Lithosphere
Architecture as a Controlling Factor of the Rifting
Process
It is known that continental
rifting tends to follow weak zones in the lithosphere,
such as orogenic belts, while stronger cratonic regions
are usually not significantly deformed. For example,
in the northern North Atlantic, rifting occurred along
the Caledonian belt, and in eastern Africa, the East
African Rift Zone is located within a Late Proterozoic
belt. My colleagues and I suggest that these old structures
not only influence the location of rifting, but that
the inherited lithosphere architecture plays a role
in the rifting process itself, the thermal evolution
of the rift, and decompressional melting during rifting.
The amount, timing and distribution of decompressional
melting for example, could be controlled by factors
such as pre-rift crustal or lithosphere thickness, orientation
of inherited structures, and lithosphere composition.
A mantle plume is not a pre-requisite to form a volcanic
province. Dynamic processes related to lithosphere extension
can explain the sometimes enigmatic amounts of melt
observed in intra-continental rifts and volcanic passive
margins.
However, when studying
continental rifts, it is difficult (or impossible) to
establish the pre-rift situation. It is not the aim
of our studies to “reconstruct” the pre-rift
situation. Instead, we investigate how small variations
in lithosphere structure that may be present at the
onset of rifting influence the system behavior.
To study the role
played by lithosphere architecture in the rifting process,
we use two-dimensional and three-dimensional numerical
models. The models are designed to study lithosphere
deformation, and detailed descriptions can be found
in Van Wijk
& Cloetingh
(2002) (2-D model) and Van
Wijk & Blackman
(2005) (3-D model). The models differ from previous
models that were used to study decompressional partial
melting during rifting (e.g., Pedersen & Ro,
1992) and as a consequence, the results predicted differ.
One of the most interesting results that are different
is that elevated mantle temperatures, e.g., as
are required by mantle plumes, are not needed to explain
volcanic margin formation in our models.
2-D Modeling
Studies: Focusing on Passive Rifted Margins
In these studies the
model domain represents a vertical section through the
lithosphere.
When the lithosphere
is extended, the crust starts to thin and warm mantle
material wells up (Figure 1). In the head of the upwelling
mantle material decompressional melting occurs. Most
melt is generated just before continental breakup, in
a ~175 km wide zone, typically between about 20 and
50 km deep.
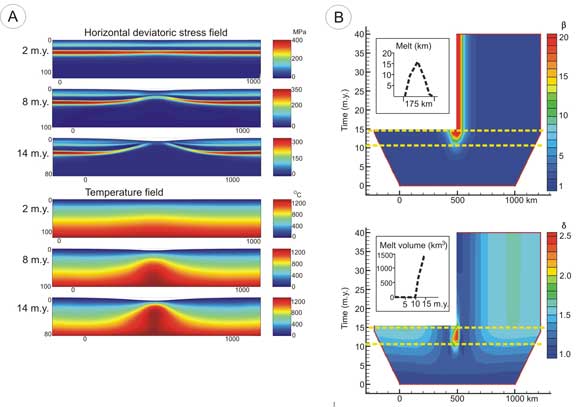
Figure 1. A) This
figure (Van
Wijk et al., 2001) shows the evolution of tensile
stresses (upper panels) and temperature (lower panels)
in the lithosphere during extension. Times are in My
after stretching started. B) This figure (Van
Wijk et al., 2001) shows the evolution of crustal
and mantle thinning during passive margin formation,
the timing of melt generation (indicated by the yellow
dashed lines), and amount of melt predicted. Models
predict that volcanic margins can form without elevated
mantle temperatures.
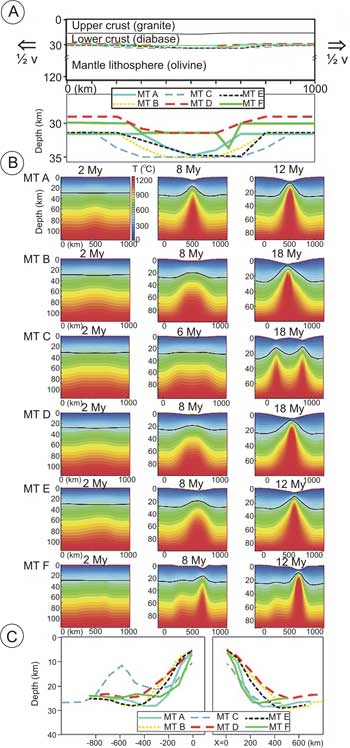 |
One of the factors
tested is the thickness of the crust and the
topography of the Moho at the onset of rifting.
We find (Figure 2) that for a fixed extension
rate, the pre-rift lithosphere configuration
influences rift duration until continental breakup
occurs, melt production, and width and symmetry
or asymmetry of the continental margin pair.
Numerical experiments show that pre-rift lithosphere
structure alone can determine whether a margin
pair will be volcanic or non-volcanic (Table
1).
Figure 2. This figure (Corti
et al., 2003) shows how the thermal evolution
of a rift basin may vary, depending on Moho
topography and thickness of the crust at the
onset of rifting. A) Initial Moho configurations
for tests A-F. The Moho tested varied in width
of depression, depth, and steepness of Moho
topography. B) Thermal evolution of lithosphere
during extension. The black line in each panel
shows the Moho. C) Final stage: Moho depths
of conjugate rifted margins predicted by the
model.
Table 1. This
table shows which of the rifted margins resulting
from the tests shown in Figure 2 are volcanic
and which are non-volcanic. The only parameter
varying in the experiments is the thickness
of the crust at the onset of extension.
Experiment |
Volcanic
or non-volcanic margin? |
A |
Volcanic |
B |
Volcanic |
C |
Non-Volcanic |
D |
Volcanic |
E |
Non-volcanic |
F |
Volcanic |
|
3-D
Modeling Studies: Focusing on Intra-continental Igneous
Provinces
Recently we have begun
to study continental lithosphere extension with a 3-D
numerical model (Van
Wijk & Blackman, 2005). Initial modeling efforts show the importance of lithosphere architecture
in the rifting process. The location and rift-axis orientation
of individual continental rift basins for example (Figure
3, and Van
Wijk, 2005) are controlled by inherited lithosphere structure. We find also that
the thermal evolution and structure of the basin (alternating
asymmetric for example), how rift zones and loci of
melt production propagate (Van
Wijk & Blackman,
2005), and mantle flow beneath rifts (Van
Wijk & Blackman,
2005) are influenced by lithosphere architecture.
Next we will apply such models to study intra-continental
hotspots (such as the Yellowstone hotspot) and volcanic
provinces.
A focus of these future
studies will be whether regional extensional tectonics
might explain the formation of, for example, the Yellowstone
hotspot. Continental extension is a 3-D process, and
we have only just begun to study lithosphere extension
from a 3-D point of view.
Figure
3. This figure (Van
Wijk, 2005) shows the location and orientation
of individual rift basins that form where a weak
structure in the lithosphere is oriented oblique
with respect to far-field plate forces (panels
labeled M2 and M3). The figure shows crustal thinning
factors, and boundaries of the weak structure
are super-positioned (white dashed lines). Crustal
thinnings and upwellings of warm mantle material
are localized in regions that follow the inherited
lithosphere structure as a whole, but cross it
individually. |
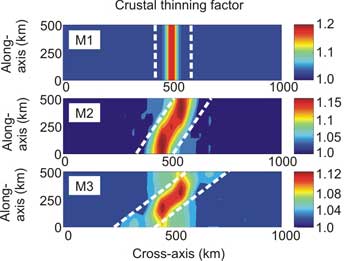 |
Formation
of the Vøring Margin (a Volcanic Passive Margin)
We suggest that volcanic rifted margins,
such as for example the mid-Norwegian Vøring
margin, are a consequence of plate tectonics, formed
without the influence of deep mantle plumes, and that
characteristic features of these margins such as underplated
or lower crustal bodies and pre-breakup reduced subsidence
(or uplift), can be explained in an alternative way,
i.e., not in terms of mantle-plume influences. The vicinity
of the Iceland hotspot (Figure 4) has led to many discussions
on its role in the formation of the Vøring margin,
but a closer look at seismic data (Gernigon et al.,
2003;
2004), and recent modeling results (Van
Wijk et al.,
2004), provide an alternative explanation for the
typical volcanic margin features (see also pages on
Iceland and the
Norwegian volcanic margin).
Gernigon et al.
(2003;
2004; and VM_Norway.html)
suggest that the continental part of the lower crustal
body is not of magmatic origin (as has often been stated
previously) and was not formed during or just prior
to the breakup event as a consequence of the influence
of the Iceland hotspot. The melt volumes observed near
the continent-ocean boundary can be explained by plate
tectonic processes alone, and do not require elevated
mantle temperatures (Van
Wijk et al.,
2004). Uplift of the Marginal High was previously
explained by arrival of the Iceland hotspot. However,
modeling results (Van
Wijk et al.,
2004) show that uplift of the Marginal High can
be explained by dynamic processes in the lithosphere/upper
mantle, as a direct result of fossil imprints in the
lithosphere from prior geological events.
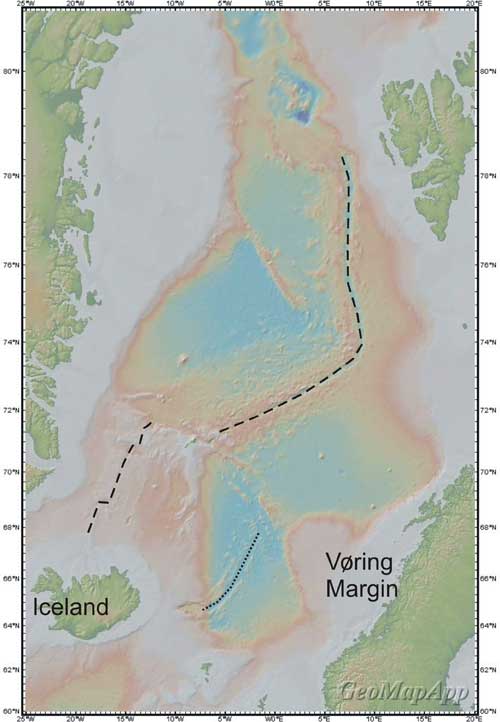
Figure 4. Locations
of the mid-Norwegian volcanic margin and the Iceland
hotspot. Many characteristic features of the Vøring
margin have been previously explained in terms of hotspot
influences.
Summary
Initial 2-D and 3-D
model experiments show that continental rifting and
decompressional melting are strongly influenced by inherited
architecture of the lithosphere. For example, pre-rift
structure alone can determine whether passive rifted
margins will be volcanic or non-volcanic, where rift
basins localize, what their orientation is, whether
and how they propagate (and how melt loci propagate),
the direction of mantle flow below the rift basin and
the amount and distribution of decompressional melting
etc. Next we aim to use these insights to study intra-continental
hotspot tracks and magmatic provinces to test whether
those tectonic features can also be explained in terms
of plate tectonics.
References
-
-
Gernigon,
L., J.C. Ringenbach, S. Planke et al., Extension,
crustal structure and magmatism at the outer
Vøring
Basin, North Atlantic margin, Norway. Journal
of the Geological Society London, 160, 197-208, 2003.
-
Gernigon,
L., J.C. Ringenbach, S. Planke and B. Le Gall, Deep
structures and breakup along volcanic rifted margins:
insights from integrated studies along the outer
Vøring Basin (Norway). Marine and Petroleum
Geology,
21, 363-372, 2004.
-
Pedersen, T.
and H.E. Ro, Finite duration extension and decompression
melting. Earth and Planetary Science Letters,
113, 15-22, 1992.
-
-
-
-
-
|