 |
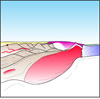 |
Tectonic
and deep crustal structures along the Norwegian
volcanic margin: Implications for the “Mantle
Plume or Not?” debate
|
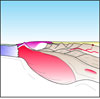
|
|
Laurent
Gernigona, Sverre
Plankeb, Jean-Claude
Ringenbachc & Bernard
Le Galld
aNorges
Geologiske Undersøkelse (NGU), Geological Survey
of Norway, leiv Erikssons vei 39, N-7491 Trondheim,
Norway
bVolcanic Basin Petroleum Research (VBPR),
Forskningsparken, Gaustadalléen 21, N-0349 Oslo,
Norway
cTotalFinaElf GSR/PN, 2, place de la Coupole
- La Défense 6 - 92078 Paris, France
dInstitut Universitaire Européen de
la Mer, Place Nicolas Copernic, 29280 Plouzané,
France
Corresponding
author email: Laurent.Gernigon@ngu.no
or gernigon_geol@hotmail.com
Co-author emails: vbpr@vbpr.no – Jean-Claude.RINGENBACH@total.com – blegall@univ-brest.fr
|
Abstract
High P-wave velocities
(7.1-7.8 km/s) in lower crustal bodies (LCBs) imaged
along volcanic margins are commonly interpreted
as plume- and breakup-related thick mafic underplating.
This interpretation is partially challenged in this
paper on the basis of new seismic observations on
the outer Vøring
Basin (Norway). An exceptionally strong reflection,
the T Reflection, is particularly well defined below
the north Gjallar Ridge (NGR). It is located near
the volcanic traps formed during the NE Atlantic
breakup (at ~55-54 Ma). The T Reflection coincides
with the top of an LCB, forming a mid-crustal dome.
Based on structural and temporal relationships,
we show that the dome clearly influences the structural
development of the NGR and predates the continental
breakup by at least 10-15 Ma. We conclude that the
continental part of the LCB observed beneath the
outer Vøring
Basin does not necessarily originate with an anomalous
Tertiary magmatic event triggered by an “Icelandic
mantle plume” (underplating). Instead we
attribute it partly or fully to inherited, high-pressure
granulite/eclogite lower crustal rocks in the
continental domain. Because the real amount of
mafic material emplaced could be 20-40% less than
is thought, this has major implications for the
plume/non-plume debate.
|
1. The Outer
Vøring Basin: a key area for understanding
the lower crustal body (LCB) and relationship to the
plume/non-plume question
1.1. Volcanic
margins: general concepts
Volcanic margins form part of large
igneous provinces, which are characterised by massive
emplacements of mafic extrusives and intrusive rocks
over very short time periods (White & McKenzie,
1989; Holbrook & Keleman, 1993; Mjelde
et al., 1997, 1998, 2002; Eldholm et al.,
2000; Menzies et al., 2002). Volcanic margins
are distinguished from non-volcanic margins (or “cold”
margins, e.g. the Iberian margin) which do not contain
large amounts of extrusive and/or intrusive rocks
and may exhibit unusual features such as unroofed,
serpentinized mantle (Boillot & Froitzheim,
2001) (Figure 1). Volcanic margins are known to differ
from classical passive margins in a number of ways,
the main ones being:
-
a huge volume of
magma forms during the early stages of crustal accretion
along the future spreading axis, typically as seaward-dipping
reflector sequences (SDRs),
-
the presence of
numerous sill/dike and vent complexes intruding
the sedimentary basin,
-
the lack of strong
passive-margin subsidence during and after breakup,
and
-
the presence of
lower crust with anomalously high seismic P-wave
velocities (7.1-7.8 km/s) – so-called lower
crustal bodies (LCBs) (Planke et al., 1991;
Eldholm et al., 2000) (Figure 1).
The high velocities (Vp > 7 kms)
and large thicknesses of the LCBs are often used to
support the theory of “hot” mantle plume
involvement leading to the formation of a huge volume
of magmatic rocks (White & McKenzie, 1989;
Eldholm & Grue, 1994). LCBs are often
located along the continent-ocean transition but can
extend beneath the continental part of the crust (Figure
1). In the continental domain, there are fewer constraints
on their nature and chronology. Better constraints
of the timing of LCB emplacement, seismic velocities,
size and geological context are pertinent to the plume/non-plume
debate.
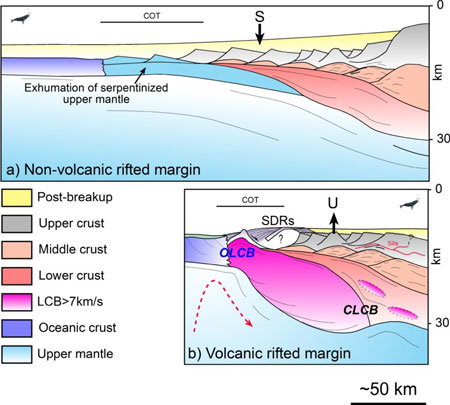
Figure 1: Main characteristics
of volcanic margins versus non-volcanic passive margins.
(a) Schematic crustal section of a wide non-volcanic
“Galician type” margin characterised by
the progressive exhumation of the underlying seprentinized
mantle (Boillot & Froitzheim, 2001). (b) Structure
and main characteristics of a narrow volcanic “Vøring
type” margin. CLCB: continental lower-crustal
body; OLCB: oceanic lower-crustal body; SDRs: Seaward
Dipping Reflector sequences. S symbolizes the post-breakup
subsidence of the non-volcanic margin, U represents
the relative uplift recorded along the volcanic margin
as an isostatic consequence of thick high velocity
underplating observed along the continent-ocean transition
(COT).
1.2. The
outer Vøring Basin: a lower crustal window
near the Tertiary lava flows
SDRs and LCBs have long been recognized
along the NE Atlantic and particularly in the outer
Vøring Basin (Figure 2). The outer Vøring
Basin is a complex system of faulted ridges defined
at the base Tertiary unconformity level. It is located
between a deep Cretaceous basin to the east and the
Vøring Marginal High to the west near the ocean-continent
transition (Lundin & Doré, 1997;
Walker et al., 1997; Mjelde et al.,
1997, 1998, 2002; Ren et al., 1998; Gernigon
et al., 2003,
2004)
(Figures 2, 3). As part of the polyrifted system,
the outer Vøring Basin was particularly affected
by Late Cretaceous-Paleocene rifting leading to breakup
and SDR emplacement at ~54-55 Ma (Figures 2, 3).
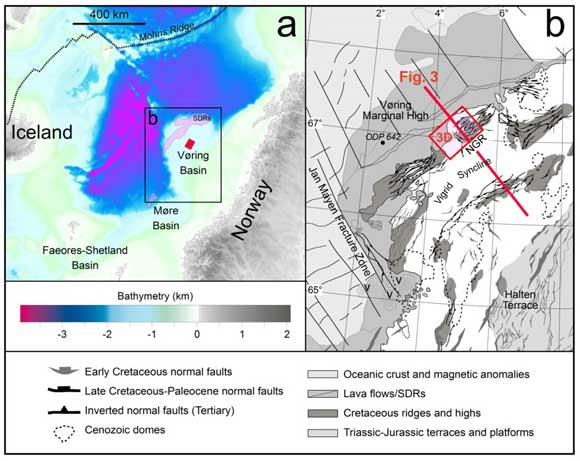
Figure 2: a) Bathymetric
map of the Vøring Margin and location of the
study area. b) Structural map of the outer Vøring
Basin. Bathymetric data from Sandwell & Smith
(1997). Red rectangle on b) represents the 3D seismic
survey used for the investigation. Lava flows, SDRs,
after Berndt et al. (2001). Modified from Gernigon
et al. (2003).
The Vøring Margin is particularly
interesting because a huge amount of geophysical data
exist (refraction, 2D/3D seismic) which has bearing
on the shallow manifestation of both lithospheric
and asthenospheric postulated processes (e.g. mantle
plume and small-scale convection; see also The
Origin of the Iceland Hotspot). It is also a good
study area because relationships between magmatism,
LCBs and the sedimentary basin can be investigated
with confidence, as extrusive sequences are relatively
narrow.
Here, we focus on the north Gjallar
Ridge (NGR), which is located between the Vigrid Syncline
and the Vøring Marginal High (Figure 3) (Lundin
& Doré, 1997; Ren et al.,
1998, Gernigon et al., 2003,
2004).
In particular, one of the most interesting features
of the NGR concerns a mid-crustal dome-shaped reflection
underlying the NGR, mapped regionally and named the
T Reflection (Gernigon et al., 2004) (Figures
4, 5). Recent investigations suggest that the T Reflection
demarcates the top of the continental LCB (Walker
et al., 1997; Ren et al., 1998; Mosar,
2002; Gernigon et al. 2003,
2004).
Earlier interpretations directly assigned the crustal
dome beneath the outer Vøring Basin to magmatic
underplating (T Reflection = top of the LCB = top
of the Tertiary breakup-related underplating; Eldholm
& Grue, 1994; Mjelde et al., 1997,
1998, 2002), or a metamorphic core complex triggered
by underlying magma chambers (Lundin & Doré,
1997). In this paper, we present and discuss the following
aspects of the LCB:
-
the 3D geometry
and geophysical properties of the crustal structure
underlying the NGR,
-
the relation between
the T Reflection, LCB and NGR structures,
-
the tectonic and
temporal evolution of the NGR including issues related
to lithospheric rupture, “mantle plume”
theory and LCB emplacements, and
-
the controversial
nature of continental LCBs and implications for
understanding of the tectonics of volcanic margins
and asthenospheric processes in general.
|
2. Magmatism,
LCBs and basin deformation: a structural approach
2.1. The
north Gjallar Ridge (NGR) and the T Reflection
The T Reflection is observed
in a large part of the NGR at the base Tertiary level
(e.g. Lundin & Doré, 1997; Ren,
1998) (Figures 3, 4). Gernigon
et al.
(2003) show that the T Reflection extends over a
large part of the outer Vøring Basin, and is
limited to the east by the Fles Fault Complex (Figure
3). The 3D geometry of the T Reflection is now fully
constrained on the NGR by 2D/3D seismic surveys, where
it comprises a rounded feature 20 km in diameter with
a thickness between 7 and 8 s two-way travel time. The
T Reflection is shallower than the present-day Moho
estimated from ocean-bottom seismometer (OBS) data to
be at 20 km depth (Raum, 2000) (Figure 3).
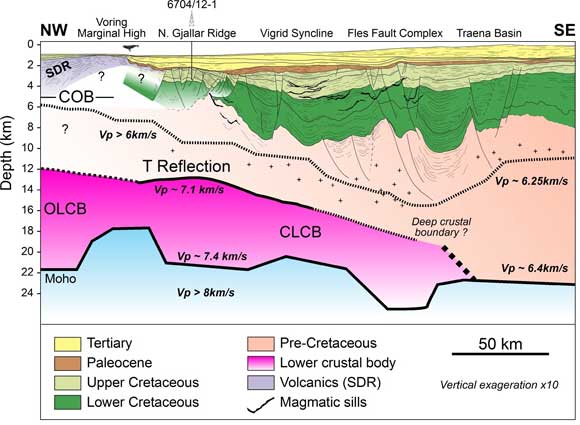
Figure 3: Depth-converted cross-section
of the outer Vøring Basin. The north Gjallar
Ridge (NGR) is located close to the breakup volcanic
rocks defined by the SDRs of the Vøring Marginal
High. The T Reflection observed in 2D seismic data match
the top of the lower crustal body (7 km/s) defined by
Raum (2000).
The top of the T Reflection,
repositioned in the OBS depth model, is at 13-14 ±
2 km depth. Ren et al. (1998) and Skogseid
et al. (2000) also suggest that the top of the
T Reflection lies between 10 and 15 km, matching the
top of the continental part of the LCB. Mjelde et
al. (1997) and Raum (2000) suggest that
the T Reflection marks the top of the interval with
Vp > 7.1 km/s, and interpret it as the top of mafic/ultramafic
underplated material, thereby invoking the underplating
hypothesis. On the basis of geophysical observations
Gernigon
et al.
(2004) show that the T Reflection represents a high-impedance
boundary associated with a high-density body (with a
high velocity contrast) but litle magnetic susceptibility.
This does not favour a mafic/ultramafic origin (Figure
4).
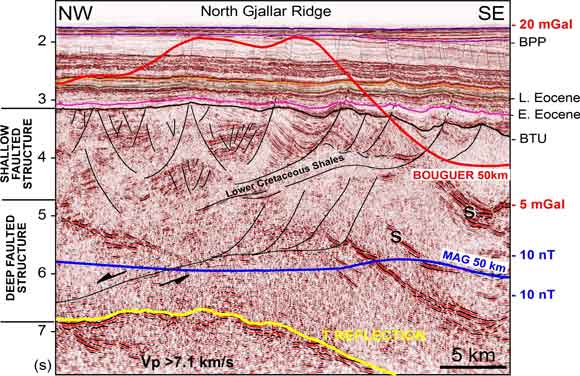
Figure 4: Reflection
seismic line (from the NGR Saga 3D survey) showing
the crustal structure of the NGR. The cross-line
illustrates the rollover geometry of the NGR, defined
by the base Tertiary unconformity (BTU) and controlled
in depth at a decollement level. During the early
Campanian-early Paleocene, extension at shallow depth
is characterised by rollover geometry accommodated
in depth by a detachment fault zone connected with
the T reflection. S represents magmatic sills. After
Gernigon et al. (2004). The blue curve represents the total
magnetic field and the red curve represents the Bouguer
gravity anomaly.
2.2. Structural
and tectonic evolution of the NGR
Different structural levels record different
types of stretching during NGR evolution from rifting
to breakup (Figures 4, 5 and Table 1).
-
The upper
level shows that syntectonic wedges and tilted blocks
formed during early Campanian-Paleocene time (well
calibration) and were accommodated at depth by a
decollement layer within lower Cretaceous (Albian-Cenomanian?)
shales. The shape of the dome defined by the T Reflection
controls the fault patterns which mostly focus around
the dome.
-
The middle
level shows block structures (poorly imaged) cut
by a large low-angle ductile shear zone at depth,
which splays upwards into normal faults. Normal
displacement along this low-angle shear zone is
expected prior to breakup rifting in order to accommodate
extension of the overlying tilted blocks.
-
The deepest
level shows the updomed T Reflection. The dome may
have accentuated block rotation and low-angle faulting,
in a similar way to the isostatic denudation and
rolling-hinge process. Extension on low-angle faults
is known to accommodate large amounts of crustal
stretching in rifted basins. The deduction that
low-angle shear zones exist in the deeper part of
the NGR is not surprising since extension in the
shallow part of the Cretaceous basin cannot accommodate
the severe crustal thinning thought to have occurred
just prior to lithospheric rupture (Figure 5).
Faulting in the NGR is
sealed at the erosive base Tertiary unconformity (uplift
~ 550-600 m), draped by Upper Paleocene-Early Eocene
sediments. This pre-breakup regional unconformity, which
is observed all along the NE Atlantic, has been interpreted
in terms of the Icelandic plume – lithosphere
impingement hypothesis (regional uplift) proposed by
Skogseid et al. (2000) to have occurred during the late
Maastrichtian-Early Paleocene (Table 1, Figure 5).
-
Before
the late Maastrichtian-Early Paleocene uplift, the
NGR was already a structural high above the crustal
dome (Figure 5a) which was already in place before
the breakup and the main volcanic event in Early
Eocene time (Figure 5c). Our key argument is that
the LCB is a pre-breakup feature.
-
Faulting
in the NGR stops before the Early Eocene breakup
and reflects a progressive or sudden focus of the
deformation toward the future breakup zone. This
migration of deformation occurred from early to
late Paleocene-early Eocene time (Figure 5b).
The early Paleocene also
marks the onset of magmatism (NE Atlantic magmatic phase
1 of Saunders et al., 1997). This strongly
suggests that early magmatic melts were most likely
involved in the breakup process. The pre-breakup focus
of extensional deformation may be interpreted as a result
of weakening of the lithosphere (perhaps due to ponding,
underplating and diking) as suggested by models showing
that a melted zone within the lithosphere may strongly
control the localization of stretching and necking (Geoffroy,
1998; Callot et al., 2002; see also Giant
Dike Patterns page).
Table 1: Chronological
synthesis of tectono-magmatic events from rifting to
breakup.
Periods |
Age
(Ma) |
Tectono-magmatic
events |
E.
Campanian-E. Maastrichtian |
80-70 |
Initiation
of the late rifting phase (late Cretaceous-Paleocene)
No evidence for magmatism |
E.
Maastrichtian-L. Maastrichtian |
70-66 |
Climax
of late Cretaceous-Paleocene continental rifting
Uplift and faulting along the North
Gjallar Ridge. |
Latest
Maastrichtian-Danian |
66-60 |
Regional
uplift of the NE Atlantic (plume?)
Maximum (?) erosion of the Maastrichtian
High located above the dome.
Progressive focus of faulting toward
the proto-oceanic axis
First evidence of alkaline magmatism |
Selandian |
60-55 |
Evidence
of Late Paleocene sediment above most of the
high previously eroded |
Latest
Paleocene-Ypresian |
55-53 |
Transient
volcanism related to the breakup (C24)
Second phase of uplift recorded
in the North Gjallar Ridge |
E.
Eocene-Mid. Eocene |
53-50 |
Decrease
in the magmatism & rapid relative
subsidence |
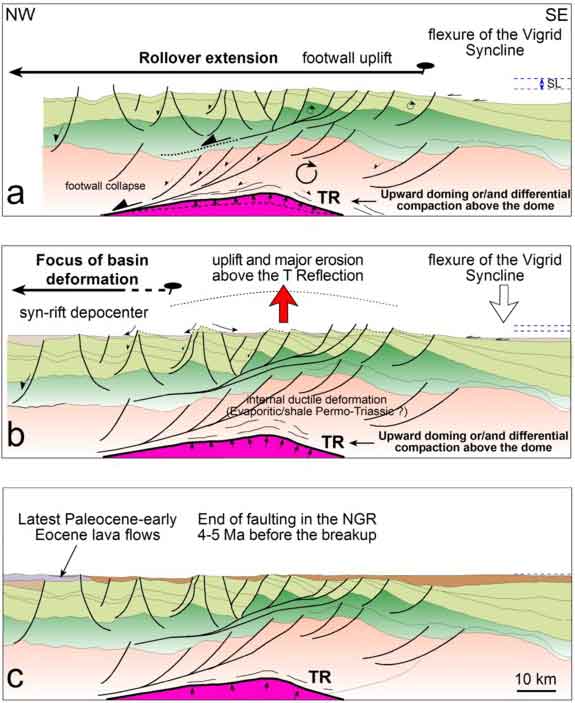
Figure
5: Three-stage kinematic model for the evolution of
the NGR from the early Campanian (~ 80 Ma) (a) to the
latest Maastrichtian-Early Paleocene main uplift (~
60 Ma), and (b) up to the final breakup in the latest
Paleocene-earliest Eocene (~ 55 Ma). (c) Onset of magmatism
in early Paleocene time that coincides with migration
of the deformation toward the pre-breakup axis. The
NGR was already influenced by the T Reflection (= top
LCB) before the breakup and the first evidence of magmatism.
This model suggests that the LCB beneath the NGR is
a pre-preakup feature.
|
3.
Discussion: the origin of the LCB: a breakup/plume-related
feature?
3.1. LCBs and implications
for the plume/non-plume debate
In the oceanic domain and along the
continent-ocean transition LCBs most likely represent
magmatic rocks but the significance of the continental
LCBs beneath the rift zone of the outer Vøring
Basin is controversial (Figure 6):
- What do we really know about the geological meaning
of the LCB?
- Are continental LCBs really magmatic features?
- Are LCBs fully representative of breakup-related
underplating?
- Do we necessarily need a mantle plume to generate
underplating?
- Do high P-wave velocities values really
reflect hot mantle temperatures (picrites).
What are the relationships between
LCB, basin deformation and continental breakup? In the
case of the NGR and volcanic margins in general, the
answers to these questions could lead to a better estimate
of the total amount of melt produced during breakup.
An improved volume estimate would have significant implications
for quantification of mantle temperatures and dynamics,
which are still poorly constrained (Figure 6).
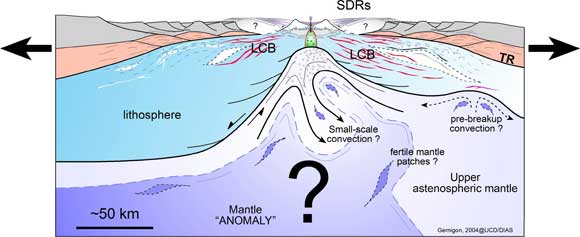
Figure 6: Conceptual cross-section
along conjugate volcanic margins during breakup. Volcanism
and underplating attributed to the whole LCB are due
to complex interactions between lithospheric extension
and controversial sub-lithospheric processes involving
a mantle “anomaly”. The most popular hypothesis
for continental breakup magmatism and SDR formation
is the arrival of a mantle plume originating from the
core-mantle boundary. The plume impact hypothesis attributes
anomalous mantle melting to a “hot” thermal
anomaly in convecting mantle. Alternative hypotheses
involve small-scale convection and circulation of fertile
and heterogeneous mantle that also result in a high
degree of melting. Click
here for enlarged figure.
3.2.
The meaning of the continental part of the LCB beneath
the NGR: mafic underplating or not?
Regional considerations show that the
magmatic activity in the NE occurred throughout the
entire Paleocene between 63 and 54 Ma with a peak at
50-55 Ma (Saunders et al., 1997). In view of
the high-velocity character of the lower crust and its
position close to the SDRs, a mafic/ultramafic interpretation
was proposed by Mjelde et al. (1997, 1998)
to explain the high Vp values observed along the breakup
axis and below the NGR.
According White & McKenzie
(1989), high-Mg underplated bodies characterised by
high-velocity lower crust should be a consequence of
the “Icelandic mantle plume” impingement
on the base of the lithosphere. At ~ 65-60 Ma, this
interaction is expected beneath Greenland located ~
500-1000 km away from the study area (Lawver &
Müller, 1994). According to this “Icelandic
mantle plume” model, direct thermal involvement
of the Norwegian margin is not expected before Early
Tertiary time.
-
If
we assume that a significant amount of underplating
requires the presence of a mantle plume, then the
T Reflection and the LCB are unlikely to have originated
from the top of the “Tertiary” magmatic
underplated unit, since it is clearly demonstrated
that they existed prior to the Paleocene.
It is, however, not clear if picritic
magmas are really related to high mantle temperatures
(Anderson
& Natland,
in press). Sheth (1999), for reference,
denounces the “basic fallacy of the surported
picrite-plume connection …… obscure at best”.
Picritic magmas do not necessarily characterise high-degree
or high-temperature melts but could simply be explained
by extensive decompression of an uprising mantle (active
or passive) and later differentiation.
Mjelde et al. (2002) suggest
that the LCB in the Vøring Basin is decoupled
from the breakup itself (oceanic/transitional LCB and
continental LCB), and could be restricted to a process
that occurred during the latest phase of rifting prior
to breakup. This could mean that significant lower-crustal
intrusions might have been formed during the Late Cretaceous.
In this case, our evolution model of the NGR could be
used as an argument in favor of quite significant Campanian-Maastrichtian
(pre-breakup) underplating, trapped beneath the Vøring
Basin.
Some geodynamic models can explain
moderate amounts of pre-breakup magmatism without involving
any mantle plume effect. Moderate temperature, fertile
mantle patches (e.g. eclogites) in the upper mantle,
and small-scale convection may explain significant pre-
and syn-breakup melt production (Figure 6) (e.g.
Boutillier & Keen, 1999; Anderson et al.,
2000; van Wijk et al., 2001; Korenaga,
2002, 2004; Anderson
& Natland,
2005; Foulger et al., 1995a,b;
Lundin
and Doré,
2005; http://www.mantleplumes.org/).
-
The
T Reflection may represent the top of a significant
pre-breakup (syn-rift?) underplating unit. Significant
pre-breakup underplating may not be directly linked
to a “hot mantle plume” but might result
from other sub-lithospheric processes that interact
with the rift system. However, if the LCB beneath
the NGR really represent mafic material, the non-magnetic
nature of the mid-crustal dome is difficult to explain.
3.3.
Alternative “non-magmatic” hypothesis
Another geological model that may account
for both the non-magnetic and high-velocity characteristics
of the NGR lower crust is that this layer consists of
pre-breakup crystalline rocks. The non-magmatic lower
continental crust below the outer Vøring Basin
is generally interpreted as granodioritic with P-wave
velocities ranging between 6.5 and 7 km/s (Mjelde
et al., 1997; 1998; Raum, 2000). However,
it has been observed that the lower crust has locally
higher velocities.
Serpentinised mantle displays a large
range of P-wave velocities, ranging between
5 and 7.5 km/s and high Vp/Vs values > 1.8 (O'Reilly
et al., 1996). These values are quite similar to
those observed below the T Reflection. The T Reflection
may represent the top of a sepentinised mantle but such
rocks are only expected to occur within highly stretched
crusta (Figure 1). Such environments are difficult to
explain at depths of 12-15 km (decompacted depth without
post-rift sediments laid down during the last stage
of rifting) below the NGR. The presence of serpentinised
mantle below the T Reflection has already been suggested
by Ren et al. (1998). The same assumption was
recently published in Mjelde et al. (2002)
based on Vp/Vs results and a discussion of the large-scale
crustal stretching of the Vøring Margin.
Along the outer Vøring Basin,
the LCB is limited to the east by the Fles Fault Complex
(Figure 3) known to be a major zone of weakness active
during the long tectonic history of the Norwegian margin
(Doré et al., 1997). It cannot be excluded
that the Fles Fault Complex may reflect a deep suture
zone between different crustal terranes and this could
also explain the velocity contrasts of the deep lower
crust beneath the Vøring Basin.
High-pressure granulite/eclogitic
material is known to have both high P-wave
velocity (7.2-8.5 km/s) and high density (2.8-3.6
g/cm3; Fountain et al., 1994).
These rocks are well documented in the eastern part
of the Norwegian Western Gneiss Region, outcropping
in the footwall of the Hornelen post-orogenic basin
(Dewey et al.,
1993; Figure 8). Its offshore continuation to the
west has been recently deduced in the northern North
Sea below the Triassic-Jurassic rift system (Christiansson
et al., 2000) and
in the eastern part of the Møre Basin (Olafsson
et al., 1992). Unpublished Expanded Spread Profiles
shot during the Elf Refranorge project (1983-1986)
also demonstrate that the geophysical nature of the
lower crust in the eastern part of the Vøring
margin is characterised by high P-wave velocity
values (> 7 km/s) less than 20 km from the Trøndelag
Platform (e.g. Planke et al., 1991). These
intermediate velocity values are also difficult
to interpret either as magmatic underplated or serpentinised
mantle because both features are generally focused
close to the breakup axis. The geophysical properties
of the Caledonian nappes also display low magnetic
succeptibilities and normal shelf-type thermal gradients
(Olesen
et al., 1997).
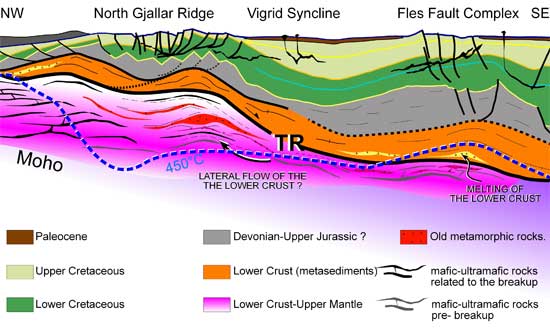
Figure 7: Crustal model proposed
to explain the LCB along the outer Vøring Basin
(at the Paleocene stage). Figure 8 (below) illustrates
eclogite outcropping below the Devonian Hornelen Basin,
onshore Norway.
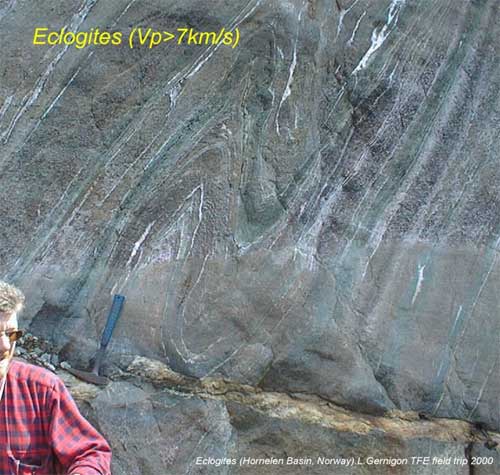
Figure 8: Eclogites (Hornelen Basin,
Norway). L. Gernigon TFE field trip 2000. |
4. Conclusions
-
3D
seismic data and data from borehole calibrations
reveal pre-breakup early-Campanian-Paleocene rifting
event along the north Gjallar Ridge (NGR). The T
Reflection represents the top of the continental
part of an LCB.
-
The
T Reflection influenced the structural development
of the sedimentary basin at least 4-10 Myr prior
to breakup. Our observations clearly demonstrate
that the continental part of the LCB, imaged beneath
the NGR was already in place before the main volcanic
event and SDR emplacement.
-
A
pre-breakup underplating hypothesis for the
LCB is not fully excluded but direct involvement
of an “Icelandic mantle plume” is
not so obvious. If the crustal dome really represents
significant pre-breakup underplating, other
dynamic processes are required. However, a magmatic
interpretation of the LCB does not explain its
low magnetic susceptibility.
-
The
LCB below the NGR is interpreted here to partly
(or fully?) represent a high P-wave
velocity crystalline basement of retrograde high-
and ultra-high-pressure rocks (granulite/eclogite
material).
-
A
non-magmatic interpretation of the LCB has major
implications for estimates of the thermal history,
mantle temperature and magmatic production along
the Vøring volcanic rifted margin because
the amount of mafic material emplaced could be 20-40%
less than has been hitherto thought.
|
Acknowledgements
This work was part of a project funded
by the TotalFinaElf Exploration Norge Research Center.
A large part of this presentation is largely based
on: Gernigon,
L., Ringenbach, J.C., Planke, S., Le Gall, B. 2004.
Deep structures and breakup along volcanic rifted margins:
Insights from integrated studies along the outer Vøring
Basin (Norway). Marine and Petroleum
Geology, 21, 3,
363-372.
We thank colleagues from the IUEM (Institut
Européen de la Mer) DIAS (Dublin
Institute for Advanced Studies), UCD (University
College Dublin), VBPR (Volcanic
Basin Petroleum Research) and TotalFinaElf
for comments and corrections. We acknowledge numerous
fruitful discussions with F. Brigaud (Total) and F.
Lucazeau (IPGP)
The results and conclusions presented
here represent the views of the authors only. |
References
-
Anderson,
D.L. & Natland, J.H. 2005. A brief history of
the plume hypothesis and its competitors: concept
and controversy. In: Foulger, G.R., Anderson, D.L.,
Natland J.H. and Presnall, D.C. (eds), Plates,
Plumes & Paradigms. Geological Society
of America, Special Paper 388, in press.
-
Anderson, D.L.
2000. The Thermal State of the Upper Mantle; No
Role for Mantle Plumes. Geophysical Research
Letters, 27, 3623-3626.
-
Berndt, C., Skogly,
O.P., Planke, S., Eldholm, O., & Mjelde, R.
2000. High velocity break-up related sills in the
Vøring Basin off Norway. Journal of Geophysical
Research, 105, 28,443-28,455.
-
Boillot, G., Froitzheim,
N. 2001. Non-Volcanic rifted margins, continental
break-up and the onset of sea-floor spreading: some
outstanding questions. In: Wilson, R.C.L, Withmarsh,
R.B., Taylor, B., Froitzheim, N. (eds.), Non-Volcanic
Rifting of Continental Margins: A comparison of
Evidence from Land and Sea. Geological Society
Special Publication, 187. The Geological
Society, London, 9-30.
-
Boutillier, R.R.
& Keen, C.E. 1999. Small-scale convection and
divergent plate boundaries. Journal of Geophysical
Research, 104, 7389-7403.
-
Christiansson,
P., Faleide, J.I., & Berge, A.M. 2000. Crustal
structure in the northern North Sea: an integrated
geophysical study. In Nøttvedt, A., Larsen,
B.T., Olaussen, S., Tørudbakken, B., Skogseid,
J., Gabrielsen, R.H., Brekke, H. & Ø.
Birkeland, (eds.), Dynamics of the Norwegian
Margin. Geological Society of London, Special
Publication 167, 15-40
-
Dewey J.F., Ryan
P.D. & Andersen T.B. 1993: Orogenic uplift and
collapse, crustal thickness, fabrics and metamorphic
changes: The role of eclogites. Geological Society
of London, Special Publication, 76,
325-343
-
Doré, A.
G, Lundin, E.R., Fischler, C. & Olsen, O. 1997.
Patterns of basement structure and reactivation
along the NE Atlantic margin. Journal of the
Geological Society of London, 154,
85-92.
-
Callot, J. P.,
Grigné, C., Geoffroy, L. & Brun, J. P.
2001. Development of volcanic passive margins: Two-dimensional
laboratory models, Tectonics, 20,
148-159.
-
Callot, J.P, Geoffroy,
L., & Brun, J.P. 2002. Development of volcanic
passive margins: three-dimensional laboratory models.
Tectonics, 21, 25-37.
-
Digranes, P., Mjelde,
R., Kodaira, S., Shimamura, H., Kanazawa, T., Shiobara,
H., & Berg, E.W. 1998. A regional shear-wave
velocity model in the central Vøring Basin,
N. Norway, using three-component Ocean Bottom Seismographs.
Tectonophysics, 293, 157-174.
-
Eldholm, O. &
Grue, K. 1994. North Atlantic volcanic margins:
dimensions and production rates. Journal of
the Geophysical Research, 99,
2955-2968.
-
Eldholm, O., Gladczenko,
T.P, Skogseid, J., & Planke, S. 2000. Atlantic
volcanic margins: a comparative study. In: Nøttvedt,
A., Larsen, B.T., Olaussen, S., Tørudbakken,
B., Skogseid, J., Gabrielsen, R.H., Brekke, H. &
Ø. Birkeland (eds.), Dynamics of the
Norwegian Margin. Geological Society of London,
Special Publication 167, 411-428
-
Foulger,
G.R., Natland, J.H. and Anderson, D.L., 2005a. Genesis
of the Iceland Melt Anomaly by Plate Tectonic Processes.
In: G.R. Foulger, D.L. Anderson, J.H. Natland and
D.C. Presnall (Editor), Plates, Plumes &
Paradigms, Geological Society of America, Special
Paper 388, in press.
-
-
Fountain, D.M.,
Boundy, T.M., Austrheim, H., & Rey, P. 1994.
Eclogite-facies shear zones-deep crustal reflectors?.
Tectonophysics, 232, 411-424.
-
Geoffroy, L. 1998.
Diapirisme et extension intraplaque: cause ou conséquence?
Compte Rendus de l’Académie des
Sciences, Paris, 326, 267-273,
1998.
-
Gernigon,
L., Ringenbach, J.C, Planke, S., Le Gall, B., &
Jonquet-Kolstø, H. 2003. Extension, Crustal
Structure and magmatism at the Outer Vøring
Basin, North Atlantic Margin, Norway. Journal
of the Geological Society of London, 160,
197-208.
-
Gernigon,
L., Ringenbach, J.C., Planke, S., Le Gall, B. 2004.
Deep structures and breakup along volcanic rifted
margins: Insights from integrated studies along
the outer Vøring Basin (Norway). Marine
and Petroleum Geology, 21,
3, 363-372.
-
Korenaga, J., W.
S., Holbrook, G. M., Kent, P. B. Kelemen, R. S.
Detrick, Larsen, H.-C., Hopper J.R. & Dahl-Jensen,
T. 2000. Crustal structure of the Southeast Greenland
margin from joint refraction and reflection seismic
tomography. Journal of. Geophysical Research,
105, 21,591-21,614.
-
Korenaga, J., 2004.
Mantle mixing and continental breakup magmatism.
Earth Planet. Sci. Lett., 218,
463-473.
-
Lundin, E.R., &
Doré A.G. 1997. A tectonic model for the
Norwegian passive margin with implications for the
NE Atlantic: Early Cretaceous to break-up. Geological
Society London Journal, 154,
545-550.
-
Lundin,
E.R., & Doré A.G. 2005. The fixity of
the Iceland "hotspot" on the Mid-Atlantic
Ridge: observational evidence, mechanisms and implications
for Atlantic volcanic margins. In: Foulger, G.R.,
Anderson, D.L., Natland J.H. and Presnall, D.C.
(eds), Plates, Plumes & Paradigms.
Geological Society of America, Special Paper 388,
in press.
-
Menzies, M.A.,
Klemperer, S., Ebinger, C., & Baker, J. 2002.
Characteristics of volcanic rifted margins. In:
Menzies, M.A., Klemperer, S., Ebinger C. & Baker,
J. (eds), Volcanic Rifted Margins. Geological
Society of America Special Paper, 362,
14.
-
Mjelde, R., Kodaira,
S., Shimamura, H., Kanazawa, T., Shiobara, H., Berg,
E.W., & Riise, O. 1997. Crustal structure of
the central part of the Vøring Basin, mid-Norway
margin from ocean bottom seismographs. Tectonophysics,
277, 235-257.
-
Mjelde, R., Diagranes,
P., Shimamura, H. Shiobara, H., Kodaira, S., Brekke,
H., Egebjerg, T., Sørenes, N., & Thornbjørnsen,
S. (1998). Crustal structure of the northern part
of the Vøring Basin, Mid. Norway margin,
from ocean bottom seismographs. Tectonophysics,
293, 175-205.
-
Mjelde, R., Kasahara,
J., Shimamura, H., Kanmimura, A., Kanazawa, T.,
Kodaira, S., Raum, T. & Shiobara, H. 2002. Lower
crustal seismic velocity-anomalies; magmatic underplating
or serpentinised peridotite? Evidence from the Vøring
Margin, NE Atlantic. Marine Geophysical Researches,
23, 169-183.
-
Mosar, J., Eide,
E., Osmundsen, P.T., Sommaruga, A., & Torsvik,
T.H. 2002. Greenland-Norway separation: a geodynamic
model for the North Atlantic. Norwegian Journal
of Geology, 82, 281-298.
-
O'Reilly, B., Hauser,
F., Jacob, A., & Shannon, P. 1996. The lithosphere
below the Rockall Trough: wide-angle seismic evidence
for extensive serpentinisation. Tectonophysics,
255, 1-23.
-
Olafsson, I., Sundvor,E.,
Eldholm, O., & Kjersti, G. 1992. Møre
Margin: Crustal Structure and Analysis of Expanded
Spread Profiles. Marine Geophysical Researches,
14, 137-163.
-
Olesen, O., Gellein,
J., Håbrekke, H., Kihle, O., Skilbrei, J.R.,
& Smethurst, M. 1997. Magnetic anomaly map Norway
and adjacent ocean areas, scale 1:3 million. Geological
Survey of Norway, Trondheim.
-
Planke, S., Skogseid,
J., & Eldholm, O. (1991). Crustal structure
off Norway, 62° to 70° north. Tectonophysics,
189, 91-107.
-
Raum, T. 2000.
Crustal structure and Evolution of the Faeroe,
Møre and Vøring margins from Wide-angle
Seismic and Gravity Data. PhD thesis, University
of Bergen.
-
Ren, S., Skogseid,
J., & Eldholm, O. 1998. Late Cretaceous-Paleocene
extension on the Vøring volcanic margin.
Marine Geophysical Research, 20,
343-369.
-
Sandwell, D. T.,
& Smith, W. H. F. 1997. Marine gravity anomaly
from Geosat and ERS1 satellite altimetry. Journal
of Geophysical Research, 102,
10039-10054.
-
Saunders, A.D.,
Fitton, J.G., Kerr, A.C., Norry, M.J., & Kent,
R.W. 1997. The North Atlantic Igneous Provinces.
In: Mahoney, J.J. & M.F. Coffin, (Eds.), Large
Igneous Provinces: Continental, Oceanic, and Planetary
Flood Volcanism. American Geophysical Union,
Geophysical Monograph, 100, 45-93
-
Sheth, H.C. 1999.
Flood basalts and large igneous provinces from deep
mantle plumes: fact, fiction, and fallacy. Tectonophysics,
311, 1-29.
-
Skogseid, J., Planke,
S., Faleide, J., Pedersen, T., Eldholm, O., &
Neverdal, F. (2000). NE Atlantic continental rifting
and volcanic margin formation. In: Nøttvedt,
A., Larsen, B.T., Olaussen, S., Tørudbakken,
B., Skogseid, J., Gabrielsen, R.H., Brekke, H. &
Birkeland, Ø. (eds.), Dynamics of the
Norwegian Margin. Geological Society of London,
Special Publication 167, 295-326.
-
Van Wijk, J.W.,
Huismans, R.S., ter Voorde, M., Cloetingh, S.A.P.L.
2001. Melt generation at Volcanic Continental Margins:
no need for a Mantle Plume?. Geophysical Research
Letters, 28, 20, 3995-3998.
-
Walker, I.M., Berry,
K.A., Bruce, J.R., Bystøl, L., & Snow,
J.H. 1997. Structural Modelling of regional depth
profiles in the Vøring Basin: implications
for the structural and stratigraphic development
of the Norwegian passive margin. Journal of
the Geological Society of London, 154,
537-544.
-
White, R.S., &
McKenzie, D. 1989. The generation of volcanic continental
margins and flood basalts. Journal of Geophysical
Research, 94, 7685-7729.
|
last
updated 20th March, 2005 |
|
|