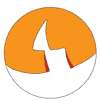 |
Rifted continental margins: Geometric control on crustal architecture and melting |
Erik R. Lundin1, T.F. Redfield2, & G. Peron-Pindivic2
1Statoil ASA, Arkitekt Ebbels v 10, 7005 Trondheim, NORWAY, erlun@statoil.com
2Geological Survey of Norway, Leiv Erikssons v 39, 7040 Trondheim, NORWAY, Tim.Redfield@NGU.NO ; Gwenn.Peron-Pinvidic@NGU.NO
In a recent conference paper (Lundin et al., 2014) we suggest that, to a first order, rifted-margin architecture and degree of melting is related to the linear rate of extension. Our hypothesis leads directly to a new model to describe the distribution of magma-poor and magma-rich rifted margins.
Our model (Figure 1) applies the simple rules of rigid plate tectonics. These require that the linear extension rate at a given location along an opening rift or ocean is controlled by the distance from the pole of rotation and the angular rate of extension (Figure 2). To a first order, lithospheric plates may be treated as rigid and their angular rate will always be constant within the times governed by a given stage pole. The linear extension rate will always be slowest close to the rotation pole and fastest most distally from the pole. Thus our hypothesis predicts that magma-poor rifted margins form proximally to the pole of rotation while magma-rich margins form distally to it.
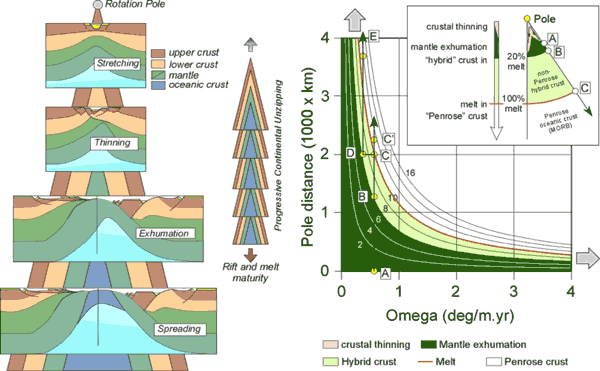
Figure 1: Left: The V-shaped nature of rift facies maturity, and how paired, diachronous patterns form under stepwise pole propagation. Right: XY plot showing the relationship between pole location, pole angular velocity, and local linear velocity (shown as isolines). The critical half-rates "hybrid in" and "melt in" are represented by 6 mm/yr and 10 mm/yr respectively after the model of Perez-Gussinyé et al. (2006). Inset shows model from the plate-tectonic Euler perspective. Path A-B-C illustrates a typical facies progression at any one instant in time. If rotation conditions change, variations are possible. Path C-D-E illustrates a case of regression resulting from a decrease in angular velocity. Such paths might occur in nature when two large rift-tip segments overlap and spreading is temporarily divided between them. Click here or on image for enlargement.
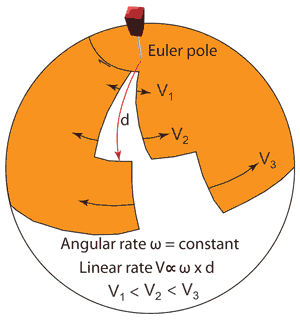
Figure 2: Schematic illustration of relationship between local linear rate of extension (V), distance from rotation pole (d), and angular rate of extension (ω).
It is well-established that magma-poor margins form during slow extension. Although perhaps not so well-emphasized, it appears clear that magma-rich margins form under more rapid extension. The term “margin” applies to continental breakup, when mantle is exhumed along the magma-poor end-members and seaward-dipping reflectors are extruded along the magma-rich end-members. Notably, exhumed mantle may contain up to ca 12% melt trapped in a fine network (Müntener et al., 2010), and are, as the term implies, not devoid of melt. Both rifted-margin types can be viewed as end-members of the same process of continental extension, firmly rooted in the geometric requirements of plate tectonics. Our model does not rule out the existence of mantle heterogeneities, nor their possible influence on melt generation, but rather suggests that such heterogeneities are secondary to the rifted-margin architecture process.
We observe the proximal-magma-poor to distal-magma-rich relationship (Figure 3) along the margins of the South Atlantic, Central Atlantic, NE Atlantic-Eurasia Basin, Red Sea, and the Arctic Canada Basin. An important aspect of the rupture of large continents is that it occurs by propagation of a rift tip. Rift propagation can be demonstrated in a number of areas such as the South, Central, North, and NE Atlantic-Eurasia Basin, the South China Sea, the Red Sea, and the Gulf of Aden. It can also be identified by magnetic isochrons on the sea floor, where such are present, and by geological features such as progressively younger rift basins (e.g., South Atlantic), age progression of rift-shoulder uplift and associated “break-up” unconformity (e.g., Bay of Biscay), and age-progression of pre-breakup magmatism along an evolving rift (e.g., Labrador Sea). Due to the limitations of resolution to the regional scale, propagation can seem to be either continuous (e.g., Iberia-Newfoundland) or via sequential, stepwise-opening segments some hundreds or thousands of kilometers long (e.g., South Atlantic and Labrador Sea).
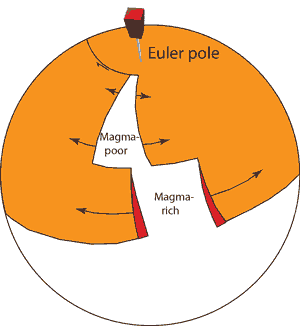
Figure 3. Schematic illustration of distribution of magma-poor and magma-rich rifted margins, proximal and distal to the rotation pole respectively. The distal position is characterized by high extension rates and vice versa (Figure 2). This proximal-distal relationship is observed in the South Atlantic, Central Atlantic, NE Atlantic-Eurasia Basin, Red Sea, and Canada Basin.
On a grander scale, magma-poor rifted margins are bordered by belts of exhumed mantle that may be thousands of kilometers in length. Oceanward of these belts, oceanic crust can be seen to young in the direction of the rotation pole. This suggests that as large continents unzip by rift propagation, the mantle is exhumed in the wake of the advancing rift tip. Laterally, these margins evolve from:
- limited crustal stretching, to
- extreme crustal thinning,
- exhumed mantle, and
- ultraslow or normal oceanic crust.
It is difficult to document the lateral increase in strain rate that our model predicts, but it may be possible off the coast of Iberia.
Our hypothesis suggests that magma-rich margins tend to form when a rift tip propagates rapidly to a more distant position and the rate of extension rapidly increases. The increase in strain rate favors higher heat-flow, sharp necking, and sub-aerial breakup. The magma itself can potentially be generated by decompression. Although current numerical models have difficulty generating sufficient melt, field observations from the fossil Tethyan margin indicate that larger quantities of basaltic type melt can be extracted from mantle rocks than has been previously thought. The Bay of Biscay system illustrates a long-distance pole jump that resulted in sea-floor exhumation of mantle changing directly to sea-floor spreading. Similarly, a drastic change in spreading rate may have been responsible for the voluminous magmatism of the NE Atlantic breakup.
References
-
Lundin, E.R., T.F. Redfield, and G. Peron-Pindivic, 2014, Rifted continental margins: geometric influence on crustal architecture and melting, in J. Pindell, B. Horn, N. Rosen, P. Weimer, M. Dinkleman, A. Lowrie, R. Fillon, J. Granath, and L. Kennian, eds, Sedimentary basins: Origin, depositional histories, and petroleum systems: 33rd Annual GCSSEPM Foundation Bob F. Perkins Conference, p. 18-53. If you are interested in this original paper, please email the corresponding author at erlun@statoil.com
-
Müntener, O., G. Manatschal, L. Desmurs, and T. Pettke, 2010. Plagioclase peridotites in ocean-continent transitions: Refertilized mantle domains generated by melt stagnation in the shallow mantle lithosphere, Journal of Petrology, 51, 255-294, doi: 10.1093/petrology/egp087.
-
Pérez-Gussinyé, M. Phipps Morgan, J., Reston, T.J. & Ranero, C.R. ,2006. The rift to drift transition at non-volcanic margins: Insights from numerical modeling, Earth and Planetary Science Letters, 44, 458-473.
last updated 2nd April, 2014 |