 |
|
|
Click
here to go to news and discussion of this page |
Abstract
Major features of the geology,
geophysics and tectonic setting of the northeast-propagating
Yellowstone “hot spot” are not explained
by a simple deep-mantle plume hypothesis. Within that
framework, they must be attributed to coincidence or
explained by auxiliary hypotheses. These features include:
-
the persistence
of basaltic magmatism along the hot spot track,
-
its commencement
during a regional mid-Miocene tectonic reorganization,
-
-
the occurrence
of both zones of propagation along a first-order
tectonic boundary,
-
control of
the volcanic track by preexisting structures,
-
the lack
of evidence for an unusual temperature anomaly,
-
the lack
of significant crustal thickening, and
-
the lack
of seismic evidence for, and several contraindications
of, a vertically extensive plume-like structure
beneath Yellowstone and a trailing plume head beneath
the eastern Snake River Plain (ESRP).
The high helium-isotope ratios
observed at Yellowstone and other hot spots are commonly
assumed to arise from the lower mantle, but upper-mantle
processes, which are consistent with the seismic
results, can explain the observations.
The available evidence suggests
an upper-mantle origin for the Yellowstone system, extending
no deeper than about 200 km. Processes involved in forming
the Yellowstone-ESRP system may include local, upper-mantle
convection at the discontinuity where the thin lithosphere
of the Basin & Range province meets the thick lithosphere
of the Archean craton to the north. The paired migration
of the Yellowstone and Newberry melt anomalies may be
related to the progressive migration of Basin-Range
extension to the east and west, with the Newberry melt
anomaly forming in the backarc environment of the Cascadia
subduction system (Christiansen,
2003). |
Yellowstone is the type example of a continental
hotspot [Morgan,
1971]. It is associated with a time-progressive
trail of silicic volcanism along the ESRP that
is approximately parallel to other hotspot trails
in a fixed-hotspot reference frame. It is also
associated with maximum 3He/4He
ratios up to 16 Ra, where Ra is the atmospheric
value. This characteristic is often taken to
be an unambiguous indicator of lower-mantle
affinity. Nevertheless, many factors such as
the regional history and setting of the volcanism
fit this model poorly and ad hoc adjustments
of the plume model have been invoked in order
to adapt it to explain the observations. These
adjustments include the migration of the Cascades
subduction system over the hypothesised plume,
and the temporary shutting off or deflection
of plume flow from its vertical ascent path
to produce the Columbia River basaltic volcanism,
followed by subsequent return to vertical flow
as it propagated along the ESRP.
|
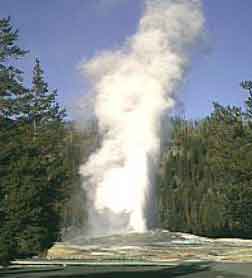
Figure 1: Old Faithful Geyser |
|
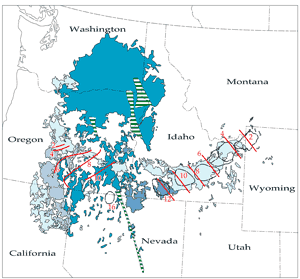
Figure 2: Yellowstone geology.
Click on image for enlargement. (from Christiansen
& Lageson, 2003) |
Geology
Geological factors that are inconsistent
with a plume hypothesis for the Yellowstone-ESRP
system include [Christiansen
et al.,
2002]:
-
The persistent basaltic
volcanism along the ESRP for hundreds of kilometres
from Yellowstone.
-
The beginning of ESRP volcanism
simultaneously with a major tectonic reorganization
to regionally distributed basin-range extension,
and fundamentally basaltic volcanism throughout
much of a region 2,000 km wide.
-
The Yellowstone-ESRP system
is paired with the Newberry
system, which propagated northwest at
the same time and rate as northeast propagation
of the ESRP-Yellowstone system (Figure 2).
|
- The Yellowstone-ESRP system marks the boundary between
a rapidly extending basin-range region to the south
and a region to the north where extension has been
much less. In the deep-mantle plume model, this location
is a coincidence.
- The Yellowstone-ESRP axis coincides with a major
regional Precambrian structural trend.
- There is no evidence from petrology for elevated
asthenosphere temperature beneath the region compared
with other volcanic areas in western North America.
Heat flow data are ambiguous, because any deep thermal
signal is obscured by groundwater circulation, but
heat flow is not higher than in neighbouring volcanically
active places in the Basin & Range province.
|
Seismology
The area has been extensively studied using several
seismological techniques:
-
There is no evidence for thicker
crust beneath Yellowstone and the ESRP compared
with the regional, which argues against a model
involving the delivery of additional melt to the
area.
-
Tomography reveals a
low-wave-speed body beneath Yellowstone
that does not extend deeper than ~ 200 km
(Figure 3) [ Christiansen
et al.,
2002]. At these depths, bodies greater
than ~ 70 km in size horizontally, with
anomalies stronger than ~ –1%, can
be resolved. Beneath the ESRP a similar
structure is detected, that is flanked by
high-wave-speed bodies up to 200 km wide
to the northwest and southeast. This is
not what is predicted if the ESRP and flanking
topographic swell represent a trailing plume
head [ Humphreys
et al.,
2000].
|
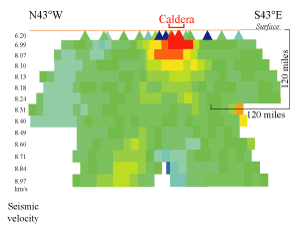
Figure 3: Cross
section through a teleseismic tomography model
of Yellowstone [from Christiansen
et al., 2002]. |
|
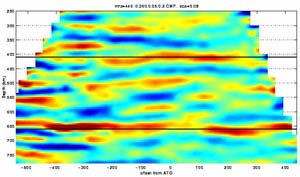
Figure 4: Vertical cross-section
showing receiver functions (mapped from the time
domain to depth) computed for seismograms recorded
on a northwest-southeast profile across the eastern
Snake River Plain in southeastern Idaho, near
the Yellowstone hot spot. Colors indicate zones
of rapid increase (red) and decrease (blue) of
wave speed with depth. Black horizontal lines:
nominal depths of the 410- and 660-km discontinuities.
Figure from the
website of Ken Dueker, University of Wyoming. |
-
A thermal anomaly in the
410–660 km depth range would be expected
to be accompanied by depression of the 410-km
discontinuity and elevation of the 660-km
discontinuity (see Transition
zone page). However, receiver function
results show that this does not occur (Figure
4) [ Dueker & Sheehan, 1997].
|
-
Whole-mantle tomography
shows that Yellowstone lies at a profound
lithospheric structural discontinuity where
the Basin-& Range region to the southwest
abuts the North American craton to the northeast.
There is no significant low-wave-speed anomaly
either in the lower mantle or the lower part
of the upper mantle. In fact, wave-speeds
are anomalously high in the lower half of
the upper mantle.
|
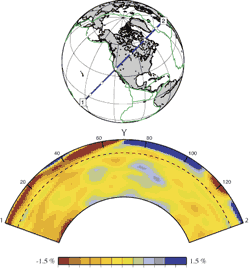
Figure 5: Whole-mantle cross
section through Yellowstone (courtesy of J.
Ritsema) |
|
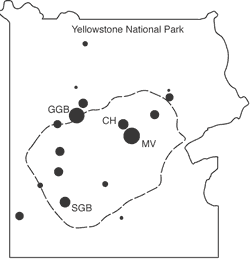
Figure 6 |
Helium
3He/4He
values at Yellowstone range from < 3 Ra outside
the caldera to 16 Ra inside the caldera. Values
higher than 8 Ra are commonly assumed to indicate
a component from the lower mantle since they are
attributed to an excess of 3He, a primordial
isotope that is thought to be largely degassed
from the convecting upper mantle. However, high
3He/4He may also result
from relatively low 4He. Since 4He
is produced by the radioactive decay of U+Th,
helium gas preserved for long periods in a low-U+Th
environment will not accumulate 4He
over time in the same way as helium in more U+Th-rich
environments [see Helium
Fundamentals page].
Figure 6: Helium-isotopic
ratios. Black dots are proportional in area to
the maximum 3He/4He observed
locally for the hydrothermal activity. The largest
dot corresponds to 3He/4He=16
Ra. Solid outline shows boundary of Yellowstone
National Park; dashed line shows Yellowstone caldera.
CH, Crater Hills; GGB, Gibbon Geyser Basin; MV,
Mud Volcano; SGB, Shoshone Geyser Basin [from
Christiansen
et al., 2002]. |
Variations in 3He/4He
in terrestrial materials thus develop over time according
to the rate of production of 4He in the host
material [Anderson,
1998a; Anderson,
1998b; Foulger
& Pearson,
2001; Meibom
et al.,
2003]. Olivine crystals represent one low U+Th host
[Natland, 2003], and may preserve ancient,
high-3He/4He in upper mantle materials.
Thus, the model that high-3He/4He
indicates the flow of helium from the lower mantle is
at best not unique, and an upper-mantle source, consistent
with the seismic observations, is possible. |
Shallow
models
If the Yellowstone-ESRP volcanic system is not due
to a thermal plume, what causes it? A number of possible
mechanisms have been suggested:
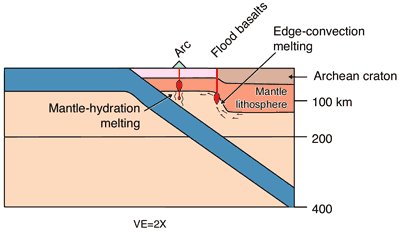
Figure 6: Model for the commencement
of volcanism, at ~ 17 Ma, that developed into
the Yellowstone-Snake River Plain system (for
more details, see Christiansen
& Lageson, 2003). |
-
The Yellowstone system
reflects interaction between local upper-mantle
convection and regional lithospheric tectonics.
This scenario involves a generic link between
eastward-migrating basin-range extension,
asthenospheric upwelling, partial melting
of lithospheric mantle, and voluminous eruption
[p. 129-130, Christiansen, 2001].
The Newberry melt anomaly has propagated west
as the subduction zone has retreated to the
west and north. These processes, are controlled
by both large-scale and local lithospheric
structure (Figure 6) (Christiansen
& Lageson, 2003).
|
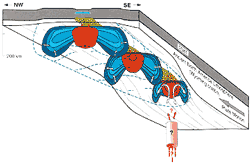
Figure 7: Convective rolls beneath Yellowstone
and the eastern Snake River Plain (from Humphries
et al., 2000)
|
-
Local, upper-mantle convection
occurs, with propagating convective rolls
organized by the sense of shear across the
asthenosphere. This can explain both the Yellowstone
and Newberry propagating volcanism with a
single upper mantle process. It incorporates
interaction of the asthenosphere with the
residuum created by melt release. The magmatic
propagation is seen as a consequence of hot,
fertile mantle subjected to shear associated
with plate transport. Such propagating, melt-driven
convective instabilities have been modeled
in computer
simulations [ Schmeling, 2000].
This model would predict the existence of
other, similar melt instabilities, and these
are seen in northwest-orientated magmatic
trends in the Great Basin such as Jemez, St.
George. The relative vigor of Yellowstone
magmatism may result from its tectonic setting
[ Humphreys
et al., 2000].
|
-
Yellowstone may represent the
tip of a propagating rift that is forming as a result
of more northerly motion of the lithosphere to the
north than to the south. There may be some evidence
for this in recent GPS surveying results. Such a
model would explain the ongoing basaltic volcanism
along the ESRP. Propagation along a pre-existing
structural discontinuity, and the triggering of
processes such as local convection, would be expected.
|
Summary
Evidence from the Yellowstone–ESRP
region strongly supports an essentially lithospheric
source for this melt anomaly. However, Yellowstone
is associated with a time-progressive volcanic trail
and high 3He/4He ratios. This
shows that such features are not diagnostic of a deep
mantle plume, and suggests that they should not be
assumed to be so at other locations.
The upper-mantle models proposed
to explain the Yellowstone system have been little
tested quantitatively as a result of the widespread
assumption of a plume as an a-priori model.
Numerical modelling of the proposed processes, and
testing against the observations at other sites of
propagating volcanism, is are important tasks for
the future.
|
News and discussion
Theories
on Park Challenged
The
Yellowstone Plume: Fact or Fiction?
Is
Yellowstone Volcanism Caused by a Deep-Seated Mantle
Plume?
|
References
-
-
-
Christiansen, R.L., The Quaternary
and Pliocene Yellowstone Plateau volcanic field
of Wyoming, Idaho, and Montana, U.S. Geological
Survey Professional Paper 729-G, 144 pp., 2001.
-
-
-
Dueker, K.G., and A.F. Sheehan,
Mantle discontinuity structure from midpoint stacks
of converted P to S waves across the yellowstone
hotspot track, J. geophys. Res., 102,
8313-8327, 1997.
-
- Hadley, D.M., G.S. Stewart & J.E. Ebel, Yellowstone
- seismic evidence for a chemical mantle plume, Science,
193, 1237-1239, 1976.
-
-
Meibom,
A., D.L. Anderson, N.H. Sleep, R. Frei, C.P. Chamberlain,
M.T. Hren, and J.L. Wooden, Are high 3He/4He
ratios in oceanic basalts an indicator of deep-mantle
plume components?, Earth planet. Sci. Lett.,
in press, 2003.
-
-
-
Schmeling,
H., Partial melting and melt segregation in a convecting
mantle, in Physics and chemistry of partially
molten rocks, edited by N. Bagdassarov, D.
Laporte, and A.B. Thompson, pp. 141-178, Kluwer
Academic Publications, Dordrecht, 2000.
|
last updated 9th
February, 2006 |
|
|