 |
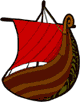 |
A
plume beneath the Oslo Graben? |
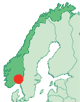 |
|
|
Introduction
In order to discuss
the development of the Oslo Graben in terms of
plate vs. plume models, it is most important to
understand its spatial and temporal relationship
with other areas in NW Europe. Despite its “abnormal”
development, the Oslo Graben is temporally associated
with nearby magmatic events in areas such as southern
Sweden, north-west Germany, Scotland and the central
North Sea (see, for example, Wilson et al.
(2004) for an overview of Carboniferous-Permian
magmatism and rifting in north-western Europe).
The most important of these events is the emplacement
of a suite of alkaline and tholeiitic basalts
at ca. 300-290 Ma (the Carboniferous-Permian boundary)
(Sundvoll et al., 1990; Breitkreuz
& Kennedy, 1999; Heeremans et al.,
2004b; Monaghan & Pringle, 2004;
Timmerman, 2004). Except for this basaltic
spike, all the areas show different tectonomagmatic
developments.
The focus of this
web page will be on the formation of the Oslo
Graben. This graben has long been known as a highly-magmatic
continental rift, with accommodation and transfer
zones separating the different graben segments
(Olaussen et al., 1994). It has been
studied for over a hundred years and a large amount
of geological and geophysical data have been acquired.
Despite this, scientists still argue about its
origin. The present paper will try to shed some
light on the discussion regarding whether a plume
contributed to the origin of the Oslo Graben or
not. Before starting the discussion, I give a
short introduction to the tectonomagmatic development
of the Oslo Graben.
The
tectonomagmatic evolution of the Oslo Graben
The onshore Oslo
Graben forms, together with the offshore Skagerrak
Graben, the Oslo Rift (Ro et al., 1990).
The rift terminates in the Sorgenfrei-Tornquist
Zone (see Figures 1 and 2).
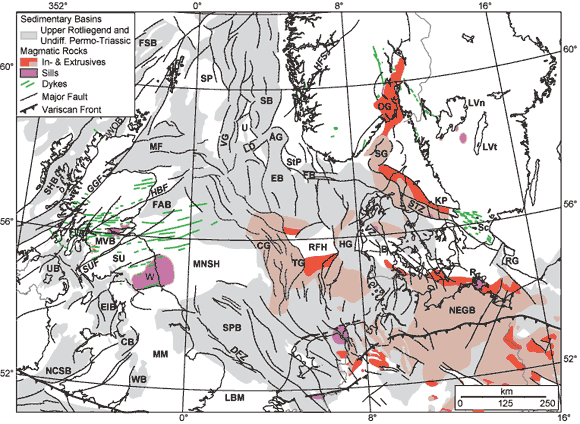
Figure 1: Overview
map for the Late Carboniferous – Early Permian
with structural names. Some basins are of undifferentiated
Permo-Triassic age. Basins: B = Brande Graben;
CB = Cheshire Basin; CG = Central Graben; DB =
Dutch Bank Basin; EB = Egersund Basin; EIB = Eastern
Irish Sea Basin; FAB = Forth Approaches Basin;
FB = Farsund Basin; FSB = Faroes-Shetland Basin;
HG = Horn Graben; LD = Ling Depression; MF = Moray
Firth; MVB = Midland Valley Basin; NCSB = Northern
Celtic Sea Basin; NEGB = North-East German Basin;
OG = Oslo Graben; RG = Rønne Graben; SB
= Stord Basin; SG = Skagerrak Graben; SHB = Sea
of Hebrides Basin; SPB = Sole Pit Basin; STZ =
Sorgenfrei-Tornquist Zone; TG = Tail End Graben;
UB = Ulster Basin; WB = Worcester Basin; WOB =
Western Orkneys Basin; ÅG = Åsta Graben.
Highs: KP = Kattegat Platform; LBM = London-Brabant
Massif; MM = Midland Massif; MNSH = Mid-North
Sea High; RFH = Ringkøbing-Fyn High; Sc
= Scania; SP = Shetland Platform; StP = Stavanger
Platform; U = Utsira High. Regional structures:
DFZ = Dowsing Fault Zone; GGF = Great Glen Fault;
HBF = Highland Boundary Fault; HSZ = Hardangerfjorden
Shear Zone; LGF = Lærdal-Gjende Fault; SUF
= Southern Uplands Fault; VDF = Variscan Deformation
Front; VFZ = Vinding Fracture Zone. Geographical
names: LVn = Lake Vännern; LVt = Lake Vättern;
R = Rügen; SU = Southern Uplands (From (Heeremans
et al., 2004a). Click
on figure for enlargement.
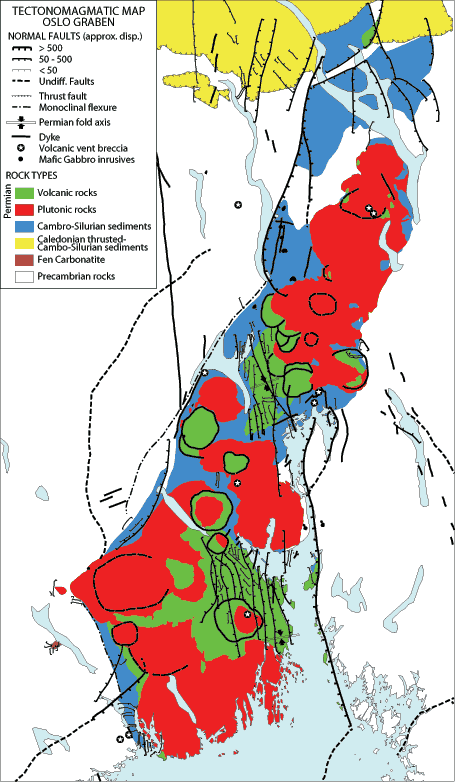
Figure 2: Tectonomagmatic
map of the Oslo Graben (from Ramberg & Larsen,
1978).
The development
of the Oslo Graben has been divided into 5 main
stages (Ramberg & Larsen, 1978; Sundvoll
et al., 1990; Sundvoll & Larsen,
1993; Olaussen et al., 1994). These are:
1.
Stage 1: Pre-rift: Representative
of this stage is the deposition of the Asker Group
sediments (Henningsmoen, 1978; Olaussen,
1981; Olaussen et al., 1994) on top of
a deformed Cambro-Silurian sedimentary succession.
These Asker Group sediments are interpreted as
representing fluvial-dominated deltas, prograding
into lacustrine or brackish water basins. A marine
incursion is found in the upper part of the succession
as indicated by fossils of Westphalian age (Olaussen,
1981). This age is confirmed by U-Pb ages to be
319 ± 5 Ma, derived from detrital zircon
grains from sandstones in the upper Asker Group
(Dahlgren & Corfu, 2001).
Stage
2: Initial rifting: The earliest
magmatic products (Rb-Sr ages 304 ± 8 and
294 ± 7 Ma) related to rifting in the Oslo
Graben are represented by trachyandesitic-rhyolitic
sills and dykes, some of them termed “mænaites”
(Ramberg & Larsen, 1978; Sundvoll
et al., 1992). The sills typically intrude
the base of Cambrian Alum-shales, on top of PreCambrian
basement. The main onset of rifting started with
the emplacement of widespread basaltic volcanism
termed B1. Whereas the southern part of the Oslo
Graben is dominated by alkaline magmatism, the
northern part experienced tholeiitic magmatism.
Thicknesses vary from 800-1500 m in the south
to ca. 15 m in the north (see Neumann et al.,
2004 and references therein). Initial faulting
took place prior to basalt extrusion as evidenced
by faulted Asker Group sediments underlying undisturbed
lavas in the Krokskogen area. Rb-Sr dating of
a tholeiitic lava flow gave an age of 291 ±
7 Ma, but U-Pb ages of a larvikite intrusion,
which is younger than the B1-basalts, are 298.6
± 1.4 Ma, suggesting that basalt volcanism
probably started earlier (Dahlgren et al.,
1996). The B1 basalts are the most primitive basalts
in the Oslo Graben.
Stage
3: The main rifting period: The
main rifting period is characterized by extensive
vertical movements along the main master faults
and extensive fissure eruptions of trachyandesitic
rhomb porphyry lavas. In addition, minor basaltic
lavas were extruded (Ramberg & Larsen,
1978). The rhomb porphyry lavas are inferred to
be associated with the intrusion of large bodies
of monzonite and nepheline syenites (larvikites
and lardalites). U-Pb age determinations of these
larvikite plutons provide ages ranging from 292.1
± 0.8 to 298.6 ± 1.4 Ma (Dahlgren
et al., 1996). Rb-Sr age determinations performed
on the rhomb porphyry lavas and the larvikites
gave ages ranging from 276 ± 6 to 294 ±
6 Ma (Sundvoll et al., 1990). These are
now assumed to underestimate the true ages.
Stage
4: Central volcanoes, caldera collapse and graben
fill: The next
step in graben evolution involved a change from
shield volcanism to the development of central
volcanoes and caldera collapse. Associated with
these phenomena are lavas, dykes, central intrusions
and ring dykes. Erosion of the rift flanks caused
deposition of fan-shaped debris flows.
Stage
5: Batholith emplacement:
The final stage of graben development involved
the emplacement of intermediate to silicic intrusions
(larvikites, syenites, granites). Parts of these
intrusions were derived from partial melting of
the crust.
Dyke intrusions
are common in the Oslo Graben and occurred over
the entire time-span of graben evolution. They
vary widely in size and composition, from mafic
to silicic. The compositions of the mafic dykes
resemble those of the basaltic lavas, i.e.
mostly alkaline, but some tholeiitic dykes exist
as well.
Discussion
Whether or not a
plume contributed to the origin of the Oslo Graben
has long been a debate among scientists. So far,
most studies point towards a plate-model solution
(Olaussen et al., 1994; Pedersen
& van der Beek, 1994; Heeremans
et al.,
1996; Heeremans & Faleide, 2004;
Pascal et al., 2004), but active contributions
from the asthenospheric mantle have not been ruled
out (Heeremans
et al.,
1996; Neumann et al., 2004). The
following sections address some important issues
related to the plume-plate discussion, some of
which are criteria used by Courtillot et al.
(2003) and Anderson (2005):
Age
progressive tracks: There is no
evidence at all that the Carboniferous-Permian
magmatism in NW Europe, including the Oslo Graben,
is related to an age progressive “hot-spot”
track as might be expected to be associated with
a plume. Paleomagnetic studies indicate that at
ca. 300 Ma, the area of interest was located approximately
10° north of the equator and ca. 10° east
of its current position (e.g., Wilson et al.,
2004). During the Carboniferous, the Laurussia
and Gondwana plates underwent rapid northward
motion (e.g., Torsvik & Cocks, 2004),
suggesting that if a plume was present a clear
“hot-spot” track should be present.
In addition, the combination of rapid plate motion
and long-lived, fixed magmatic activity does not
support a plume model.
Uplift:
An angular unconformity exists between the Asker
Group sediments and the underlying Cambro-Silurian
successions, which represent a time-span of ca.
100 m.y. During this time erosion took place,
but it did not remove the entire Cambro-Silurian
succession, since this succession is preserved
within the Oslo Graben proper. The deposition
of the Asker Group sediments indicates pre-rift
subsidence. Only after the termination of tectonomagmatic
activity in the Oslo Graben, erosion removed ca.
3-5 km of Palaeozoic rocks (Zeck et al.,
1988; Rohrman et al., 1994). All this
indicates that no pre-rift uplift occurred prior
to the development of the Oslo Graben, suggesting
the absence of a plume.
3He/4He:
No studies on 3He/4He have
been done directly on samples from the Oslo Graben,
due to the lack of mantle xenoliths. However,
olivines extracted from mantle xenoliths found
in Carboniferous-Permian dykes from Scotland,
contemporaneous with the B1 basalts of the Oslo
Graben, have been analysed for 3He/4He
(Kirstein et al., 2004). These studies
show that 3He/4He ±
1σ ratios vary from 0.97 ± 0.05 Ra
to 6.33 ± 0.21 Ra. (Ra is the atmospheric
ratio.) 3He/4He values higher
than ~10 Ra are postulated to be associated with
mantle plume activity (Courtillot et al.,
2003). These results thus argue for a non-plume
source for the Scottish magmatic rocks. Assuming
a genetic relationship between the Scottish basalts
and those in the Oslo Graben, suggests that a
similar conclusion can be drawn for the formation
of the most primitive basalts in the Oslo Graben.
[Ed: See also Helium
fundamentals page]
Large
Igneous Province: Large Igneous
Provinces are generally believed to represent
the start of plume activity and are often found
at the beginning of “hot-spot” tracks
(Courtillot et al., 2003; Anderson,
2005). Although the volumes of magmas produced
during the Carboniferous-Permian period in north-western
Europe are relatively large (estimates are: Oslo
Graben, 120,000 km3 total volume of
magmatic rocks; NW Germany, 48,000 km3
of volcanic rocks, Benek et al., 1996;
Neumann et al., 2004), they are small
compared to Large Igneous Provinces. In addition,
the time span of magmatic activity extends for
more then 60 m.y. in the Oslo Graben. Although
the magmatic activity occurred in pulses, the
overall magma production rate was fairly low (ca.
0.01 km3a-1).
Mantle
temperatures: Neumann
(1994) estimated the extrusion temperatures of
the basaltic lavas to be approximately 1270-1340°C.
This indicates normal or slightly elevated mantle
temperatures and suggests that the extensive Oslo
Graben magmatism was not associated with a major
temperature anomaly. Other alternative hypotheses
proposed by Neumann (1994) are:
-
a “wet-spot”,
-
decompression
melting due to lithospheric stretching, and
-
a combination
of causal mechanisms.
Numerical studies
focusing on the formation of the southern and
northern Permian Basins (van Wees et al.,
2000; Frederiksen et al., 2001), which
formed as a result of late Carboniferous-early
Permian rifting, indicate that a thermal event
occurred prior to the formation of these basins.
However, these authors do not discuss possible
temperature anomalies.
Other
related topics
Geochemical
studies: The geochemical signature
of the basaltic lavas in the Oslo Graben indicate
that they are derived from mantle sources of the
types tapped at ocean islands (HIMU, PREMA) (Neumann
et al., 2004) (Figure 3). However, melts
derived from refractory mantle beneath the continental
lithosphere may acquire such signatures through
interaction with metasomatized lithospheric mantle.
The lithospheric mantle melts beneath the Oslo
Graben were probably metasomatically enriched
by carbonatite fluids in early Palaeozoic times
in association with the formation of the Fen intrusion
(see for location Figure 2). Rocks from related
areas (Scotland, Scania, the central North Sea
and NW Germany) show similar geochemical signatures
(Neumann et al., 2004; Upton et al.,
2004). In the NW German Basin, 70% of the volcanic
succession consists of rhyolites. Their chemical
signature points to derivation from, or interaction
with, a lithospheric mantle source with a subduction-related
component (Benek et al., 1996).
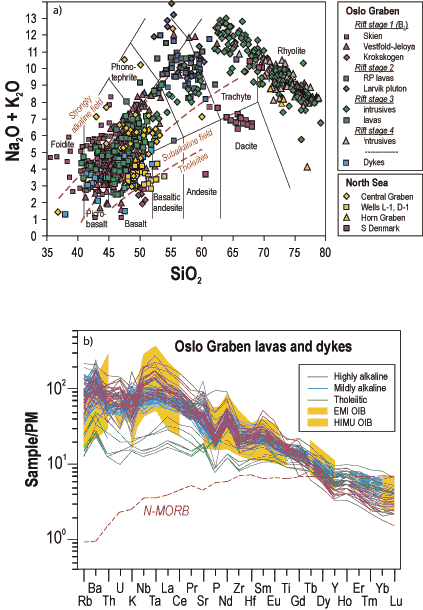 |
Figure
3: a) Petrochemical classification diagram
for Permo-Carboniferous magmatic rocks in
the Oslo Graben (after Le Bas et al. 1986),
based on Figure 6 in Neumann et al. (2004).
b) Trace element concentrations in mafic
lavas, dykes and sills in the Oslo Graben,
normalised to the primordial mantle (PM)
as defined by (Mcdonough and Sun, 1995)
based on Figure 7 in Neumann et al. (2004). |
Numerical
modelling studies: Numerical modelling
studies have shown that the Oslo Graben could
have formed in passive plate models (Pedersen
& van der Beek, 1994; Pascal et al.,
2004). Pedersen & van der Beek (1994)
presented a 1-dimensional model that includes
conduction and differential thinning of the lithosphere
and concluded that the Oslo Graben was not affected
by a mantle plume. However, their model assumed
a constant melt production over 60 m.y., indicating
the very low magma production rate of ca. 0.005
km3a-1. The model presented
by Pascal et al. (2004) shows the development
of the Oslo Graben as a genetic response to pre-existing
lithospheric structure. Their model shows significant
lithospheric thinning in a narrow zone below the
Oslo Graben, suggesting the possibility of small-scale
convection within the asthenospheric mantle (Figure
4). Unfortunately, due to limitations in the modelling
software, their model terminates when magma generation
starts.
Although these models
have their limitations, they indicate that tectonic
forces and pre-existing lithospheric structure
played an important role in the formation of the
Oslo Graben.
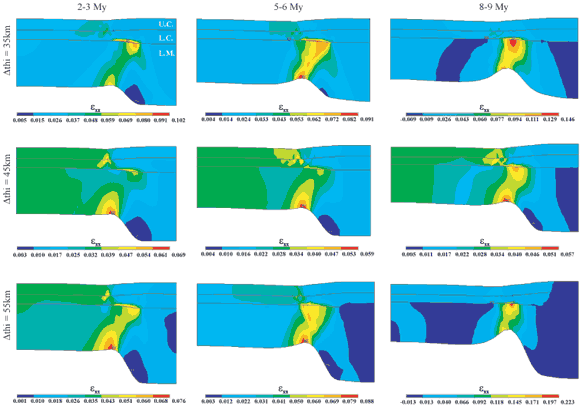
Figure 4: Horizontal
strain (exx) distributions computed
for different lithosphere thickness contrasts
(Δthi) across an east-west section over the
Oslo Graben at different times of the model run.
Positive strain values indicate stretching. The
models were stretched for 10 m.y. with a velocity
of 1.6 cm/a. Note the different scales for strain
in each figure. U.C., L.C. and L.M. are respectively
Upper Crust, Lower Crust and Lithospheric Mantle
(from Pascal et al., 2004). Click
on figure for enlargement.
Plate
configuration
A major factor that
must be taken into account when discussing the
tectonomagmatic evolution of the Oslo Graben and
related areas is the plate configuration at the
time of magmatic activity. The entire area has
undergone major lithospheric compression over
the last 100-150 m.y., resulting in heterogeneous
crustal and lithospheric thicknesses (e.g.,
Kinck et al., 1993). Two major collisions,
the Laurentia-Greenland – Baltica collision
(the Caledonian orogeny) and the Laurussia –
Gondwana collision (the Variscan orogeny) had
a major impact on the later development of the
area (Ziegler, 1990; Olaussen et
al., 1994; Veevers et al., 1994).
The area of interest is located exactly at the
intersection of the Caledonian and Variscan orogenies.
During the Variscan orogeny and its aftermath,
the foreland was strongly affected by strike-slip
tectonics with dextral transtensional and transpressional
movements along deep-seated pre-existing faults
(Ziegler, 1990; Coward, 1993;
Mogensen, 1994; Olaussen et al.,
1994; Veevers et al., 1994; Heeremans
et al.,
1996; Verdier, 1996). Extensional
continental basins developed, creating space for
the deposition of continental clastic material
(Ziegler, 1990; Heeremans & Faleide,
2004; Heeremans et al., 2004a). These
basins are interpreted as large extensional sag
basins caused by extensional tectonics and thermal
cooling after a large thermal event (van Wees
et al., 2000; Frederiksen et al.,
2001).
Distribution
of magmatic products
Carboniferous-Permian
magmatic activity in NW Europe was not evenly
distributed over space or time. The distribution
of alkaline and tholeiitic dykes with an age of
ca. 300-290 Ma in Scotland, Oslo and Scania, caused
Ernst & Buchan (1997) to suggest
a plume head below Jutland (Denmark). However,
the Scottish dyke swarm seems to terminate abruptly
in the central North Sea (Figure 1). No evidence
of other dyke activity has so far been discovered
on the east side of the Central Graben. Figure
1 shows that the distribution of the magmatic
successions seems to be controlled by fault-related
structures closely associated with the development
of the northern and southern Permian Basins (e.g.,
Heeremans et al., 2004a). This indicates
tectonic control on magma migration and possibly
also on magma generation.
Conclusion
Most
studies of the Oslo Graben and related areas,
do not show unambiguous evidence for the contribution
of a mantle plume in the tectonomagmatic development.
However, studies do show possible contributions
from asthenospheric melt sources. Numerical modelling
studies have shown that prior to the development
of the northern and southern Permian basins, a
strong thermal event occurred (van Wees et
al., 2000; Frederiksen et al., 2001).
Strong evidence against plume activity includes:
-
The lack
of an age-progressive volcanic track,
- pre-magmatic subsidence
instead of uplift,
-
low to moderate
3He/4He values,
-
the absence
of a large igneous province, and
-
normal or
only slightly elevated mantle temperature.
However, the widespread basaltic spike at ca.
300-290 Ma suggests a sudden, hot anomaly below
the area at the time of dyke and lava emplacement.
This anomaly has a considerable lateral extent,
of the order of 103 km.
Numerical modelling and careful examination
of the plate configuration and paleogeography
suggests that extensional tectonic activity
was the main driving force for basin development.
However, extension of a heterogeneous lithosphere
may result in asthenospheric mantle rising adiabatically
and a more active role for asthenospheric mantle
melts. This might also explain the uneven distribution
of magmatic rocks in the area.
Whether or not a plume was active beneath NW
Europe at the time of formation of the Oslo
Graben, is still an unresolved question. Most
studies favour a model in which plate tectonic
forces were responsible for rifting and consequent
magma generation resulted from lithospheric
thinning. However, an active role of a hot mantle
cannot be ruled out and a combination of different
models might explain the formation of the Oslo
Graben better.
|
-
Anderson,
D.L., 2005. Scoring hotspots: the plume &
plate paradigms, in: G.R. Foulger, J.H. Natland,
D.C. Presnall and D.L. Anderson (Editors),
Plates, Plumes and Paradigms, Geological
Society of America Special paper 388, in press.
-
Benek, R.,
Kramer, W., McCann, T., Scheck, M., Negendank,
J.F.W., Korich, D., Huebscher, H.D. and Bayer,
U., 1996. Permo-Carboniferous magmatism of
the Northeast German Basin, Tectonophysics,
266, 379-404.
-
Breitkreuz,
C. and Kennedy, A., 1999. Magmatic flare-up
at the Carboniferous/Permian boundary in the
NE German basin revealed by SHRIMP zircon
ages, Tectonophysics, 302,
307-326.
-
Courtillot,
V., Davaille, A., Besse, J. and Stock, J.,
2003. Three distinct types of hotspots in
the Earth's mantle, Earth and Planetary
Science Letters, 205,
295-308.
-
Coward, M.P.,
1993. The effect of late Caledonian and Variscan
continental escape tectonics on basement structure,
Paleozoic basin kinematics and subsequent
Mesozoic basin development in NW Europe, in:
J.R. Parker (Editor), Petroleum Geology
of Northwest Europe; Proceedings of the
4th conference, Geological Society of London,
London, United Kingdom, pp. 1095-1108.
-
Dahlgren,
S., Corfu, F. and Heaman, L.M., 1996. U-Pb
isotopic time constraints, and Hf and Pb source
characteristics of the Larvik plutonic complex,
Oslo Paleorift. Geodynamical and geochemical
implications for the rift evolution, V.M.
Goldschmidt Conference, Ruprecht-Karls
Universität, Heidelberg, Germany, pp.
120.
-
Dahlgren,
S. and Corfu, F., 2001. Northward sediment
transport from the late Carboniferous Variscan
Mountains: zircon evidence from the Oslo Rift,
Norway, Journal of the Geological Society,
158, 29-36.
-
Ernst, R.E.
and Buchan, K.L., 1997. Giant radiating dyke
swarms; their use in identifying pre-Mesozoic
large igneous provinces and mantle plumes,
in: J.J. Mahoney and M.F. Coffin (Editors),
Large igneous provinces; continental,
oceanic, and planetary flood volcanism,
Geophysical Monograph, 100, American Geophysical
Union, Washington, DC, United States, pp.
297-333.
-
Frederiksen,
S., Nielsen, S.B. and Balling, N., 2001. A
numerical dynamic model for the Norwegian-Danish
Basin, Tectonophysics, 343,
165-183.
-
-
Heeremans,
M. and Faleide, J.I., 2004. Late Carboniferous-Permian
tectonics and magmatic activity in the Skagerrak,
Kattegat and the North Sea, in: M. Wilson,
E.R. Neumann, G.R. Davies, M.J. Timmerman,
M. Heeremans and B.T. Larsen (Editors), Permo-Carboniferous
Magmatism and Rifting in Europe, Geological
Society Special Publication, 223, The Geological
Society, London, pp. 157-176.
-
Heeremans,
M., Faleide, J.I. and Larsen, B.T., 2004a.
Late Carboniferous - Permian of NW Europe:
an introduction to a new regional map, in:
M. Wilson, E.R. Neumann, G.R. Davies, M.J.
Timmerman, M. Heeremans and B.T. Larsen (Editors),
Permo-Carboniferous Magmatism and Rifting
in Europe. Geological Society Special
Publication, The Geological Society, London,
pp. 75-88.
-
Heeremans,
M., Timmerman, M.J., Kirstein, L.A. and Faleide,
J.I., 2004b. New constraints on the timing
of late Carboniferous - early Permian volcanism
in the central North Sea, in: M. Wilson, E.R.
Neumann, G.R. Davies, M.J. Timmerman, M. Heeremans
and B.T. Larsen (Editors), Permo-Carboniferous
Magmatism and Rifting in Europe, Geological
Society Special Publication, 223, The Geological
Society, London, pp. 177-194.
-
Henningsmoen,
G., 1978. Sedimentary rocks associated with
the Oslo region lavas. In: J.A. Dons and B.T.
Larsen (Editors), The Oslo Paleorift;
a review and guide to excursion, Bulletin
- Norges Geologiske Undersøkelse, 337.
Universitetsforlaget, pp. 17-24.
-
Kinck, J.J.,
Husebye, E.S. and Larsson, F.R., 1993. The
Moho Depth Distribution in Fennoscandia and
the Regional Tectonic Evolution from Archean
to Permian Times, Precambrian Research,
64, 23-51.
-
Kirstein,
L.A., Dunai, T.J., Davies, G.R., Upton, B.G.J.
and Nikogosian, I.K., 2004. Helium isotope
signature of lithospheric mantle xenoliths
from the Permo-Carboniferous magmatic province
in Scotland - no evidence for a lower-mantle
plume, in: M. Wilson, E.R. Neumann, G.R. Davies,
M.J. Timmerman, M. Heeremans and B.T. Larsen
(Editors), Permo-Carboniferous Magmatism
and Rifting in Europe, Geological Society
Special Publication, 223, The Geological Society,
London, pp. 243-258.
-
Mcdonough,
W.F. and Sun, S.S., 1995. The Composition
of the Earth, Chemical Geology, 120,
223-253.
-
Mogensen,
T.E., 1994. Paleozoic Structural Development
along the Tornquist Zone, Kattegat Area, Denmark,
Tectonophysics, 240,
191-214.
-
Monaghan,
A.A. and Pringle, M.S., 2004. 40Ar/39Ar
geochronology of Carboniferous-Permian volcanism
in the Midland Valley, Scotland, in: M. Wilson,
E.R. Neumann, G.R. Davies, M.J. Timmerman,
M. Heeremans and B.T. Larsen (Editors), Permo-Carboniferous
Magmatism and Rifting in Europe, Geological
Society Special Publication, 223, The Geological
Society, London, pp. 219-241.
-
Neumann, E.R.,
1994. The Oslo Rift - P-T Relations and Lithospheric
Structure, Tectonophysics, 240,
159-172.
-
Neumann, E.R.,
Wilson, M., Heeremans, M., Spencer, E.A.,
Obst, K., Timmerman, M.J. and Kirstein, L.,
2004. Carboniferous-Permian rifting and magmatism
in southern Scandinavia, the North Sea and
northern Germany: a review, in: M. Wilson,
E.R. Neumann, G.R. Davies, M.J. Timmerman,
M. Heeremans and B.T. Larsen (Editors), Permo-Carboniferous
Magmatism and Rifting in Europe, Geological
Society Special Publication, 223, The Geological
Society, London, pp. 11-40.
-
Olaussen,
S., 1981. Marine Incursion in Upper Paleozoic
Sedimentary-Rocks of the Oslo Region, Southern-Norway,
Geological Magazine, 118,
281-288.
-
Olaussen,
S., Larsen, B.T. and Steel, R., 1994. The
Upper Carboniferous-Permian Oslo Rift; basin
fill in relation to tectonic development,
in: A.F. Embry, B. Beauchamp and D.J. Glass
(Editors), Pangea; global environments
and resources, Memoir - Canadian Society
of Petroleum Geologists, 17. Canadian Society
of Petroleum Geologists, Calgary, AB, Canada,
pp. 175-197.
-
Pascal, C.,
Cloetingh, S. and Davies, G.R., 2004. Asymmetric
lithosphere as the cause of rifting and magmatism
in the Permo-Carboniferous Oslo Graben, in:
M. Wilson, E.R. Neumann, G.R. Davies, M.J.
Timmerman, M. Heeremans and B.T. Larsen (Editors),
Permo-Carboniferous Magmatism and Rifting
in Europe, Geological Society Special
Publication, 223, The Geological Society,
London, pp. 139-156.
-
Pedersen,
T. and van der Beek, P., 1994. Extension and
magmatism in the Oslo rift, southeast Norway:
no sign of a mantle plume, Earth and Planetary
Science Letters, 123,
317-29.
-
Ramberg, I.B.
and Larsen, B.T., 1978. Tectonomagmatic evolution,
in: J.A. Dons and B.T. Larsen (Editors), The
Oslo Paleorift: a review and guide to excursions,
Bulletin - Norges Geologiske Undersokelse,
337, Universitetsforlaget, Trondheim, pp.
55-73.
-
Ro, H.E.,
Larsson, F.R., Kinck, J.J. and Husebye, E.S.,
1990. The Oslo Rift - Its Evolution on the
Basis of Geological and Geophysical Observations,
Tectonophysics, 178,
11-28.
-
Rohrman, M.,
van der Beek, P. and Andriessen, P., 1994.
Syn-rift thermal structure and post-rift evolution
of the Oslo Rift (southeast Norway): new constraints
from fission track thermochronology, Earth
and Planetary Science Letters, 127,
39-54.
-
Sundvoll,
B., Neumann, E.R., Larsen, B.T. and Tuen,
E., 1990. Age Relations among Oslo Rift Magmatic
Rocks - Implications for Tectonic and Magmatic
Modeling, Tectonophysics, 178,
67-87.
-
Sundvoll,
B., Larsen, B.T. and Wandaas, B., 1992. Early
magmatic phase in the Oslo Rift and its related
stress regime, Tectonophysics, 208,
37-54.
-
Sundvoll,
B. and Larsen, B.T., 1993. Rb-Sr and Sm-Nd
relationship in dyke and sill intrusions in
the Oslo Rift and related areas, Bulletin
- Norges geologiske undersøkelse,
425, 23-40.
-
Timmerman,
M.J., 2004. Timing, geodynamic setting and
character of Permo-Carboniferous magmatism
in the foreland of the Variscan Orogeny, NW
Europe, in: M. Wilson, E.R. Neumann, G.R.
Davies, M.J. Timmerman, M. Heeremans and B.T.
Larsen (Editors), Permo-Carboniferous
Magmatism and Rifting in Europe, Geological
Society Special Publication, 223. The Geological
Society, London, pp. 41-74.
-
Torsvik, T.H.
and Cocks, L.R.M., 2004. Earth geography from
400 to 250 Ma; a palaeomagnetic, faunal and
facies review, Journal of the Geological
Society of London, 161,
555-572.
-
Upton, B.G.J.,
Stephenson, D., Smedley, P.M., Wallis, S.M.
and Fitton, J.G., 2004. Carboniferous and
Permian magmatism in Scotland, in: M. Wilson,
E.R. Neumann, G.R. Davies, M.J. Timmerman,
M. Heeremans and B.T. Larsen (Editors), Permo-Carboniferous
Magmatism and Rifitng in Europe, Geological
Society Special Publication, 223, The Geological
Society, London, pp. 195-218.
-
van Wees,
J.D., Stephenson, R.A., Ziegler, P.A., Bayer,
U., McCann, T., Dadlez, R., Gaupp, R., Narkiewicz,
M., Bitzer, F. and Scheck, M., 2000. On the
origin of the Southern Permian Basin, Central
Europe, Marine and Petroleum Geology,
17, 43-59.
-
Veevers, J.J.,
Clare, A. and Wopfner, H., 1994. Neocratonic
magmatic-sedimentary basins of post-Variscan
Europe and post-Kanimblan eastern Australia
generated by right lateral transtension of
Permo-Carboniferous Pangea, Basin Research,
6, 141-157.
-
Verdier, J.P.,
1996. The Rotliegend sedimentation history
of the southern North Sea and adjacent countries,
in: H.E. Rondeel, D.A.J. Batjes and W.H. Nieuwenhuijs
(Editors), Geology of Gas and Oil under
the Netherlands, Kluwer Academic Publisheres,
Dordecht, pp. 45-56.
-
Wilson, M.,
Neumann, E.R., Davies, G.R., Timmerman, M.J.,
Heeremans, M. and Larsen, B.T., 2004. Permo-Carboniferous
Magmatism and Rifting in Europe, Geological
Society Special Publication, 223, The Geological
Society, London, 498 pp.
-
Zeck, H.P.,
Andriessen, P.A.M., Hansen, K., Jensen, P.K.
and Rasmussen, B.L., 1988. Paleozoic paleo-cover
of the southern part of the Fennoscandian
Shield-fission track constraints, Tectonophysics,
149, 61-6.
-
Ziegler, P.A.,
1990. Geological Atlas of Western and
Central Europe, Shell Internationale
Petroleum Maatschappij, The Hague.
|
last updated
30th June, 2005 |
|
|
|