 |
|
Radiating
Volcanic Migrations:
An example from the Pacific Northwest, U.S.A.
|
|
|
|
This
webpage is a abstracted from a more thorough discussion
of the CRB magmatic system in Camp
& Ross
(2004)
Click here to
download a PDF version of this webpage
|
Introduction
A great variety of criteria have
been used in assessing the validity of plume and non-plume
models (e.g., Foulger et al., 2002;
Anderson, 2003; Courtillot et al.,
2003; Ernst & Buchan, 2003), not the
least of which is the recognition of temporal and
spatial trends in the eruption sequence of large igneous
provinces. The geometry and presumed outward propagation
of giant radiating dike swarms, for example, appears
to be more compatible with a plume origin than with
non-plume alternatives, such as EDGE convection or
lithospheric rifting along linear trends (see Giant
Radiating Dike Swarm page, and EDGE
convection page). Lateral flow away from the focal
point of such swarms appears to be supported by limited
AMS (anisotropy of magnetic susceptibility) studies
(e.g., Ernst and Baragar, 1992). However, questions
on the magnetic fabric of individual dikes have introduced
doubt on the reliability of AMS measurements as predictors
of long-distance horizontal flow (see Giant
Dike Patterns page). This doubt places greater
emphasis on the application of field and stratigraphic
data to the assessment of migratory trends in large
igneous provinces.
|
Migrating Magmatic
Trends in the Pacific Northwest
Recent mapping, together with petrochemical
and paleomagnetic correlations (Ferns et al.,
1993a, b; Lees, 1994; Binger, 1997; Johnson
et al., 1998; Cummings et al., 2000; Hooper
et al., 2002; Glen and Ponce, 2003; Camp
et al., 2003) demonstrate a series of temporal and
spatial trends in the Miocene flood-basalt succession
of the Pacific Northwest. These appear to delineate
the outward propagation of magmatism from a centralized
source, analogous to similar trends denoted by the AMS
studies on giant radiating dike swarms.
Two types of age-progressive trends
have been recognized in the Columbia Plateau-Oregon
Plateau-Snake River Plain-Northern Nevada Rift magmatic
system (Camp and Ross, 2004):
- those with rapid migration rates, significantly
greater than rates of convection and plate motion
(~10-100 cm/yr), and
- those with moderate migration rates, similar to
estimated rates of mantle convection and plate motion
(~1-5 cm/yr).
The rapid-rate migrations are associated
with the main phase of flood-basalt volcanism, propagating
outward from a centralized source in southeastern Oregon.
The moderate-rate migrations are defined by later eruptions
emanating outward from the same central site.
Rapid-Rate Migrations Contemporaneous with Flood-Basalt
Volcanism
The main eruptive phase of flood-basalt
volcanism occurred over a period of six paleomagnetic
intervals (R0-N2), from ~16.6 to 15.0 Ma (Figure 1). The initial eruptions
began in southeastern Oregon, generating the Steens
Basalt succession. These lavas are well exposed at Steens
Mountain (Johnson et al., 1998), where more than
900 m of basalt erupted over the R0-N0 paleomagnetic transition (Mankinen et al., 1987).
Mapping of the paleomagnetic and petrochemical stratigraphy,
in concert with Ar-Ar geochronology, demonstrate that
flood basalt volcanism migrated rapidly outward along
three primary trends (Figure 2):
- the Chief Joseph trend, marked by the northward
advance of dikes and progressively younger lavas of
the Imnaha and Grande Ronde Basalts during N0-N2 (e.g., Camp, 1995),
- the Steens-Picture Gorge trend, marked by the discontinuous
northward advance of progressively younger eruptions
of Steens (R0-N0), Picture Gorge (N1-R2), and Prineville (R2-N2) Basalts, from Steens Mtn. to the northern end of the Monument
dike swarm, and
- the Northern Nevada Rift trend, marked by the southward
advance of dikes and a diminished volume of Steens
Basalt (R0-N0) and younger intermediate to felsic volcanic rocks (e.g.,
Glen & Ponce, 2002).
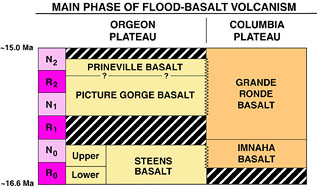
Figure 1. Magnetostratigraphy
of the flood basalt units on the Columbia and
Oregon Plateaus during the main phase of eruption
(~16.5-15.0 Ma).
Figure 2 (right). Distribution
map for the Columbia River Flood Basalt Pronvince.
MC, McDermitt caldera; OIG, Oregon-Idaho graben;
WSRP, western Snake River Plain. Click on figure
for enlargment. |
|
Moderate-Rate Migrations after the Flood-Basalt
Event
Flood-basalt volcanism was followed by the progressive
eruption of rhyolite along two well-recognized trends
(Figure 3):
- to the northeast, along the Snake River Plain hotspot
track, and
- to the west-northwest, along the Oregon High Lava
Plains (see High Lava
Plains page).
Whereas the Snake River Plain hotpsot
track was generated above Precambrian continental lithosphere
lying east of the cratonic margin (the 0.706 Sr isopleth
in Figure 3), the Oregon High Lava Plains trend was
generated above oceanic lithosphere west of the cratonic
margin.
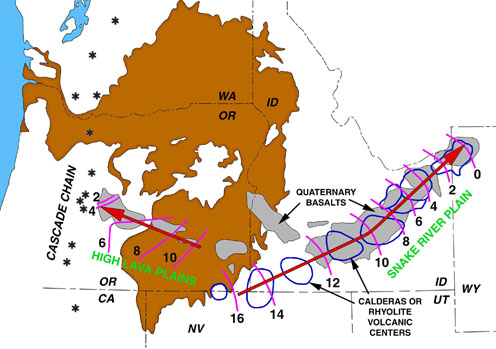
Figure 3. Moderate-rate migrations
of rhyolte magmatism associated with the Oregon High
Lava Plains and the Snake River Plain hotspot track.
Distribution of Quaternary basalt and isochrons for
rhyolitic volcanism from Jordan et al. (2002) and Christiansen
et al. (2002).
The post-15 Ma felsic eruptions west
of the cratonic margin were contemporaneous with sporadic
eruptions of low-volume, but widely scattered, high-alumina
olivine tholeiite (HAOT) (Hart et al., 1984).
Figure 4 illustrates the map distribution of the youngest
of these HAOTs, with ages < 8 Ma. Although these
young HAOTs are widespread and exhibit a lack of orderly
age-progression across eastern Oregon, the initial eruptions
of HAOT appear to have progressed westward away from
the cratonic boundary, as denoted by the dashed contours
in Figure 4. The oldest post-15.0 Ma HAOTs yet recognized
are the ~13.9-to-13.1 Ma Tims Peak basalts of east-central
Oregon. The oldest dates farther to the west and south
indicate that inception of HAOT volcanism was between
11.0 and 8.0 Ma. Still farther to the west, the initial
HAOT eruptions are no older than 8.0 Ma. In this westernmost
zone, there is a systematic westward younging of HAOT
outcrops across northeastern California and adjacent
Oregon, with ages divisible into two belts: 8-4 Ma in
the east, and < 4 Ma against the Cascade volcanic
arc to the west.
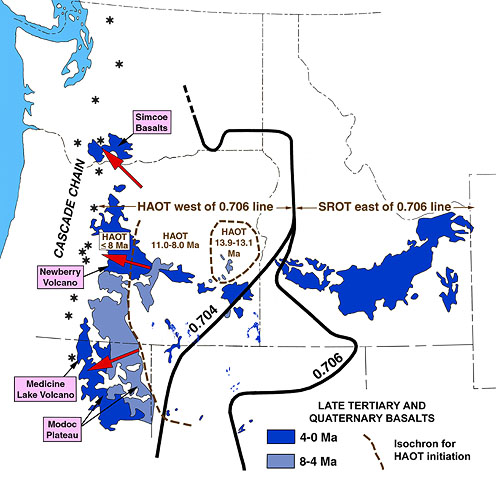
Figure 4. Distribution of Late Tertiary
to Quaternary basalts, derived from Hart et al. (1984),
Jordan et al. (2002), and Christiansen (2002). The 0.704
and 0.706 lines correspond with the initial 87Sr/86Sr
isopleths of Kistler and Peterman (1978) and Leeman
et al. (1992). HAOT and SROT are restricted to areas
lying west and east of the 0.706 line, respectively.
Isocrhons (dashed lines) showing a west to east migration
of initial HAOT eruptions are based the field mapping,
chemical correlations and chronological data of Hart
et al. (1984), Ferns et al. (1993a), Christiansen et
al. (2002), Hooper et al. (2002), and Camp et al. (2003). |
Assessing Tectonomagmatic
Models for the Pacific Northwest
A great variety of non-plume models
have been proposed for the Columbia Plateau, the Oregon
Plateau, the Northern Nevada Rift, and the Snake River
Plain provinces. Some of these include back-arc extension
for the Oregon Plateau (Carlson & Hart, 1988),
EDGE convection for the Oregon and Columbia Plateaus
(King & Anderson, 1998), Basin and Range
rifting for the Northern Nevada Rift (Zoback &
Thompson, 1978), an eastward propagating rift for
the Snake River Plain (Hamilton, 1987), divergent
upper mantle flow around a residuum body for the Snake
River Plain and High Lava Plains (Humphreys et al.,
2000), and a self-sustaining melting anomaly generated
by thermal feedback between lithospheric rifting and
shear melting along the Snake River Plain hotspot track
(Christiansen et al., 2002). However, to develop
separate models for each province is at odds with the
geologic evidence that they are contemporaneous and
genetically related, each province forming an inherent
part of a single magmatic system. Although many of these
models are adequate in explaining the geologic features
of any one province, they are generally inadequate in
explaining the geologic evolution of coeval adjacent
provinces composing the magmatic system as a whole.
Of the non-plume alternatives for flood-basalt
volcanism, the boundary edge model of King &
Anderson (1998) provides an elegant explanation
for the location of the Chief Joseph dike swarm against
the Precambrian boundary of North America. However,
this model is incompatible with rifting and volcanism
across the cratonic boundary along the Northern Nevada
Rift. Neither the EDGE model nor the other non-plume
alternatives offers a reasonable mechanism to explain
the rapid outward migration of volcanism along the Chief
Joseph, Steens-Picture Gorge, and Northern Nevada Rift
trends. The outward progression of volcanism away from
a focal point in southeastern Oregon is more compatible
with a plume model, as originally suggested by Draper
(1991), and consistent with AMS studies on the propagation
of dikes in giant radiating dike swarms.
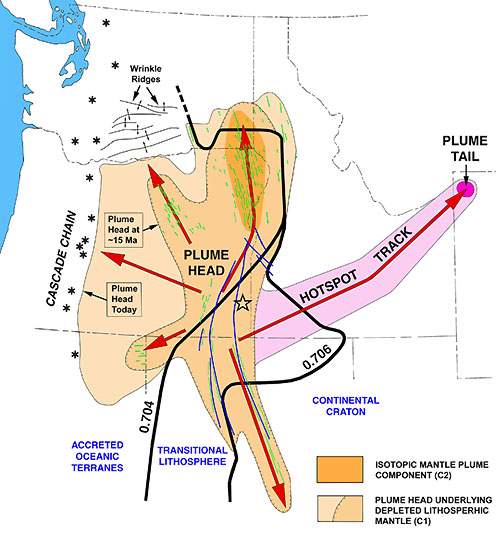
Figure 5. Two-stage spreading model
of the proposed Yellowstone mantle plume head, showing
(1) the approximate position of the proposed plume head
after impingement and rapid spreading (~15.0 Ma), and
(2) the approximate position of the proposed plume head
today after moderate-rate spreading associated with
asthenospheric drag and counterflow above the subducting
plate. The short lines located above the area of first-stage
spreading are surficial dikes, and the longer curvilinear
lines are the linear magnetic anomalies of Glen and
Ponce (2002), which are thought to be buried intrusions
or keel dikes. The open star near the Oregon-Idaho border
corresponds with the focus of the dike swarms after
correcting for block rotation (Ernst & Buchan, 2001).
The approximate boundaries of distinct lithospheric
domains beneath the plume head are delineated by the
87Sr/86Sr isopleths of Kistler
& Peterman (1978), and Leeman et al. (1992).
A two-stage spreading model for the
Yellowstone mantle plume is shown in Figure 5. The model
suggests that impingement of a mantle plume head in
the Pacific Northwest resulted in rapid spreading in
all directions, but preferentially to the north beneath
thin lithosphere of the accreted oceanic terranes. Spreading
to the south, beneath thicker transitional to continental
lithosphere produced shallow intrusions and a much smaller
volume of lava along the southward propagating Northern
Nevada Rift system (Glen & Ponce, 2003).
Shearing off of the plume head against the westward-advancing,
thick cratonic margin at ~15 Ma allowed the plume tail
to generate a hotspot track through the overidding craton.
Once decapitated from its feeding plume tail, the plume
head continued to advance westward, at a much slower
rate, by asthenospheric drag and by counterflow above
the descending Juan de Fuca plate (see High
Lava Plains page), thus generating a westward migration
of HAOTs and rhyolitic melts, at a rate consistent with
mantle convection. |
Summary
Although we are open to alternative
views, we believe that the existing non-plume models
are inadequate in explaining the overall geology of
mafic magmatism in the Pacific Northwest. A plume genesis,
however, appears to provide:
- a unifying model for the entire system, which is
lacking in nonplume interpretations,
- a rational mechanism to account for rapid, radiating
volcanic migrations, also lacking in non-plume interpretations,
and
- a more reasonable explanation for the sudden outburst
and short duration (~1.5 million years) of the main
phase of flood-basalt volcanism.
|
References
-
Anderson, D.L., What is a plume?,
in The Hotspot Handbook, Proceedings of
the Penrose Conference Plume IV, Hveragerdi, Iceland,
1-3, 2003.
-
Binger, G.B., The volcanic
stratigraphy of the Juntura region, eastern Oregon,
M.Sc. thesis, 206 pp., Washington State University,
Pullman, 1997.
-
Camp, V.E., Mid-Miocene propagation
of the Yellowstone mantle plume head beneath the
Columbia River Basalt source region, Geology,
23, 435-438, 1995.
-
Camp, V.E., M.E. Ross and W.
E. Hanson, Genesis of flood basalts and Basin and
Range volcanic rocks from Steens Mountain to the
Malheur River Gorge, Oregon, Geol. Soc. Am.
Bull., 115, 105-128, 2003.
-
- Camp V.E.,
and Ross, M.E., 2004, Mantle dynamics and genesis
of mafic magmatism in the intermontane Pacific Northwest,
Jour. Geophys. Res., vol. 109, B08204, doi:1029/2003JB002838
-
Carlson, R.W. and W.K. Hart,
Flood basalt volcanism in the Pacific Northwestern
United States, in Continental Flood Basalts,
edited by J.D. Macdougal, pp. 35-62, Kluwer Academic
Publishers, Dordrecht, The Netherlands, 1988.
-
Christiansen, R.L., G.R. Foulger
and J.R. Evans, Upper-mantle origin of the Yellowstone
hotspot, Geol. Soc. Am. Bull., 114,
1245-1256, 2002.
- Courtillot, V., A. Davaile, J. Besse and J. Stock,
Three distinct types of hotspots in the Earth's mantle,
Earth Planet. Sci. Lett., 205,
295-308, 2003.
-
Cummings, M..L., J.G. Evans,
M.L. Ferns and K.R. Lees, Stratigraphic and structural
evolution of the middle synvolcanic Oregon-Idaho
graben, Geol. Soc. Am. Bull., 112,
668-682, 2000.
-
Draper, D.S., Late Cenozoic bimodal
magmatism in the northern Basin and Range Province
of southeastern Oregon, J. Volc. Geotherm. Res.,
47, 299-328, 1991.
-
Ernst, R.E. and W.R.A. Baragar,
Evidence from magnetic fabric for the flow pattern
of magma in the Mackenzie giant radiating dyke swarm,
Nature, 356, 511-513,
1992.
- Ernst, R.E. and Buchan, K.L., The use of mafic dike
swarms in identifying and locating mantle plumes,
pp. 247-265 In: Ernst, R.E. and Buchan, K.L. (eds.)
Mantle Plumes: Their Identification Through Time.
Geol. Soc. Am. Special Paper 352, 2001.
-
Ernst, R.E. and K.L. Buchan,
Recognizing mantle plumes in the geological record,
Ann. Rev. Earth Planet. Sci., 29,
469-523, 2003.
-
Ferns, M.L., H.C. Brooks, J.G.
Evans and M.L. Cummings, Geologic map of the
Vale 30x60 minute quadrangle, Malheur County, Oregon,
and Owyhee County, Idaho, Dept. Geol. &
Min. Ind. Geol. Map Ser. GMS-77, Scale 1:100000,
1993a.
-
Ferns, M.L., J.G. Evans and M.L.
Cummings, Geologic map of the Mahogany Mountain
30x60 minute quadrangle, Malheur County, Oregon,
and Owyhee County, Idaho, Dept. Geol. &
Min. Ind. Geol. Map Ser. GMS-77, Scale 1:100000,
1993b.
- Foulger, G.R., Plumes, or plate tectonic processes?,
Astron. Geophys., 43, 6.19-6.23,
2002.
-
Glen, J.M.G. and D.A. Ponce,
Large-scale fractures related to inception of the
Yellowstone hotspot, Geology, 30,
647-650, 2003.
-
Hamilton, W.B., Plate-tectonic
evolution of the Western U.S.A., Episodes,
10, 271-276, 1987.
-
Hart, W.K., J.L. Aronson and
S..A. Mertzman, Areal distribution and age of low-K
high alumina olivine tholeiite magmatism in the
northwestern Great Basin, Geol. Soc. Am. Bull.,
95, 185-195, 1984.
-
Hooper, P.R., G.B. Binger and
K.R. Lees, Constraints on the relative and absolute
ages of the Steens and Columbia River basalts and
their relationship to extension-related clac-alkaline
volcanism in eastern Oregon, Geol. Soc. Am.
Bull., 114, 43-50, 2002.
-
Humphreys, E.D., K.G. Dueker,
D.L. Schutt and R.B. Smith, Beneath Yellowstone:
Evaluating plume and nonplume models using teleseismic
images of the upper mantle, GSA Today,
10, 1-6, 2000.
-
Johnson, J.A., C.J. Hawkesworth,
P.R. Hooper and G.B., Binger, Major and trace element
analyses of Steens Basalt, southeastern Oregon,
U.S. Geol. Sur. Open-File Rep. 98-482,
26 p., 1998.
-
Jordan, B.T., M.J. Streck and
A.L. Grunder, Bimodal volcanism and tectonism of
the High Lava Plains, Oregon, in Field guide
to geologic processes in Cascadia, edited by
G.W. Moore, Oregon Depart. of Min. Indus. Special
Pub. 36, pp. 23-46, 2002.
-
King, S.D. and D.L. Anderson,
Edge-driven convection, Earth and Planet. Sci.
Lett., 160, 289-296, 1998.
-
Kistler, R.W. and Z.E. Peterman,
Reconstruction of crustal California on the basis
of intitial strontium isotopic compositions of Mesozoic
granitic rocks: U.S. Geol. Surv. Prof. Pap.
1071, 17 pp., 1978.
-
Leeman, W.P. J.S. Oldow and W.K.
Hart, Lithosphere-scale thrusting in the western
U.S. Cordillera as constrained by Sr and Nd isotopic
transitions in Neogene volcanic rocks, Geology,
20, 63-66, 1992.
-
Lees, K.R., Magmatic and
tectonic changes through time in the Neogene volcanic
rocks of the Vale area, Oregon, northwestern USA,
Ph.D. dissertation, 284 pp., Open University, Milton
Keynes, 1994.
-
Mankinen, E.A., E.E. Larson,
C.S. Gromme, M.Prevot and R.S. Coe, The Steens Mountain
(Oregon) geomagnetic polarity transition, 3, Its
regional significance, J. Geophys. Res.,
92, 8057-8076, 1987.
-
Zoback, M.A. and G.A. Thompson,
Basin and Range rifting in northern Nevada: clues
from a mid-Miocene rift and its subsequent offsets,
Geology, 6, 111-116.
|
last
updated April 11th, 2006 |
|
|