Plate-driven Micro-Hotspots |
|
Xing Yua & Henry Dickb
aKey Laboratory of Submarine Geosciences, Second Institute of Oceanography, Ministry of Natural Resources, Hangzhou, China; yuxing@sio.org.cn ; Chinese home page
bDepartment of Geology and Geophysics, Woods Hole Oceanographic Institution, Woods Hole, MA, USA; hdick@whoi.edu
This webpage is a summary of Yu, X., Dick, H.J.B. 2019. Plate-driven micro-hotspots and the evolution of the Dragon Flag melting anomaly, Southwest Indian Ridge. Earth planet. Sci. Lett. https://doi.org/10.1016/j.epsl.2019.116002
Melting anomaly along the ridges
The magmatism along the axes of mid-ocean ridges is far more heterogeneous than hitherto thought, with excessive magma, thick crust and shallow bathymetry in some segments, while others are extremely magma-poor, have thin crust or even display lower oceanic crust or upper mantle directly exhumed. The variation of magmatism is closely related to partial melting beneath the ridge axis.
The crust along the Southwest Indian Ridge (SWIR) is generally thin and discontinuous, with the mantle spreading directly on the seafloor in many regions (Zhou & Dick, 2013). There are also areas with robust magmatism, such as the Dragon Flag supersegment (SWIR 46-52°E) (Figure 1). Sauter et al. (2009) showed that the flanking rift mountains are, anomalously, 1-2-km higher than adjacent older crust, indicating the sudden onset of excess volcanism at ~8-11 Ma. Seismic and gravity observations indicate that this region has the thickest crust yet found along the entire SWIR, up to 10 km thick for segment 27 (Sauter et al., 2009; Niu et al., 2015 and references therein). This volcanism rapidly migrated east from the Indomed FZ to Gallieni FZ, creating an east-directed, narrow, V-shaped trend.
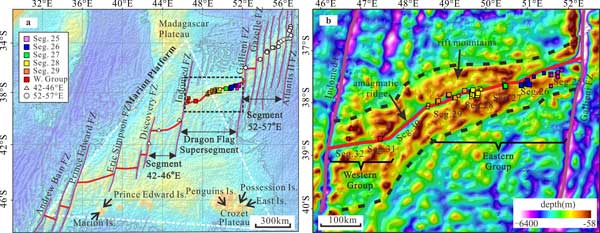
Figure 1. Bathymetric maps for the central SWIR and the Dragon Flag Supersegment with sample locations. Click here or on Figure for enlargement.
Debate on the origin of the melting anomaly
The cause of the melting anomaly could be due to many factors:
- The influence of mantle plumes, including Marion and Crozet (e.g., Georgen et al., 2001);
- mantle source modification by subduction with sediments and oceanic crust (e.g., Rehkämper & Hofmann, 1997; Kempton et al., 2002);
- stranded lower continental crust or continental lithospheric mantle mixed with the upper mantle (e.g., Meyzen et al., 2005; Mahoney et al., 1992; Janney et al., 2005).
Meyzen et al. (2005), based on MORB 87Sr/86Sr, 143Nd/144Nd and 206Pb/204Pb isotopes, suggest that there was no influence from Marion and Crozet mantle plumes on the supersegment. However, Sauter et al. (2009) suggested that the melting anomaly resulted from interaction with Crozet hotspot 800-900 km to the southeast. Dalton et al. (2014) suggest no significant plume influence because of relatively low mantle potential temperature estimated from seismic tomography and MORB major element compositions.
However, Breton et al. (2013), based on Pb-Sr-Nd-He isotopes from the Crozet archipelagos, proposed that the upper mantle of the Supersegment was contaminated by Crozet mantle plume materials. Yang et al. (2017) proposed that during upslope flow from a Crozet mantle plume to the ridge, decompression melting along the path would deplete the plume in incompatible elements. Remelting this residue by further melting beneath the ridge would then produce isotopically enriched N-MORB at Segment 27.
A new model for the origin of the Dragon Flag melting anomaly
Here we present new major-, trace-element, and Sr-Nd-Pb isotopic data for the Dragon Flag Supersegment and review its geological background. We find that the V-shaped bathymetric trend is opposite to that predicted for excess volcanism related to the hotspot reference frame, and the propagation rate (~51 mm/yr) exceeds the spreading rate (14 mm/yr) by a factor of 4. This suggests a plate-controlled source process for the excess volcanism rather than a source heterogeneity fixed in the hotspot reference frame.
Based on plate reconstruction, the Crozet hotspot is currently beneath crust formed at the Southeast Indian Ridge, rather than at the SWIR (Zhang et al., 2011). The Crozet hotspot also lies south of the NE extension of the Del Cano Rise, whose thickened lithosphere would likely impede mantle flow from Crozet to the ridge. Moreover, while seismic crustal thickness has been measured up to 10.2 km at Segment 27 (e.g., Niu et al., 2015), it rapidly thins to the west, and virtually disappears 80 km to the east. Yang et al. (2017) found a Crozet influence only for Segment 27. It is hard to explain, then, why this is not seen at Segments 28 and 29. The small-scale heterogeneity within a segment is difficult to explain by plume-ridge interaction. The Pb isotopes of the supersegment cannot be explained by only integration of the Crozet component, especially in 207Pb/204Pb - 206Pb/204Pb space (Figure 2). Thus, overwhelming geological, geophysical and geochemical evidence suggests Crozet magmatism has no direct relationship to the Dragon Flag Supersegment.
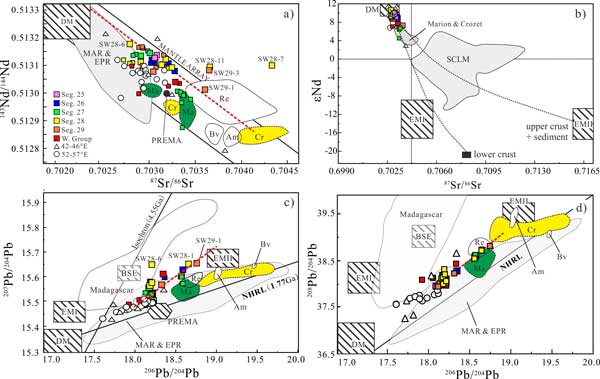
Figure 2. Isotopic variations for MORBs from Dragon Flag Supersegment and adjacent ridge segments. Also included are isotopic data for Crozet (Cr), Marion (Ma), Bouvet (Bv), Amsterdam (Am), Reunion (Re) and Madagascan lavas (Mad). Click here or on Figure for enlargement.
We therefore present a possible mechanism for local melting anomalies that may be widely, perhaps uniquely, applicable to ultraslow spreading ridges [Ed: See also http://www.mantleplumes.org/Disclosure.html, http://www.mantleplumes.org/RR_VSRs.html and http://www.mantleplumes.org/RR_Reorg.html]. Their origin is not directly related to the nearby Marion and Crozet mantle hotspots. Rather they reflect their plate tectonic setting, with deep regions of melt focusing in the mantle controlled by the lithospheric stress field, interacting with an upper mantle source with components of delaminated ancient continental and arc lithosphere entrained in the asthenosphere. These local melting anomalies could be termed “micro-hotspots”.
By reviewing the geochemistry and geology of 5 additional SWIR micro-hotspots, such as the 9° to 16°E oblique spreading center, Joseph Mayes Seamount, the Narrowgate Magmatic Segment and the 61.4° and 63.9°E melting anomalies (Figure 3), we find that micro-hotspots represent excess volcanism at the ultraslow spreading SWIR unrelated to mantle hotspots, but related to focused melt flow in the mantle, wide volcano spacing, and/or increased mantle fertility. Individual micro-hotspots can reflect variations in regional mantle fertility simultaneously affecting 100s of km of ridge, or extreme melt focusing in isolated segments in regions with unusually thick lithosphere.
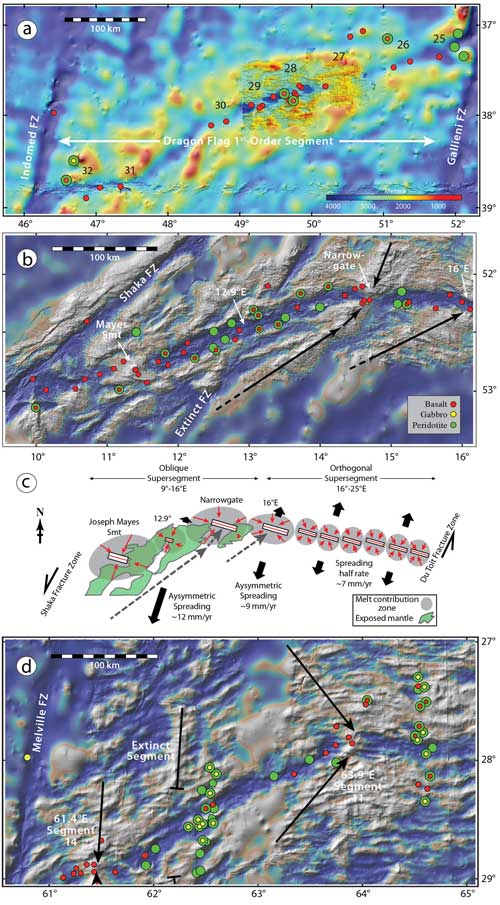
Figure 3. Locations of the melting anomalies of Dragon Flag Supersegment, 9° to 16°E oblique spreading center, Joseph Mayes Seamount, the Narrowgate Magmatic Segment and 61.4° and 63.9°E micro-hotspots. Click here or on Figure for enlargement.
Micro-hotspots are an order of magnitude smaller than Wilson’s classic hotspots, and are not fixed in the hotspot reference frame. They can grow and remain stationary for extended periods, or migrate freely with respect to one another, driven by the evolving plate-tectonic stress field.
References
-
Breton, T., F. Nauret, S. Pichat, B. Moine, M. Moreira, E. F. Rose-Koga, D. Auclair, C. Bosq, and L.-M. Wavrant, 2013. Geochemical heterogeneities within the Crozet hotspot. Earth Planet. Sci. Lett., 376, 126–136, doi:10.1016/j.epsl.2013.06.020.
-
Dalton, C.A., C.H. Langmuir and A. Gale, 2014. Geophysical and Geochemical Evidence for Deep Temperature Variations Beneath Mid-Ocean Ridges. Science 344, 80.
-
Georgen, J. E., J. Lin, and H. J. B. Dick, 2001. Evidence from gravity anomalies for interactions of the Marion and Bouvet hotspots with the Southwest Indian Ridge: effects of transform offsets. Earth Planet. Sci. Lett. 187, 283–300.
-
Janney, P.E., le Roex, A.P., Carlson, R.W., 2005. Hafnium isotope and trace element constraints on the nature of mantle heterogeneity beneath the central Southwest Indian Ridge (13°E to 47°E). J. Petrol. 46, 2427–2464.
-
Kempton, P. D., J. A. Pearce, T. L. Barry, J. G. Fitton, C. Langmuir, and D. M. Christie, 2002. Sr-Nd-Pb-Hf isotope results from ODP Leg 187: Evidence for mantle dynamics of the Australian-Antarctic Discordance and origin of the Indian MORB source. Geochem. Geophys. Geosyst. 3, 1074, doi:10.1029/2002GC000320.
-
Mahoney, J., A. P. le Roex, Z. Peng, R. L. Fisher, and J. H. Natland, 1992. Southwestern limits of Indian Ocean Ridge Mantle and the origin of low 206Pb/204Pb mid-ocean ridge basalt: Isotope systematics of the central Southwest Indian Ridge (17°-50° E). J. Geophys. Res. 97, 19771–19790.
-
Meyzen, C. M., J. N. Ludden, E. Humler, B. Luais, M. J. Toplis, C. Mevel, and M. Storey, 2005. New insights into the origin and distribution of the DUPAL isotope anomaly in the Indian Ocean mantle from MORB of the Southwest Indian Ridge. Geochem. Geophys. Geosyst. 6, Q11K11, doi:10.1029/2005GC000979.
-
Niu, X., A. Ruan, J. Li, T. A. Minshull, D. Sauter, Z. Wu, X. Qiu, M. Zhao, Y. J. Chen, and S. Singh, 2015. Along-axis variation in crustal thickness at the ultraslow spreading Southwest Indian Ridge (50°E) from a wide-angle seismic experiment. Geochem. Geophys. Geosyst. 16, doi:10.1002/2014GC005645.
-
Rehkämper, M., Hofmann, A.W., 1997. Recycled ocean crust and sediment in Indian Ocean MORB. Earth Planet. Sci. Lett. 147, 93–106.
-
Sauter, D., M. Cannat, and C. Meyzen, 2009. Propagation of a melting anomaly along the ultraslow Southwest Indian Ridge between 46°E and 52°20′E: interaction with the Crozet hotspot? Geophys. J. Int. 179, 687–699.
-
Yang, A. Y., Zhao T.-P., Zhou M.-F., and Deng X.-G., 2017. Isotopically enriched N-MORB -– a new geochemical signature of off-axis plume-ridge interaction: A case study at 50°28’E, Southwest Indian Ridge, J. Geophys. Res. 121, doi:10.1002/2016JB013284.
-
Zhang, T., Lin, J., and Gao. J.Y., 2011. Interactions between hotspotsand the Southwest Indian Ridge during the last 90 Ma: Implications on the formation of oceanic plateaus and intra–plate seamounts. Sci. China-Earth Sci. 54, 1177–1188.
-
Zhou, H., and H. J. B. Dick, 2013. Thin crust as evidence for depleted mantle supporting the Marion Rise. Nature 494, 195–200, doi:10.1038/nature11842.
last updated 15th
January, 2020 |