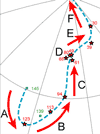 |
Insight
into Motion of the Hawaiian Hotspot from Paleomagnetism |
William
W. Sager
Department of Earth and Atmospheric Sciences, University of Houston, Houston, TX 77204-5007
wwsager@uh.edu
The
situation with hotspots – and in particular the
best known, the Hawaiian hotspot – reminds me
of an old Saturday
Night Live skit. To
quote Steve Martin: “What the hell is that!?” Like
his character, I can’t seem to decide just what
it is, nor can I turn away. For 25 years, I thought
I knew exactly what it is. Now recent research
is casting the Hawaiian hotspot in a new light. Perhaps
our earlier views on this hotspot were just too simplistic. Perhaps
they were just wrong. In a paper that is in press
in the forthcoming GSA volume “Plates,
Plumes and Planetary Processes” I compare
the motion of the Pacific plate a) reconstructed from
paleomagnetism, and b) from models of plate motion
relative to the hotspots. I
find that the two reference frames fit together nicely
for the past ~49 Ma, but before that they
are drastically different. (Sager,
in press) Those
differences may in fact be illuminating and I would
like to share a few salient points here.
For many
years it appeared that the common explanation for the
Hawaiian hotspot – and
others by implication – was just right. The
Pacific plate seemed to have about the right amount
of northward motion to be explained by a steady drift
of the plate over the nearly fixed hotspot, with a
kink in the middle where the Hawaiian and Emperor chains
join [Ed: See also The
Emperor and Hawaiian Volcanic Chains and Speculations
on tectonic origin of the Hawaii hotspot]. One
of the first indications that there might be a problem
with this explanation was from Deep Sea Drilling Project
Leg 55 and a study by Masoru Kono (Kono, 1980)
showing that lavas from Suiko Seamount, in the middle
Emperor chain, were formed at a paleolatitude of ~27° rather
than the ~20° expected
from the present location of the hotspot. Surprisingly,
this difference created little in the way of controversy
about the fixity of the Hawaiian hotspot. Ocean
Drilling Program Leg 197 took this observation to the
next level, deriving paleolatitudes for several more
Emperor seamounts and showing a progressive decrease
in paleolatitude with time and a total of ~13° displacement
(Tarduno et al., 2003). This
time, the paleolatitude discrepancy results were seen
differently because other study results were also suggesting
that the hotspot was not fixed. Efforts
to reconstruct the Hawaiian-Emperor chain geometry
from a plate circuit and plate motions relative to
the hotspots in other oceans also showed less northward
drift of the plate during the Emperor chain period
(e.g., Raymond
et al.,
2000). Furthermore,
models of mantle flow implied that hotspot conduits
ought not to stay fixed, but should instead “sway
in the mantle wind” (e.g.,
Steinberger & O’Connell, 2000; Steinberger,
2000). My
impression is that most marine geologists still accept
the hotspot explanation for the Hawaiian-Emperor chain,
but accept also that it has drifted significantly. Perhaps
this is true, but the results of my work, and comparison
with others, stretches this view still further.
In my
study, I compiled a paleomagnetic apparent polar wander
path (APWP) for the Pacific plate in much greater
detail than previously. I compared this APWP
with a synthetic APWP constructed from models of Pacific
plate motion relative to the hotspots (Wessel
et al.,
2006). If the hotspots are fixed and
the Earth’s spin axis stays fixed relative to
the mantle, the two reference frames should give comparable
results. For the past ~49 Ma, the two APWP
match well and are statistically indistinguishable
(Figure 1). Thus, the
hotspot model of plate motion seems to fit well
over that time period. Before
that, there are major differences, however.
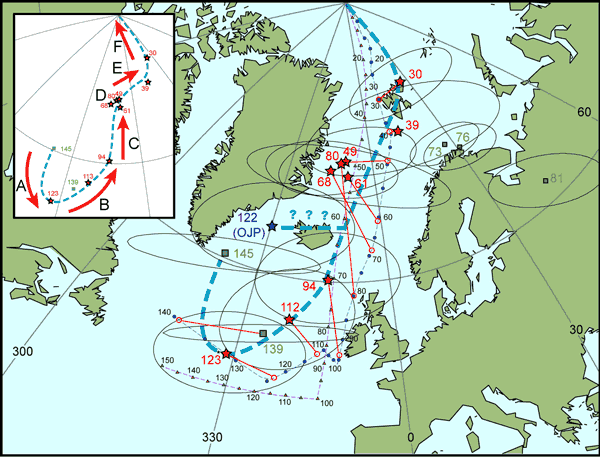
Figure 1. Pacific
apparent polar wander path. Red stars denote
pole positions defining the most likely APWP (Sager,
2006; Beaman et al., submitted), shown by the blue,
heavy dashed line. Poles are surrounded by
95% confidence ellipses and labeled by age in Ma. Blue
star denotes Ontong Java Plateau pole, which is considered
anomalous (Sager, 2006). Green squares show poles
determined from magnetic lineation skewness (73, 76,
and 81 Ma poles from Petronotis & Gordon (1999),
Vasas et al., (1994); 139 and 142 Ma poles from Larson
& Sager (1992)). The Late Cretaceous skewness
poles are considered anomalous (Beaman et al., submitted). Thin
dashed lines show the predicted polar wander path from
plate/hotspot motion models of Duncan & Clague
(1985) (purple with triangles) and Wessel et al. (2006)
(blue with dots). Triangle and dot symbols show
predicted pole positions at 5-Ma intervals, labeled
every 10 Ma. Red lines show offset between paleomagnetic
and hotspot model predicted poles. Inset sketch
map shows interpreted phases of polar wander. Plot
is an equal area map. Numbers are pole ages in
Ma. Figure from Sager
(in press). Click here or
on Figure for enlargement.
For the
period 80-49 Ma, the paleomagnetic APWP shows no motion,
i.e., a polar "standstill". This
result indicates that the Pacific plate had no discernible
northward motion. Given
the uncertainty regions for the paleomagnetic poles,
a slight amount of northward motion could have occurred, but not much more than
about 3-4°. In
contrast, the Emperor chain itself implies N-S motion
of the Pacific plate by ~19° over the same period. Although the paleomagnetic
data are far from being free of uncertainties, the magnitude of this difference
is so great that I doubt whether one can dismiss the discrepancy as a result
of poor data. There are too many different paleolatitude estimates from
different sources and the data agree. This result is very important because
it shows that the change in paleolatitude came about virtually entirely as a
result of southward motion of the melting anomaly. Most models of Pacific
plate motion in the Late Cretaceous and early Cenozoic call upon a large amount
of northward drift, but the paleomagnetic data argue otherwise (Figure 2). This
finding makes sense because it is thought that the
northern Pacific plate did not begin subducting in the Aleutian trench until
the Eocene , so the plate would not have experienced a great amount of northward
slab pull (Sager,
in press).
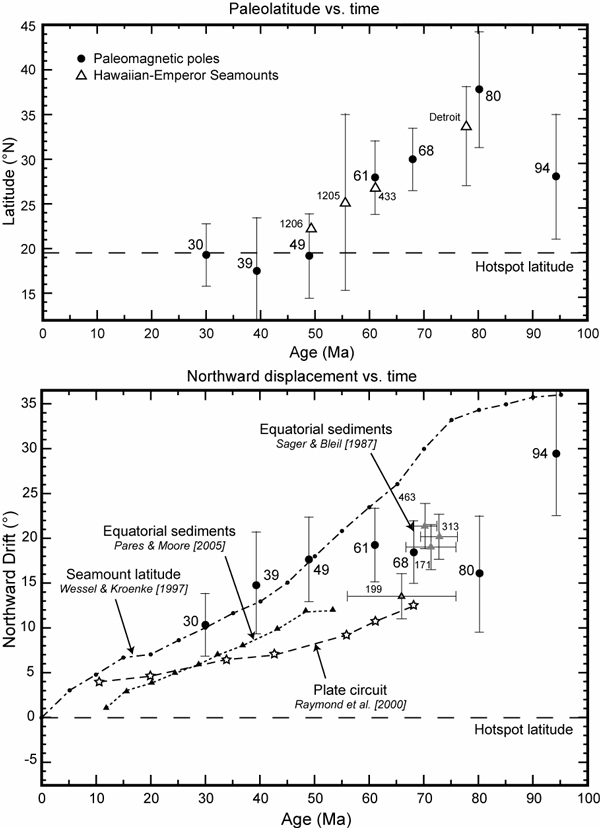
Figure 2. Paleolatitude
(top) and northward drift (bottom) implied by Pacific
paleomagnetic data vs. age. Top: Filled circles
show estimated paleolatitudes of Hawaiian-Emperor seamounts
determined by distance from paleomagnetic poles to
seamount sites (estimated from Wessel et al. (2006)
model). Open triangles show paleolatitudes from
DSDP/ODP basalt drill cores (Tarduno et al., 2003).
Bottom: Filled circles show northward drift implied
by paleomagnetic poles. Dash-dot line and dots
show northward drift of Hawaiian-Emperor Seamounts
with time (from Wessel et al., 2006). Small dashed
line and triangles denote northward drift shown by
equatorial sediments (Parés & Moore, 2005). Gray
triangles show additional estimates of northward drift
from equatorial sediments (Sager & Bleil, 1987). Heavy
dashed line and stars are estimates of seamount latitude
from the plate circuit model of Raymond et al. (2000). Numbers
are pole ages. Figure from Sager
(in press).
The formation
of the Emperor chain becomes all the more interesting
when new radiometric ages are considered. Sharp & Clague (2006)
show that the volcanic progression along the chain was not steady, as had been
thought previously (Figure 3). Instead,
the motion implied for the southern Emperor chain (17
cm/yr) is about twice the old rate (~9 cm/yr; Clague & Dalrymple,
1989). If
these dates are accurate, they raise the following questions. Would one
expect a hotspot to undergo such drastic changes in drift rate (from 5 cm/yr
to 17 cm/yr) and would one expect the drift rate to be so high (17 cm/yr)? I
am not privy to the details of mantle flow models, but my impression from articles
on the subject is that reasonable mantle parameters do not give drift rates that
are so fast (Steinberger
et al., 2004).
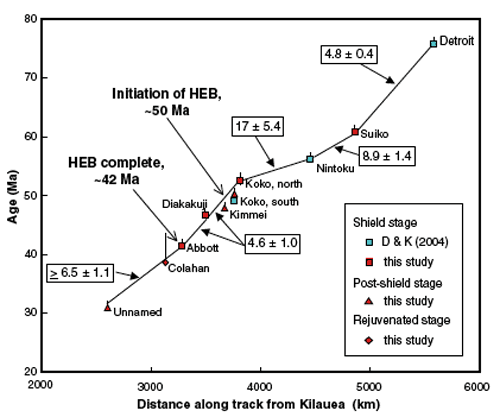
Figure 3. 40Ar/39Ar
ages of Hawaiian-Emperor volcanoes vs.
distance along the chain from the modern hotspot at
Kilauea volcano. Age errors shown include geological
uncertainties, as discussed by Sharp & Clague (2006). Boxed
values are volcanic migration rates for respective
segments of the Hawaiian-Emperor chain in cm/year.
Previously published ages (blue symbols) are from Duncan
& Keller (2004). Figure from Sharp & Clague (2006).
The paleomagnetic
results also allow a crude estimate of the motion of
the hotspot relative to a “fixed” mantle reference frame. As
shown in Figure 4, the motion of the hotspot relative to the mantle, mVh,
can be described as the vector sum of the motion of the plate relative to the
hotspot,
hVp, and the motion of the plate relative to the
mantle, mVp. In
Figure 4, I have made crude estimates of these using a planar approximation. Although
the velocity estimates are rough, the actual numbers are less important than
the implications. For
the period of the Emperor chain, the chain shows the
relative motion of the plate relative to the hotspot (hVp). The
motion of the plate relative to the mantle is ill-constrained, but the paleomagnetic
data testify that the northward motion was negligible. Assuming the spin
axis did not move significantly relative to the mantle, the motion of the Pacific
plate should have had little or no northward component. In
Figure 4, I show two possibililities:
- plate drift relative to the mantle
was equivalent to that shown by the Hawaiian chain
for the Cenozoic
(i.e., the Hawaiian chain shows the motion), or
- the
motion was entirely E-W.
Either
way, the melting anomaly must have had a significant
westward component in its motion (about the same
as the southward component). It
seems unlikely that the plate was standing still,
so the westward component of plate and hotspot motion
had to nearly cancel to produce the N-S trending
Emperor chain. This
large westward motion for the Hawaiian hotspot is
not predicted by mantle flow models (e.g., Steinberger & Antretter,
2006).
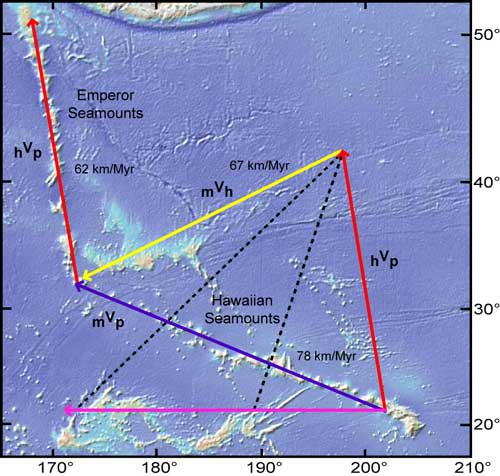
Figure 4. Sketch
of motion vectors indicating Hawaiian hotspot drift
during the formation of the Emperor Seamounts. Motion
of plate relative to hotspot, hVp (red
vector), given by trend of Emperor Seamounts. Motion
of plate relative to mantle (assumed fixed relative
to spin axis), mVp (purple vector),
is assumed to be same as at present (Hawaiian Chain). Sum
is motion of hotspot relative to the mantle, mVh (yellow
vector), which has a large westward component. Horizontal
vector at bottom (magenta) shows Pacific plate motion
if the plate had no northward component of velocity. Dashed
line vectors show predicted motion of hotspot relative
to mantle if Pacific plate motion had no northward
component. Different dashed lines correspond
to different westward velocities. Background
is a shaded relief plot of Hawaiian-Emperor Chain bathymetry. Figure
from Sager
(in press).
What
of true polar wander (TPW), the motion of the spin
axis relative to the mantle? Some
authors, myself included, have called upon this mechanism
to explain the southward motion of the Hawaiian hotspot
(e.g., Gordon & Cape, 1981; Sager
& Bliel, 1987). While
there is some support for this explanation in global
TPW curves (see Sager,
in press), I think it is unsatisfactory
because under TPW, the plate and hotspot would move
together. How then can we explain
the Hawaiian-Emperor bend? The simplest explanation
would be that the southward motion of the melting anomaly
relative to the plate stopped. If that southward
motion includes TPW, then both the TPW and motion and
the hotspot motion have to stop simultaneously.
Although
I am not yet brave enough to jump entirely off the
hotspot bandwagon (it has been a good ride for 25 years and besides, if there
was ever a hotspot, you would think that it would be
Hawaii), I have
to admit that the behavior of the hotspot seems unhotspotlike. It apparently zoomed
southwest at high speeds, sometimes going fast and sometimes slower, and screeched
to a halt at the time of the Hawaiian-Emperor bend. I suspect something
is amiss. Either
we are being misled by some of the tectonic or age
data, or we do not understand how the Hawaiian-Emperor chain formed. Back
to Saturday Night Live: What the hell is that? I don’t know what
the hell that thing is!
References
-
Clague, D. A., and Dalrymple, G. B.,
1989, Tectonics, geochronology, and origin of the Hawaiian-Emperor
volcanic chain, in Winterer, E. L., et al.,
eds., The Geology of North
America, The eastern Pacific and Hawaii, Decade
of North American Geology, vol.
N, Geol. Soc. Amer., Boulder, CO, p. 188-217.
-
Gordon, R. G., and Cape, C., 1981, Cenozoic latitudinal
shift of the Hawaiian hotspot and its implications
for true polar wander: Earth
and Planetary Science Letters, v. 55, p. 37-47.
-
Kono, M., 1980, Paleomagnetism of DSDP Leg 55 basalts
and implications for the tectonics of the Pacific
plate, in Initial
Reports of the Deep Sea Drilling Project, v. 55:
Washington, D.C., U.S. Government Printing Office,
p. 737-752.
-
Raymond, C. A., Stock, J. M.,
and Cande, S. C.,
2000, Fast Paleogene motion of the Pacific hotspots, in Richards,
M. A., et al., eds., The history
and dynamics of global plate motions: Washington, D. C., American
Geophysical Union Geophysical Mongraph 121, p. 359-375.
-
Sager,
W. W., in press, Divergence between paleomagnetic
and hotspot model predicted polar wander for the
Pacific plate with implications for hotspot fixity, in Foulger,
G. R., Jurdy, D. M., eds., Plates,
plumes, and planetary processes, Special paper 430, Geol. Soc.
Am., Boulder, CO.
-
Sager, W. W., and Bleil, U., 1987, Latitudinal
shift of Pacific hotspots during the Late Cretaceous
and early Tertiary: Nature, v. 326, 488-490.
-
Sharp, W. D., and Clague, D. A., 2006, 50-Ma initiation
of Hawaiian-Emperor bend records major change in
Pacific plate motion: Science, v. 313, p. 1281-1284.
-
Steinberger, B., 2000, Plumes in a convecting mantle:
Models and observations for individual hotspots:
Journal of Geophysical Research, v. 105, p. 11,127-11,152.
-
Steinberger, B., and Antretter, M., 2006, Conduit
diameter and buoyant rising speed of mantle plumes:
Implications for the motion of hot spots and shape
of mantle plumes: Geochemistry,
Geophysics, and Geosystems,
v. 7, p. 1-25, doi: 10.1029/2006GC001409.
-
Steinberger, B., and O’Connell,
R. J., 2000, Effects of mantle flow on hotspot
motion, in Richards,
M. A., Gordon, R. G., and van der Hilst, R. D., eds.,
The history and dynamics of
global plate motions:
Washington, DC, American Geophysical Union, Geophysical
Monograph, v. 121, p. 377-398.
-
Steinberger, B., Sutherland,
R., and O’Connell,
R. J., 2004, Prediction of Emperor-Hawaii seamount
locations from a revised model of global plate
motion and mantle flow: Nature, v. 430, p. 167-173.
-
Tarduno, J. A., Duncan, R. A.,
Scholl, D. W., Cottrell, R. D., Steinberger, B.,
Thordarson, T., Kerr, B. C., Neal, C. R., Frey,
F. A., Torii, M., and Carvallo, C., 2003, The Emperor
Seamounts: Southward motion of the Hawaiian hotspot
plume in the Earth’s
mantle: Science, v. 301, p. 1064-1069, doi: 10.1126/science.1086442.
-
Wessel, P., Harada, Y., and Kroenke, L. W., 2006,
Toward a self-consistent, high-resolution absolute
plate motion model for the Pacific: Geochemistry,
Geophysics, Geosystems, v. 7, Q03L12, doi: 10.1029/2005GC00100.
last updated 4th
June, 2007 |