whole is cooling, this early heat production, volcanic activity, and lithospheric cooling would have eventually slowed, leading to increased warming of the lithosphere as conductive heat-loss became more dominant. This conductive warming is postulated to be the cause of thermal expansion in our new model.
We illustrate this process of thermal expansion, lithospheric rupture, and the rapid growth of a fault network dividing Earth's lithosphere into plates by a series of numerical simulations of spherical shells generated using the 3D finite element program RFPA (Rock Failure Process Analysis) developed by Tang (1997) (Figure 1). In our models, up to 3.45 million elements are used in the three-dimensional spherical shell model and subjected to quasi-static, slowly increasing internal pressure in a displacement controlled manner (i.e. due to gradual thermal expansion). Brittle failure is implemented by a strength criterion, which represents the stress limit when strength decreases and fracture occurs. In order to consider local randomness, each component is assigned a failure threshold, which is obtained by Weibull probability distribution, which contains a homogeneity index and a parameter describing the uniformity of materials. According to the criterion of rock strength and Poisson's ratio, a range of rock strength is obtained.
Results
Nine experiments were carried out on a model sphere with a radius of 6000 km. Models 1 to 7 used different values of shell thickness (20, 40, 60, 80, 100, 120, and 200 km) to explore the effect of lid thickness on fracture modes.
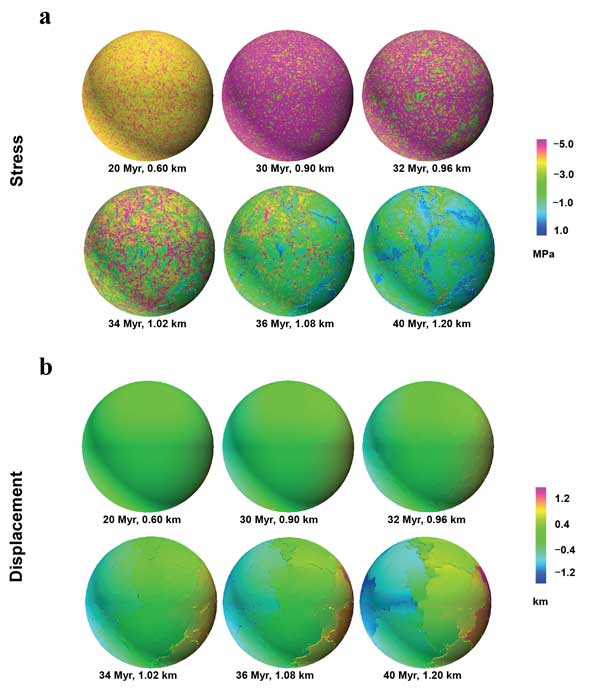
Figure 1. Plate-breaking reference model (from Tang et al., 2020). The reference model shows progressive development of a global fracture network in response to displacement-controlled increasing interior pressure (at 0.03 km/Myr) applied to an 80 km thick lithosphere. A. Global surface distribution of minimum principal stress magnitudes through time (negative values denote tensile stress, positive values compressive stress). B. Associated displacement (articulated along a horizontal axis, from left to right through each sphere’s center). In both A. and B. surface elements which attain their ultimate tensile strain are displayed as fractures in black. At 20 Myr, the single-plate lithosphere remains macroscopically undamaged. At 30-32 Myr, with sustained interior pressure, cracks initiate and propagate. By 34 Myr, crack coalescence generates features such as triple junctions. By 40 Myr, with the intersection of most major crack systems, a global fracture network is established, dividing the surface of the sphere into plate-like segments.
Similar patterns were observed in all models, despite variations in fracture growth rates and fracture spacing (polygonal areas) (Figure. 2). Comparison of the simulation results shows that crack spacing increases with shell thickness under the condition that other parameters remain unchanged. For greater shell thickness, the loading times/quantity required for rapid global-scale crack network development is greater. Fractures propagate in a zigzag manner and bend and bifurcate at the critical fracture length, controlled by the strength of the shell. As the cracks grow and intersect, they equate to plate boundaries connected at triple junctions (Figure. 1).
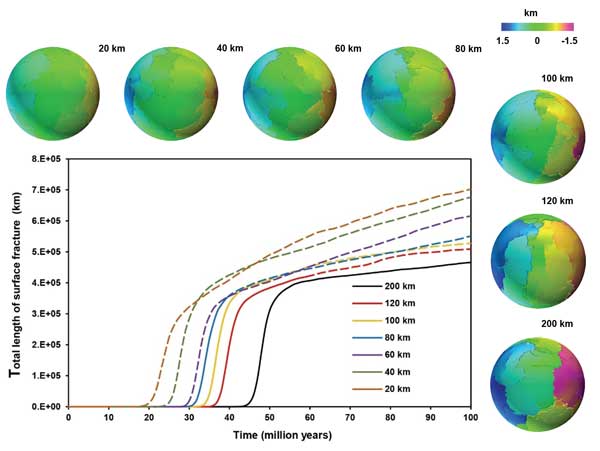
Figure 2. Fracture development across shells with various thicknesses (from Tang et al., 2020). Surface fracture development for seven models with different shell thickness (other variables held constant), imaged in terms of uplift with total fracture lengths. Dashed lines indicate evolution beyond the thermal expansion limit. The spheres show displacement (articulated as in Figure 1) as each model achieves a total length of surface fractures of 4.0E+05 km.
Our modeling demonstrates a self-organized fracture mechanism analogous to thermal-expansion-driven lithospheric uplift where globe-spanning rifting occurs as a consequence of horizontal extension. Resulting fracture spacing is a function of lithospheric thickness and rheology (Tang et al., 2006) where geometrically regular polygonal tessellation is an energy efficient solution because it minimizes total crack length. Polygonal fissure patterns could develop on Earth’s surface in response to shallow lithospheric processes. Such a fracture mechanism allows for lithospheric rifting on a global scale with triple-junctions as by-products of fracturing.
Summary
Our modeling illustrates how early warming of the lithosphere, as expected in the heat-pipe model, should lead to the necessary conditions for fracture, fault propagation and initiation of multi-plate tectonics. We note that our model does not explicitly produce subduction boundaries. Nonetheless, because the global rift network would likely focus volcanism by providing easy eruptive pathways, we would anticipate differential loading of the lithosphere. Prior studies suggest that such weighting could have initiated subduction (e.g., Rey et al., 2014; Gerya et al., 2015).
References
- Bercovici, D. & Ricard, Y. Plate tectonics, damage and inheritance. Nature 508, 513-516 (2014).
- Gerya, T. V., Stern, R. J., Baes, M., Sobolev, S. V. & Whattam, S. A. Plate tectonics on the Earth triggered by plume-induced subduction initiation. Nature 527, 221-225 (2015).
- Hansen, V.L., Subduction origin on early Earth: a hypothesis. Geology 35, 1059-1062 (2007).
Lenardic, A. The diversity of tectonic modes and thoughts about transitions between them. Phil. Trans. R. Soc. A 376: 20170416 (2018).
- Rey, P. F., Coltice, N. & Flament, N. Spreading continents kick-started plate tectonics. Nature 513, 405-408 (2014).
- Moore, W. B. & Webb, A. A. G. Heat-pipe Earth. Nature 501, 501-505, doi:10.1038/nature12473 (2013).
- Tang, C. A. Numerical simulation of progressive rock failure and associated seismicity. Int J Rock Mech Min 34, 249-261 (1997).
- Tang, C. A. et al. Fracture spacing in layered materials and pattern transition from parallel to polygonal fractures. Phys Rev E 73 (2006).
last updated 10th
August, 2020 |